Computed Tomography in Hemorrhagic and Ischemic Stroke
Pearls
Because hyperacute or subacute hemorrhage can be of similar attenuation to that of brain, recognition can be difficult. Clues to the presence of isodense hemorrhage include subtle mass effect; displacement of sulci from the calvaria (in the case of subdural or epidural hemorrhage); obscuration of the basal cisterns or sulci (in subarachnoid hemorrhage); or subtle alterations in ventricular contour (intraventricular hemorrhage or periventricular parenchymal hemorrhage).
Acute hemorrhage in anemic patients (hematocrit <30%) may be of lower density, and possibly isodense in the acute phase, making it more difficult to recognize.
The presence of a hematocrit “layering” effect, with graded increase in density from antidependent to dependent, is suggestive of coagulopathy or anticoagulation, though it is not specific.1
The appearance of hyperdensity within a previously isodense or low-density hemorrhage is evidence of acute-on-chronic (i.e., repeat) hemorrhage.
Subacute hemorrhage may enhance peripherally, mimicking neoplasm.
Potential Pitfall
A congenitally hypoplastic or absent transverse venous sinus can mimic thrombosis. The caliber of the ipsilateral jugular vein can provide a clue: if it is small, the sinus is likely hypoplastic or aplastic; if normal in caliber, thrombosis is likely. Partial recanalization of a thrombosed vein or sinus can occur in subacute or chronic thrombosis, resulting in irregularity and decreased caliber.
♦ Role of Computed Tomography Imaging in Stroke
Computed tomography (CT) imaging has been, for nearly 35 years, the most often used imaging modality for the initial assessment of stroke patients. Modern CT scanning equipment and techniques permit a detailed study of the brain tissue and blood vessels and can even provide physiologic information about perfusion and vascular permeability. Each of these techniques can provide information helpful for the care of patients with ischemic and hemorrhagic stroke.
Noncontrast CT (NCT) remains the first-line imaging modality of choice at most institutions for evaluation of patients with acute stroke. The reasons for this include the wide availability, sensitivity to hemorrhage, and lower cost of CT compared with magnetic resonance imaging (MRI). Although MRI provides valuable information, it is often performed as a second imaging test after CT. The first diagnostic assessment is whether or not intracranial hemorrhage is present, as intracranial hemorrhage changes both the diagnosis and the management. This assessment is typically determined from the nonenhanced CT scan. If hemorrhage is present, a CT angiogram is often helpful to determine if the cause of hemorrhage is a vascular lesion (e.g., arteriovenous malformation [AVM], aneurysm). Finally, brain hemodynamic measurements with CT (or CT perfusion [CTP]) can provide increased physiologic information in patients with stroke. Such measurements include blood flow, blood volume, transit time, and even blood-brain barrier permeability.
♦ Acquisition and Display Techniques
Nonenhanced Computed Tomography
A typical adult noncontrast head CT protocol consists of 5-mm axial images every 5 mm from skull to vertex, parallel to the orbitomeatal line at 120 kVp and 120 mA for a 2-second scan. A typical display field of view (DFOV) of 25 cm with a matrix size of 512 × 512 results in a pixel size of 0.5 mm (FOV/matrix size).
Computed Tomography Angiography
In CT angiography (CTA) of the head, thin axial images acquired in the arterial phase of the infused contrast bolus are reconstructed in two-dimensional (2D) and three-dimensional (3D) fashion for diagnosis of arterial stenosis or occlusion in the setting of stroke. Optimal arterial enhancement is essential, but variable due to both technical and patient-related hemodynamic factors such as decreased cardiac output or severe arterial stenosis. In a patient with normal cardiac output, optimal arterial enhancement can be achieved with an iodine injection rate of 2.2 g/s, which translates to 6 mL/s for 370 mg/mL contrast, or a total volume of 120 mL during a 20-second scan.3 Because of this short acquisition time and wide variability in hemodynamics between patients, a bolus-triggering technique for timing the scan following contrast administration is preferable to a fixed delay, which in some patients can result in missing the arterial phase of the bolus altogether.3 For the bolus triggering technique, the scan is scheduled to begin a certain number of seconds after the attenuation in the chosen reference vessel (i.e., the aorta) reaches a certain value. Typical acquisition parameters are slice thickness of 0.625 mm acquired at a 0.625-mm interval with a helical CT pitch ratio of 0.56 to 1, 5.625 mm table speed, 120 kVp and auto mA (80–220) with 0.7-second scans. Images should be transferred to a 3D workstation and multiplanar 2D and 3D reconstructions evaluated.
A CTA of the neck may be appropriate in the setting of suspected arterial dissection, carotid or vertebral occlusive disease of the neck, or trauma. Scanning parameters and reconstruction/viewing guidelines are similar to the above.
Computed Tomography Venography
Computed tomography venography (CTV) involves the acquisition of thin axial images of the head in venous phase, which may then be transferred to a workstation for 3D and multiplanar reconstruction. A typical protocol would be acquisition of 0.625-mm images from vertex to skull base with a 0.625-mm interval, pitch of 0.56 to 1, table speed of 5.625 per revolution, 0.7 second scans with 120 kVp, and auto mA with noise index 8.0 (minimum 80, maximum 220) with an FOV of 22. As with CTA, scan timing may be with a simple delay (typically 30 to 40 seconds) following power injection of approximately 100 mL of 350 to 370 mg/mL iodinated contrast at 3 to 4 mL/s. Alternatively, the scan may be performed with a bolus-triggering technique to allow for variability in patient hemodynamics. Images should be processed and viewed as 3D and multiplanar 2D reconstructions. Although rendering of maximum intensity projection (MIP) images of the venous sinuses in isolation may be performed, this requires subtraction of osseous structures from the images (usually manually) and can be very time consuming without adding diagnostic information. For this reason, we usually favor multiplanar assessment of the venous system.
Computed Tomography Perfusion
Computed tomography perfusion is used in stroke imaging to try to define the ischemic penumbra, to follow perfusion after therapy for embolic stroke, and to predict ischemia due to vasospasm following subarachnoid hemorrhage. Dynamic first-pass CTP estimates capillary-level tissue perfusion via serial imaging of a slab of brain following the administration of intravenous contrast, taking advantage of the relationship between contrast concentration and attenuation (in Houns-field units [HU]) to measure regional cerebral blood flow (rCBF), regional cerebral blood volume (rCBV), and mean transit time (MTT). CTP is performed at 80 kVp to reduce the dose and to exploit the k-edge of iodinated contrast.4 Following administration of 35 to 50 mL of contrast (350–370 mg/mL) at 4 to 6 mL/s followed by a 20- to 40-mL saline “chaser,” a 20- to 40-mm slab of supraorbital brain (proximal middle cerebral artery [MCA]/anterior cerebral artery [ACA] territory) is scanned in two phases: (1) one image per second for approximately 45 seconds, and then (2) one image every 2 to 3 seconds for approximately 45 seconds, for a total imaging time of 90 seconds (100 mAs, FOV = 24 cm).5 Permeability measurements can also be performed but require a third phase in which an image is acquired every 10 to 15 seconds for 2 minutes.4 An additional slab may also be chosen; CTP can be performed before or after CTA. Perfusion parameters are calculated by dedicated software according to user-defined regions of interest (ROIs).
♦ Ischemic Stroke
Acute Stroke: Nonenhanced Computed Tomography
The expected findings on noncontrast CT will depend on the duration of time from onset of the ictus to the time of imaging, as well as the severity and location of ischemia. Within the first 6 hours, CT of the brain may appear normal.6 The use of newer generation CT scanners and stroke-specific window/level review settings has increased the sensitivity for early ischemic changes in the <6-hour time period.7 After 6 to 12 hours, most patients with ongoing cortical ischemia develop fairly clear-cut findings on CT. Presently, the primary role of CT imaging is to exclude hemorrhage, and a secondary role is to confirm the diagnosis of ischemic stroke. In general, the imaging findings related to acute ischemia can be classified as (1) intravascular thrombus and (2) brain edema. Intravascular thrombus is hyperattenuating on CT, whereas the CT findings associated with edema (in order of most subtle to most obvious) are sulcal effacement, loss of the normal gray matter-white matter attenuation difference, and parenchymal hypodensity.
Hyperdense Vessels
The presence of acute thrombus within a segment of the intracranial vasculature may result in increased density of that vessel on CT compared with other cerebral vessels of similar caliber. This sign is most commonly encountered in the M1 segment of the MCA (“hyperdense MCA” sign; Fig. 7.1A ), but can be observed in any vessel, including the internal carotid arteries, anterior cerebral arteries, posterior cerebral arteries, and basilar artery. Typically, normal vessels measure 30 to 40 HU, whereas arteries containing acute thrombus may measure higher, on the order of approximately 80 HU. The reported specificity of the sign is high, but the sensitivity is low, and the absence of the finding should not be used to exclude intravascular thrombus.8 When present, recognizing the presence of the hyperdense artery is important, as it portends a worse clinical outcome. Confirmation of vessel occlusion and evaluation of the extent of the thrombus can be obtained with CT angiography ( Fig. 7.1B ) or MR angiography in equivocal cases, especially if catheter directed intraarterial therapy is considered. The hyperdense appearance of the artery may resolve following spontaneous vessel recanalization or treatment with thrombolytics.

Ischemic Brain Edema
Loss of normal gray-white attenuation difference is an important early ischemic change on CT. In nonischemic brain, the gray matter of the cortex and basal ganglia is normally of greater attenuation than the white matter, causing it to appear brighter on CT images. Because gray matter is preferentially subject to edema in early ischemia, ischemic gray matter often shows a decrease in density (and thus decreased brightness). As a result, the visual contrast between the gray and white matter becomes less pronounced, resulting in obscuration of the normal gray-white differentiation. There are two well-described signs that reflect loss of gray-white contrast in the MCA territory. First, when thrombus in the M1 segment of the MCA occludes blood flow in the lateral lenticulostriate branches, developing edema leads to obscuration of the lentiform nucleus, called the “loss of basal ganglia” sign ( Fig. 7.2 ). Second, if blood flow to the insular branches of the MCA is reduced, there is obscuration of the normal gray-white attenuation difference of the insular cortex, leading to the so-called “loss of insular ribbon” sign. Although these are commonly described signs specific to MCA ischemia, the general principle that the loss of gray-white attenuation difference can be a sign of early ischemia is applicable to all vascular territories.
As the water content of the ischemic brain increases, hypodensity also develops in the affected parenchyma on CT. The degree of hypodensity at a given time is influenced by both the duration and degree of ischemia, which on the anatomic level are determined by both the location and extent of proximal vascular occlusion and the degree of collateral blood flow. Early hypoattenuation (within the first 6 hours of stroke) has been shown to correlate with irreversible ischemia, greater severity of symptoms, and worse clinical outcome.9 , 10

The presence of hypodensity alone is not sufficient to make the diagnosis of ischemia. A wide variety of other pathologic processes that affect the brain, such as neoplasms, infections, metabolic disorders, and trauma, can result in hypodensity. To distinguish stroke from these other etiologies, the hypodensity should conform to a known vascular territory. Knowledge of the geographic areas of the brain supplied by the major branches of the anterior and posterior circulation is therefore useful in making a successful interpretation. If CT hypodensity crosses major vascular territories, an etiology other than stroke should be considered first. Moreover, in stroke, the hypodensity frequently involves both gray and white matter; hypodensity that spares the cortex suggests vasogenic edema, such as due to tumor or infection, or venous ischemia.
Extent of Acute Computed Tomography Abnormality
Whether or not the size of the observed hypodensity can be used to influence therapeutic decision making remains controversial. Post hoc evaluation of the data from the European Cooperative Acute Stroke Study (ECASS-I) demonstrated a higher risk of fatal hemorrhage in patients with hypodensity in greater than one third of the expected MCA territory who were treated with recombinant tissue-type plasminogen activator (rtPA).10 This and other similar observations led to reservations about treating patients with intravenous thrombolytic therapy when large areas of hypodensity were present on initial CT. This finding was not replicated, however, in the data analysis from the National Institute of Neurological Disorders and Stroke (NINDS) rtPA trial, in which patients with hypodensity in greater than one third of the territory of the MCA who were treated with rtPA demonstrated clinical benefit, without significantly higher rates of symptomatic hemorrhage.11 The American Heart Association/American Stroke Association consensus guidelines from 2007 conclude that “data are insufficient to state that, with the exception of hemorrhage, any specific CT finding (including evidence of ischemia affecting more than one third of a cerebral hemisphere) should preclude treatment with rtPA within 3 hours of onset of stroke (class IIb, level of evidence A).”12 Nevertheless, this remains a controversial topic and the subject of ongoing investigation.
The Alberta Stroke Program Early CT Score (ASPECTS) was developed in 2000 as a standardized method for quantifying early ischemic changes on CT.13 This system, which divides the MCA territory into 10 subregions, each of which is assigned one point if normal and zero points if abnormal on CT, was created in response to the observation that even among those experienced in stroke diagnosis and care, there was poor reproducibility in identifying and classifying the extent of early ischemic change.14 The authors reported that the ASPECTS score demonstrated good reproducibility, correlated inversely with National Institutes of Health Stroke Scale (NIHSS), and predicted functional outcome and risk of hemorrhage. Subsequent evaluations of ASPECTS have demonstrated mixed results in its ability to predict outcomes and help plan therapy.15 – 17
Potential Pitfalls: Acute Ischemic Stroke
Early Mass Effect
A potential pitfall in evaluating early mass effect may be seen in the setting of diffuse cerebral volume loss. Cerebral volume loss can be the result of normal aging, a variety of medical therapies (including steroids and chemotherapeutic agents), atherosclerotic disease, or toxins (including alcohol and illicit drugs). In these patients, the sulci are abnormally wide at baseline due to the volume loss. In the situation of ischemia, there may be a pseudonormalization of the sulcal width within the region of ischemia, thereby making the changes less apparent than in a patient without baseline global volume loss, and leading to a false-negative interpretation. For this reason, an assessment of overall brain volume and comparison of sulci with the contralateral hemisphere to evaluate for asymmetry can be performed.
Hyperdense Vessel
Not all hyperattenuating arteries contain thrombus. Increased hematocrit may result in diffuse increased density of the intracerebral vessels. Atherosclerotic disease with diffuse calcification in the vessel wall, and beam hardening artifact, particularly affecting the basilar artery, can all result in apparent high attenuation within the vessel. Knowledge of the presenting clinical symptoms, pretest probability for acute stroke, and evaluation of the contralateral vasculature for density and atherosclerotic disease can be helpful in avoiding false-positive results.
Tissue Hypodensity
A pitfall to the use of the “obscuration of basal ganglia” sign is that patients are frequently asymmetrically positioned such that the appearance of the right-sided basal nuclei on a given slice may be different from that of the left-sided basal nuclei. In practice, we have found this a difficult sign to use, especially when other signs are not present. Interpretation of such a finding should be carefully correlated with the side of symptoms.
Sometimes more than one major vascular territory can be involved in stroke at the same time. For example, MCA stroke can be accompanied by ACA stroke or even posterior cerebral artery (PCA) stroke. Also, lacunar infarcts, infarcts due to occlusion of small penetrating arteries that are usually <1.5 mm in size, often produce focal hypodensities in the deep gray matter or the white matter, and typically do not involve the cortex.
Watershed infarcts, caused by focal or global decreased perfusion pressure (e.g., hemodynamic stroke; stroke due to hypotension) often cause decreased blood flow to the boundary zones between major vascular distributions, and involve regions of brain between, rather than within, the major arterial territories. Furthermore, watershed strokes may involve a disproportionally larger amount of white matter than gray matter and, in fact, can affect solely white matter in some cases. Finally, in ischemia due to venous occlusion, cortical gray matter may or may not be involved, and the pattern of hypodensity depends on the patterns of venous drainage, and therefore is not confined to normal arterial distributions.
Venous ischemia should be suspected particularly in patients with risk factors for venous thrombosis (hypercoagulable states, dehydration, oral contraceptive use, peripartum patients), with bilateral or midline distributions of hypodensity, with primarily subcortical hypodensity, and when hypodensity crosses normal arterial boundaries. Hemorrhage is commonly associated with venous infarcts, very commonly at the gray matter-white matter junction.
Subacute and Chronic Stroke
Beyond the acute phase, the appearance of ischemic stroke on noncontrast CT continues to evolve. Wedge-shaped, cortically based hypodensity becomes more pronounced over the first week, with progressively sharper definition of the affected area. Mass effect increases initially, usually peaking around 3 to 5 days, then progressively decreases. At approximately 2 to 3 weeks, the infarcts may become isodense to the adjacent brain, the so-called fogging effect. This is hypothesized to be due to the influx of macrophages and the proliferation of astrocytes, endothelial cells, and new blood vessels.18 During this period, the infarct may be inapparent, and could be overlooked if prior imaging is not available for comparison. Contrast enhancement can be seen in the subacute phase as the result of breakdown of the blood-brain barrier, which usually peaks at 2 to 3 weeks, although uncommonly can be seen earlier.19 Patterns of enhancement may be patchy, cortical, ring enhancement, or homogeneous.20
After approximately 1 month, the affected brain once again becomes hypodense as the parenchyma is replaced by gliotic tissue. In the chronic stage, encephalomalacia results in volume loss of the affected brain, with corresponding atrophy of gyri, widening of sulci, and ex vacuo dilation of the adjacent ventricular system. Injury to neurons may result in degeneration of the axon distal to the site of injury, known as wallerian degeneration. This can result in volume loss and hypodensity along white matter tracts remote from the original ischemic insult. A frequently observed manifestation of this phenomenon is atrophy of the cerebral peduncle ipsilateral to a chronic MCA infarct, due to injury of the corticospinal tracts.
Use of Computed Tomography Angiography in Stroke Imaging
Computed tomography angiography, as an adjunct to nonenhanced CT, has become a very helpful clinical tool for assessment of patients with acute ischemic stroke. CTA can help to locate or exclude arterial stenoses and occlusions, and often can provide the specific cause of stroke (e.g., arterial dissection or carotid atherosclerosis). In the special case of ischemia due to post-subarachnoid hemorrhage (SAH) vasospasm, the extent and severity of vasospasm can be documented. Occasionally, CTA can help to make an unusual diagnosis such as cerebral vasculitis.
Computed Tomography Perfusion in Ischemic Stroke
Ischemic stroke is likely the most important potential clinical application for cerebral blood flow (CBF) imaging. CTP is a highly sensitive method for detecting hemodynamic alteration. Thus, detection of perfusion abnormality, especially if it corresponds well to an expected arterial territory, can be helpful for confirming ischemia in a patient with acute symptoms.
Computed tomography perfusion imaging also provides access to knowledge of the pathophysiologic pattern of a lesion. For example, nearly all ischemic lesions are characterized by prolonged MTT but often have variable measurements of CBF and cerebral blood volume (CBV). It has been proposed that different patterns may be present for viable and nonviable ischemic tissue. For example, it has been proposed that viable tissue may be characterized by increased MTT, decreased CBF, and normal-to-increased CBV, whereas nonviable tissue may be characterized by increased MTT, decreased CBF, and decreased CBV.21 Characterizing tissue by prognosis and developing treatment protocols related to tissue prognosis is an area of very active research.22 , 23 At the present time, the available evidence does not support treatment based on CT perfusion imaging.
Evaluation of the axial CTA source images has also been proposed to be helpful as a marker for hypoperfusion. Although partly dependent on technique and individual hemodynamics, underperfused regions of brain can have decreased uptake of iodinated contrast material and so the apparent attenuation difference between hypoperfused regions and adjacent normal regions can be accentuated, making them easier to recognize.24 It is not clear at the time of this writing that the evidence supports the general use of CTA source images to make treatment decisions.
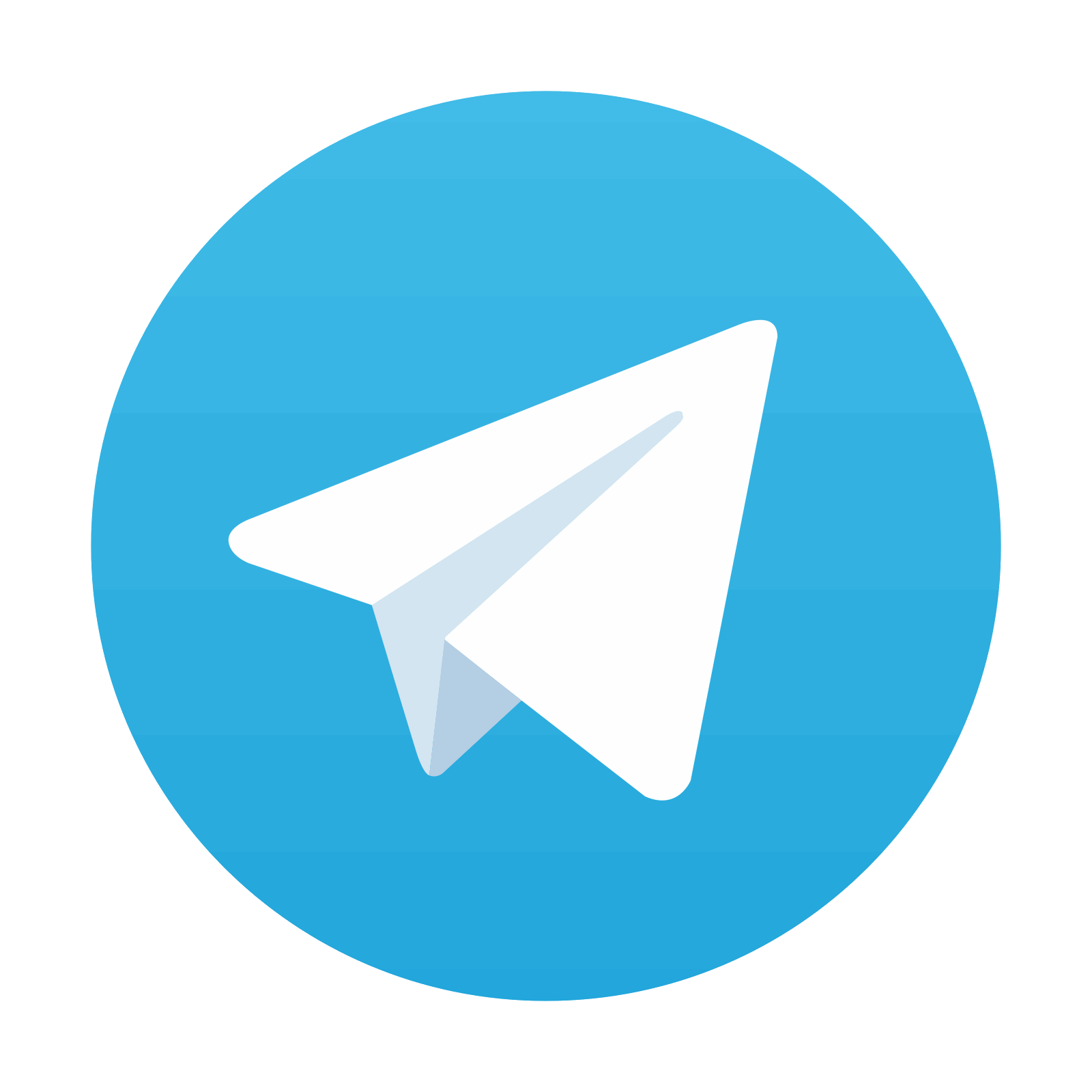
Stay updated, free articles. Join our Telegram channel

Full access? Get Clinical Tree
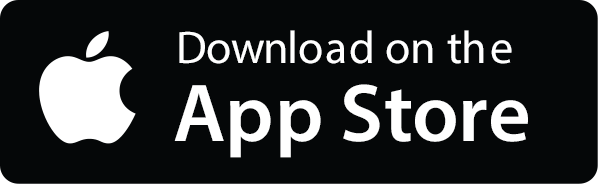
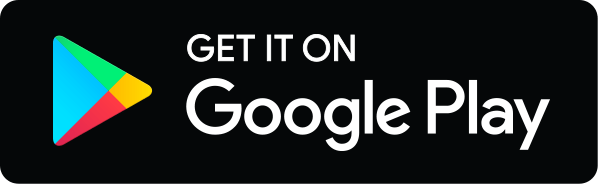
