Magnetic Resonance Imaging in Hemorrhagic and Ischemic Stroke
Pearls
Susceptibility weighted imaging differs from conventional echo planar imaging (EPI) or gradient echo (GRE) T2*-weighted imaging in that both phase and magnitude information are incorporated.
Susceptibility weighted imaging has greater sensitivity for detection of microbleeds, diffuse axonal injury hemorrhages, intratumoral hemorrhage, and cerebral cavernous malformations.
Susceptibility weighted imaging may depict slow-flow lesions such as capillary telangiectasias.
Susceptibility weighted imaging may distinguish calcium from hemorrhage.
See also Table 8.13 .
♦ Acute Ischemic Stroke
The introduction of magnetic resonance imaging (MRI) for acute ischemic stroke imaging has greatly improved our ability to identify stroke, understand the underlying pathophysiology, triage patients, and select appropriate treatments. Of the multiple parameters, diffusion weighted imaging is the most reliable for detecting acute ischemic stroke, and it can do so with high sensitivity and specificity, within 30 minutes of symptom onset. Perfusion MRI depicts hemodynamic conditions at the microvascular level, and can therefore identify regions of brain tissue that are viable, but at risk of infarction if timely reperfusion does not occur. This chapter discusses how diffusion and perfusion MRI can help identify infarct core, penumbra, benign oligemia, and the risk of hemorrhagic transformation, and how diffusion and perfusion MRI can be used to predict patient outcome and select appropriate therapy. This chapter also discusses magnetic resonance angiography (MRA) and its uses and applications.
Conventional Magnetic Resonance Imaging
Acute Stroke
Conventional MRI sequences (fluid-attenuated inversion recovery [FLAIR] and T2) are most useful for assessing extent and age of subacute and chronic infarctions ( Figs. 8.1 and 8.2 ; Table 8.1 ). Their utility rests on detection of edema- related changes after an occlusive event. In the acute setting (first 6 hours), tissue changes are primarily due to shifts of water from the extra- to intracellular space, with relatively little increase in total tissue water. Consequently, the sensitivity of FLAIR and T2-weighted images for detecting the parenchymal changes associated with acute stroke are very low, ranging from 8.3 to 26% in the first 6 hours after symptom onset.1 – 3
The FLAIR and T2-weighted images are therefore most useful as adjuncts to diffusion-weighted imaging (DWI) and vessel imaging. When a patient presents with stroke symptoms of unknown duration, with a DWI-hyperintense lesion but little or no associated FLAIR hyperintensity, it is generally accepted that the stroke is less than 6 hours old. FLAIR can also show a thrombus or slow flow, demonstrated as high signal in the affected vessel, and present in up to 65% of cases.4 , 5 On T2-weighted images, thrombus and slow flow can be detected by the absence of normal flow voids.6
Gradient echo T2* images are typically included in acute stroke protocols, as they are highly sensitive to the detection of paramagnetic blood products, and are at least as sensitive as computed tomography (CT) for the detection of hemorrhage.7 They also have relatively high sensitivity (86% sensitivity in one large study8) for the detection of acute thrombus. Gadolinium-enhanced T1-weighted sequences can also be obtained; in the acute period, arterial vessels in the affected region may enhance due to slow flow in collateral vessels.6 During this period, the parenchyma usually does not enhance. However, in rare cases, early parenchymal enhancement may occur when there is early reperfusion or good collateralization.9

Subacute and Chronic Stroke
In the early subacute period (1 to 7 days), vasogenic edema predominates, resulting in increased T2 and FLAIR hyperintensity, T1 hypointensity, and brain swelling. The brain swelling peaks at 3 to 5 days and usually resolves by 7 to 10 days. Vascular enhancement can persist for 1 week, and arterial FLAIR hyperintensity can persist for up to 2 weeks.10
During this period, parenchymal, gyral, and meningeal enhancement can also be seen. Parenchymal enhancement (usually gyral) is due to collateral circulation, or recanalization and breakdown of the blood-brain barrier. It is consistently present by 6 days after stroke, and usually persists for 6 to 8 weeks. Gyral enhancement without mass effect is unusual in other settings, and therefore is almost pathognomonic for late subacute infarction. Meningeal enhancement, thought to represent reactive hyperemia, usually occurs within 1 to 3 days and disappears within 1 week.11
By 6 weeks, the chronic stage of infarction is well established: the inflammatory response is complete, edema has resorbed, and the blood-brain barrier and reperfusion have been established. Enhancement of parenchyma, meninges, or vessels has resolved. This stage is characterized by encephalomalacia, with cystic cavitation and increased water content causing T2 hyperintensity and T1 hypointensity. Peripheral gyriform T1 shortening, due to laminar necrosis, can also occur. Large middle cerebral artery (MCA) territory infarcts usually have associated wallerian degeneration, with T2 hyperintensity and tissue loss of the ipsilateral cortical spinal tract.11 – 13
Diffusion Magnetic Resonance Imaging of Acute Stroke
Basic Physics
For detailed descriptions of the physics of diffusion weighted imaging, several reviews are available.14 , 15 Briefly, DWI is based on a spin-echo echo planar imaging sequence, with a large magnetic field gradient applied before a radiofrequency (RF) pulse, followed by application of equal and opposite gradient pulses. The first gradient de-phases protons, and the second gradient re-phases them. Within any given voxel, if there is no net movement of water protons, the two balanced gradients negate each other. However, if there is net movement of water molecules due to diffusion, the protons experience the first gradient pulse at one location and the second gradient pulse at a different location; the two gradients are no longer experienced as equal in magnitude, and therefore do not cancel each other out. Faster-moving water protons undergo a larger net dephasing. The resultant signal intensity of a voxel of tissue containing moving protons is equal to its signal intensity on a T2-weighted image, decreased by an amount related to the rate of diffusion. Therefore, tissue with the most restricted protons (e.g., in acute ischemic tissue) has the highest signal.14 , 15

It is important to note that in addition to contrast due to differences in diffusion, DWI images also have T2-weighted contrast. Signal intensity is linearly related to T2 signal, and exponentially related to diffusion. To remove the T2 contrast, a map of apparent diffusion coefficient (ADC) values is created by obtaining two image sets, one with a very low b value and one with b = 1000 second/mm2. By plotting the natural logarithm of the signal intensity versus b for these two b values, the ADC can be determined from the slope of this line. Alternatively, the DWI can be divided by the echoplanar spin echo (SE) T2 image (or low b value image), to give an “exponential image,” the signal intensity of which is exponentially related to the ADC.14
Regions with abnormally restricted diffusion are hyperintense on DWI. However, regions with normal or increased diffusion may be hypo-, iso-, or hyperintense, depending on the strength of the diffusion and T2 components (T2 shine-through effect). For this reason, review of the ADC maps or the exponential images is essential; a DWI-hyperintense lesion with truly restricted diffusion is dark on ADC maps and bright on exponential images. These images are also useful for detecting areas of increased diffusion that may be masked by T2 effects on the DWI images.14
Theory for Restricted Diffusion in Acute Stroke
The physiologic basis for the restricted diffusion associated with acute stroke is still under investigation ( Table 8.2 ). The predominant theory is that acute ischemia causes adenosine triphosphate (ATP) concentrations to fall, and Na+/K+ adenosine triphosphatase (ATPase) and other ionic pumps to fail. This leads to net translocation of water from the extracellular into the intracellular compartment where water movement is relatively more restricted. Resultant cell swelling causes a decrease in the size of the extracellular space, leading to more restricted movement of protons in that space as well. Degradation of intracellular components, leading to increased intracellular viscosity, may also play a role. Temperature decreases and cell membrane permeability have also been proposed as minor contributing factors.15
Time Course of Acute Stroke in Diffusion-Weighted Imaging
Restricted diffusion in the setting of acute stroke, manifest as high signal on diffusion-weighted images and low signal on ADC maps, has been reported in as early as 11 minutes,16 and is reliably visible 30 minutes after symptom onset15 ( Fig. 8.3 ; Table 8.3 ). Diffusion continues to decrease, reaching a nadir at 1 to 4 days. Several hours after stroke onset, the release of inflammatory mediators from ischemic brain tissue results in increasing vasogenic edema and increasing numbers of more mobile water molecules. Cell membrane breakdown and degradation result in the further removal of obstacles to water diffusion. Consequently, after 1 to 4 days, the ADC begins to rise, returning to baseline (“pseudo-normalizing”) at 1 to 2 weeks. At this point, the infarct is isointense on ADC and exponential images but remains hyperintense on DWI images due to the T2 component. As the infarct cavitates and fluid or gliosis replace the dead tissues, the ADC continues to rise; in the chronic stage, ADC maps demonstrate increased signal, whereas exponential images demonstrate decreased signal. DWI images may vary from slight hypointensity to slight hyperintensity, due to the varying contributions of diffusion and T2-weighting.
The time course is influenced by several factors including infarct type and patient age.17 For example, in lacunar infarcts, ADC reaches its nadir more slowly, and increases later than in other stroke types. In non-lacunes, younger patients demonstrate faster increases in ADC.18 Early reperfusion may also alter the time course: in acute stroke patients who receive intravenous recombinant tissue-type plasminogen activator (rTPA) within 3 hours of symptom onset, pseudonormalization can occur as early as 1 to 2 days.19 Furthermore, there can be different rates of tissue evolution within the same lesion. One study demonstrated that while the average ADC of an ischemic lesion is depressed within 10 hours, different zones within an ischemic lesion may demonstrate low, pseudo-normal, or elevated ADCs.20 In spite of these variations, in the absence of thrombolysis, tissue with reduced ADC nearly always progresses to infarction.

Accuracy of Diffusion-Weighted Imaging in Acute Stroke
Diffusion-weighted imaging is highly sensitive and specific for the detection of hyperacute and acute infarction. Reported sensitivities range from 88 to 100% and reported specificities range from 86 to 100%.3 , 21 Infarctions not identified on DWI are usually very small and located in the brainstem, deep gray nuclei, or cortex22 ( Fig. 8.4 ). False-positive acute infarctions can occur in patients with subacute or early chronic infarcts that appear bright on DWI due to T2 shine-through. This error is easily avoided by interpreting the DWI images in combination with ADC maps or exponential images. Falsepositive DWI images can also occur with restricted diffusion due to several other entities, as outlined in Table 8.4 . When reviewed in conjunction with conventional MRI images, these lesions can usually be easily distinguished from acute infarctions.15 Certain entities, including hypoglycemia ( Fig. 8.5 ), acute demyelinative lesions, and hemiplegic migraine, can present with an acute neurologic deficit and a single FLAIR hyperintense, nonenhancing lesion with restricted diffusion. Because of this, they are at times misdiagnosed as acute strokes.15
Diffusion-Weighted Imaging Reversibility
It is commonly accepted that DWI represents tissue that is destined to infarct. The ultimate volume of an infarct is usually larger than that seen on initial DWI images, encompassing both the initial DWI abnormality and other tissue into which the infarct extends. Indeed, reversibility (abnormal on initial DWI but normal on follow-up images) of DWI hyperintense lesions is very rare except in the setting of early reperfusion, usually following intravenous or intraarterial thrombolysis ( Fig. 8.6 ; Table 8.5 ).
In fact, more recent studies have suggested that partial DWI reversal following thrombolysis with early reperfusion is relatively common. For example, of the 32 of 74 patients enrolled in the Diffusion and Perfusion Imaging Evaluation for Understanding Stroke Evolution (DEFUSE) trial who had early recanalization following administration of intravenous tissue-type plasminogen activator (rTPA), 23 had partial reversal of the initial DWI lesion, with an average 47% reversal of the original DWI lesion size to the follow-up 3-month T2 lesion size.23 However, it should be noted that judging whether tissue with a diffusion abnormality is normal at follow-up can be difficult. Kidwell et al24 reported a decrease in size from the initial to the follow-up DWI abnormality immediately after intraarterial thrombolysis in eight of 18 patients, with a mean decrease in lesion volume of 52%. However, despite the initial apparent recovery, a subsequent increase in DWI lesion volume was observed in five of the eight patients. Additionally, because tissue loss is a feature of chronic infarction, diminished infarct volumes on 1-month follow-up could be due to tissue loss rather than to reversal of the initial DWI lesion.



Although an absolute ADC threshold for tissue infarction has not been defined, a number of studies have demonstrated that ADC values are significantly higher in DWI-reversible tissue compared with DWI-abnormal tissue that progresses to infarction. Mean ADCs range from 663 to 732 × 10−6 mm2/sec in DWI reversible regions compared with 608 to 650 × 10−6 mm2/sec in DWI abnormal regions that progress to infarction.24 , 25 Fiehler et al26 also showed that 49% of acute ischemic tissue with a relative ADC of 70 to 80% demonstrated DWI reversal, whereas only 6% of acute ischemic tissue with a relative ADC of less than 50% demonstrated reversal.
Perfusion Magnetic Resonance Imaging of Acute Stroke
Perfusion-weighted imaging (PWI) describes techniques that visually depict hemodynamic conditions that occur in capillaries and other microscopic blood vessels. Because impairment at the microvascular level most directly causes ischemic damage to brain tissue, PWI can guide acute stroke therapy by identifying regions of brain tissue that are underperfused but not yet infarcted, and can thus be rescued by timely initiation of appropriate treatment.
Dynamic Susceptibility Contrast: Principles
In the acute stroke setting, perfusion imaging is generally performed with a bolus tracking technique, dynamic susceptibility contrast (DSC) imaging. DSC relies on the decrease in signal due to magnetic susceptibility effects of gadolinium as it passes through the intracranial vasculature. Because blood passes through the brain parenchyma rapidly, the most commonly used sequence is a single-shot gradient echo echo planar imaging (EPI) sequence capable of multiple slice acquisitions from a single repetition time (TR). Typically, gadolinium is injected rapidly (5 to 7 cc/s) into a peripheral intravenous catheter, and then images are obtained repeatedly as the contrast agent passes through the brain. The technique takes approximately 1 to 2 minutes, and is performed so as to track the first pass of the contrast bolus through the intracranial vasculature, without recirculation effects. Approximately 60 images are obtained for each 5-mm brain slice, covering the entire brain.27
Perfusion Maps and Technical Considerations
The images obtained in the examination are converted by a computer to a contrast agent concentration-versus-time curve. The cerebral blood volume (CBV), the fraction of tissue that is occupied by blood within a given voxel, is proportional to the area under the curve. The cerebral blood flow (CBF), cubic centimeter of blood per minute per cubic centimeter of tissue, and mean transit time (MTT), the average time it takes a tracer to pass through the tissue, are typically computed with an arterial input function (AIF) and deconvolution methodology. These three cerebral perfusion parameters are related according to the central volume theorem: MTT = CBV/CBF. Other transit time measures that are commonly obtained are time to peak (TTP), the time it takes to reach maximal susceptibility effect; and Tmax, the time-to-peak of the deconvolved residue function.
Singular value decomposition (SVD) is the standard deconvolution algorithm used to create perfusion maps. However, maps created with this algorithm are susceptible to error introduced by a delay in the bolus arrival, and dispersion or dilution of the bolus.28 Selecting an ipsilateral AIF proximal to the region of interest minimizes delay but increases dispersion, whereas selecting a contralateral AIF increases delay and minimizes dispersion.29 In general, delay and dispersion cause underestimation of the CBF and overestimation of the tissue at risk. To reduce this error, automated, bolus-delay corrected, and tracer arrival time-insensitive AIF selection have been proposed as solutions.30 , 31
Other Types of Perfusion-Weighted Imaging
In arterial spin labeling (ASL), an additional MRI coil is placed over the patient’s neck and used to excite hydrogen nuclei (“spins”) as they pass through one of the major cervical arteries en route to the brain. The spins themselves serve as an endogenous contrast agent. Passage of these spins through the brain can be used to measure cerebral blood flow. This method is attractive because it is completely noninvasive and can be used safely in patients with contrast allergies. In addition, individual cervical arteries can be selectively labeled, so that the vascular territory of each cervical artery can be individually imaged. However, current ASL pulse sequences are more time-consuming than DSC and thus more susceptible to patient motion. In addition, the maps generated are far noisier, with poorer spatial resolution than DSC maps. They require further validation as a tool in acute stroke.32
Diffusion and Perfusion in Acute Stroke Treatment
Correlation of Diffusion-Weighted Imaging and Perfusion-Weighted Imaging with Clinical and Tissue Outcome
A number of studies have shown that DWI can be used to predict clinical and radiologic outcomes. Initial DWI lesion volume correlates well with final infarct volume on T2 and FLAIR images, and slightly underestimates it, with correlation coefficients ranging from 0.72 to 0.9.3 , 21 , 25 , 31 , 33 Initial DWI lesion volume also correlates well with clinical outcome, as measured by multiple neurologic assessment tests, including the National Institutes of Health Stroke Scale (NIHSS) score, Canadian Neurological Scale, Glasgow Outcome Scale, Barthel index, and modified Rankin Scale.3 , 34 Correlation coefficients between DWI lesion volume and clinical outcome range from 0.65 to 0.78. In general, correlations are stronger for cortical strokes than for penetrator artery strokes and for left-sided versus right-sided strokes.34 Recent work in early reperfusion trials shows that DWI correlates best with final infarct and with clinical outcome scales when there is complete reperfusion.35 Furthermore, large initial DWI volumes predict poor outcome. For example, one study demonstrated that for internal carotid artery (ICA) and MCA strokes treated with various therapies including thrombolytic agents, a DWI volume greater than 89 cc was highly predictive of early neurologic deterioration (receiver operating characteristics [ROC] curve with 85.7% sensitivity and 95.7% specificity).36 Another study, performed in patients with proximal MCA emboli, who received intravascular thrombolytic agents, demonstrated that patients with an initial DWI lesion volume of greater than 70 cc had a much worse outcome compared with those patients with an initial DWI lesion volume of less than 70 cc (71.5% vs. 0% 90-day mortality).37 A third study, investigating anterior circulation strokes treated with intraarterial therapy, showed that 100% of patients with an initial DWI lesion volume greater than 70 cc did poorly (defined as a modified Rankin Scale score of 3 to 6).31 Because patients with an initial large DWI stroke volume have poor outcomes and an increased risk of intracranial hemorrhage, those with an initial DWI lesion volume of greater than one third of the MCA territory or greater than 100 cc are typically excluded from acute stroke trials.33 The CBV abnormality (depicting changes due to capillary collapse) also represents infarct core; however, it is not as accurate as DWI and is only used if the DWI images are technically inadequate.
In general, perfusion parameters such as CBF, MTT, TTP, and Tmax lesion volumes are generally much larger than the DWI lesions, correlate less well with final infarct volume, and on average greatly overestimate final infarct volume23 , 38 ( Table 8.6 ). Tissue that appears normal on DWI but abnormal on the perfusion images is thought to represent the ischemic penumbra. The ischemic penumbra usually surrounds the core, where collateral vessels supply some residual perfusion. The penumbra represents tissue that may progress to infarction or may recover depending on the timing of reperfusion and the degree of collateralization.39
The number of patients who have an ischemic penumbra is estimated to be at least 80% within the first 3 hours.40 Additionally, a penumbra is much more likely to occur with proximal arterial occlusions than with lacunar or distal infarcts. In fact, a recent study showed that 80% of patients with untreated proximal artery occlusions can have mismatches that last for more than 9 hours.41 Volumes of perfusion lesions also correlate with clinical outcome. The correlations are highest when there is no reperfusion and the infarction eventually extends into the penumbra.
The best perfusion parameter for determining the penumbra has been a subject of much investigation; a recent study comparing different PWI parameters within the same cohort found large variances in penumbral estimates, within the same patient.42 There is no current consensus on which PWI lesion to measure, how to measure it, and which postprocessing software to use. Earlier studies suggested that deconvolved MTT maps best predicted follow-up tissue outcome. However, more recent studies have found no benefit of MTT over other transit time measures.43 In general, MTT, TTP or Tmax are chosen to represent the penumbra because contrast-to-noise ratios are higher on these maps compared with others.
Furthermore, the PWI defect may contain regions with benign oligemia, that is, tissue that will survive whether or not there is early reperfusion. To separate true mismatch from benign oligemia, some investigators have compared thresholded blood flow and transit time map lesion volumes with follow-up lesion volumes in patients who did not reperfuse. A Tmax of 4 to 6 seconds provided the best estimate of final infarct volume in a subset of patients from the DEFUSE trial.44 Christensen et al43 demonstrated that a relative Tmax of 1.45 seconds, a relative MTT of 1.78 seconds, and a relative first moment of 3.51 seconds were the optimal operating points on ROC curves comparing initial transit time abnormalities to follow-up infarct, on a voxel by voxel basis.
In patients who reperfuse, a threshold for tissue infarction is more difficult to define because the thresholds are dependent on the timing of reperfusion. In a seminal primate study, the CBF threshold for tissue infarction was 17 to 18 mL/100 g/min if the subject experienced permanent occlusion. However, if perfusion was reestablished in 2 to 3 hours, the subject could tolerate a much lower CBF, 10 to 12 mL/100 g/min, before infarction occurred.45 Similarly, Schaefer et al,46 comparing initial CBF with follow-up infarction on a voxel by voxel basis, demonstrated optimal relative CBF thresholds of 0.27 (i.e., relative CBF 27% of normal) for tissue reperfused at less than 6 hours, versus 0.41 for tissue that is reperfused at greater than or equal to 6 hours. Better models to predict tissue at risk are under development. For example, Wu et al47 developed a voxel-based generalized linear threshold model utilizing DWI and multiple perfusion maps that improved predictive value of tissue outcome compared with models based on a single parameter.
Mismatch and Patient Selection
After a patient presents with an acute stroke syndrome, the current standard of care is to perform noncontrast head CT, which if negative for a hemorrhage or for a >100 cc hypodensity in an MCA territory, allows for administration of IV rTPA. This is based on well-accepted clinical data dating back to the National Institute of Neurological Disorders and Stroke (NINDS) study in 1995.48 A recent large phase 3 thrombolysis trial suggested that administration of IV rTPA up to 4.5 hours after the onset of the stroke is efficacious.49 , 50 If a patient presents with acute stroke but is outside the 3- to 4.5-hour window for IV therapy, delayed IV therapy, or intraarterial recanalization, currently recommended up to 6 hours after onset of symptoms, may be attempted.49 In either case, to ensure best application of an aggressive treatment with inherent risks, proper patient selection is crucial. This is where PWI in conjunction with DWI is most useful.
There are five possible scenarios when obtaining DWI and PWI, as outlined in Table 8.7 and Figs. 8.7 , 8.8 , and 8.9 . A DWI lesion without a PWI lesion or larger than the PWI lesion implies early spontaneous reperfusion. If the DWI lesion is equal to the PWI lesion, it is generally accepted that the infarct has completed. Patients with these patterns usually do not receive thrombolytic therapy or recanalization because the infarction is not expected to extend.51 , 52 Aggressive therapies generally concentrate on two groups, a DWI lesion smaller than the PWI lesion, or a PWI lesion without a DWI lesion, because those are the patients with tissue at risk of further infarction.

Several clinical trials addressing the use of intravenous thrombolytic agents beyond the 3-hour time window have validated the mismatch concept. The Desmoteplase in Acute Ischemic Stroke (DIAS) trial51 and the Dose Escalation of Desmoteplase for Acute Ischemic Stroke (DEDAS) trial53 utilized a 20% mismatch between the PWI and DWI lesions as a trial entry criterion; patients were given intravenous desmoteplase (a thrombolytic agent derived from bat venom) or placebo at between 3 and 9 hours after symptom onset. These trials demonstrated that administration of desmoteplase was associated with dose-dependent rates of higher reperfusion and better clinical outcomes compared with placebo. In the DEFUSE study, 74 patients recruited on the basis of CT findings received IV rTPA within 3 to 6 hours after stroke onset. Early reperfusion was associated with a significantly increased chance of a favorable clinical response in patients with at least a 20% DWI-PWI mismatch, whereas patients without a mismatch did not benefit from early reperfusion.52 In the Echoplanar Imaging Thrombolytic Evaluation Trial (EPITHET), 101 patients were recruited on the basis of CT selection criteria and received IV rTPA or placebo within 3 to 6 hours after stroke onset; of these, 86% had a mismatch. Although there was no significant difference in infarct growth between patients who received placebo and those who received IV rTPA, there was increased reperfusion in those with a mismatch; additionally, reperfusion was associated with less infarct growth and improved clinical outcomes.54 When mismatch as a target for therapy was first introduced, it was arbitrarily defined as PWI lesion 20% larger than the baseline DWI lesion volume. However, some investigators have suggested using a larger mismatch. For example, the DEFUSE investigators retrospectively determined an optimum PWI/DWI ratio of 2.6 (i.e., a PWI lesion 2.6 times larger than the DWI lesion) for the best chance of a favorable outcome after early reperfusion.55 The EPITHET investigators suggest a 2.0 ratio.56 The MRA-DWI mismatch is a possible alternative model. THE DEFUSE investigators found that patients with a MRA-DWI mismatch (defined as a DWI lesion less than 25 cc with a proximal vessel occlusion, or a DWI lesion less than 15 cc with a proximal vessel stenosis or distal abnormal vessel) had an increased rate of favorable clinical response when reperfused compared with those without a mismatch.57

Most patients do not receive MRI prior to intraarterial therapy due to the relative inaccessibility of MRI and the consequent delay in treatment. However, one recent study demonstrated that in patients with proximal occlusions, large diffusion-perfusion mismatches and initial infarct volumes greater than 70 cc had significantly better clinical outcomes if they recanalized early, versus those who recanalized late or not at all.58 Another study, showed that patients with a clinical-diffusion mismatch (NIHSS scale >8 and DWI lesion volume <25 cc) and presentation beyond 8 hours of symptom onset could be successfully revascularized without intracranial hemorrhage or early neurologic deterioration.59
For patients for whom the stroke onset time is unknown, early MRI may also be helpful. One study assessed 32 patients with unclear onset time, little to no FLAIR changes, a DWI abnormality, and a PWI-DWI mismatch who were treated with IV rTPA within 3 hours and/or intraarterial urokinase within 6 hours of symptom detection. There was no difference in recanalization rates, early neurologic improvement, symptomatic intra cranial hemorrhage, or 3-month clinical outcome in these patients compared with 223 patients with known stroke onset time.60
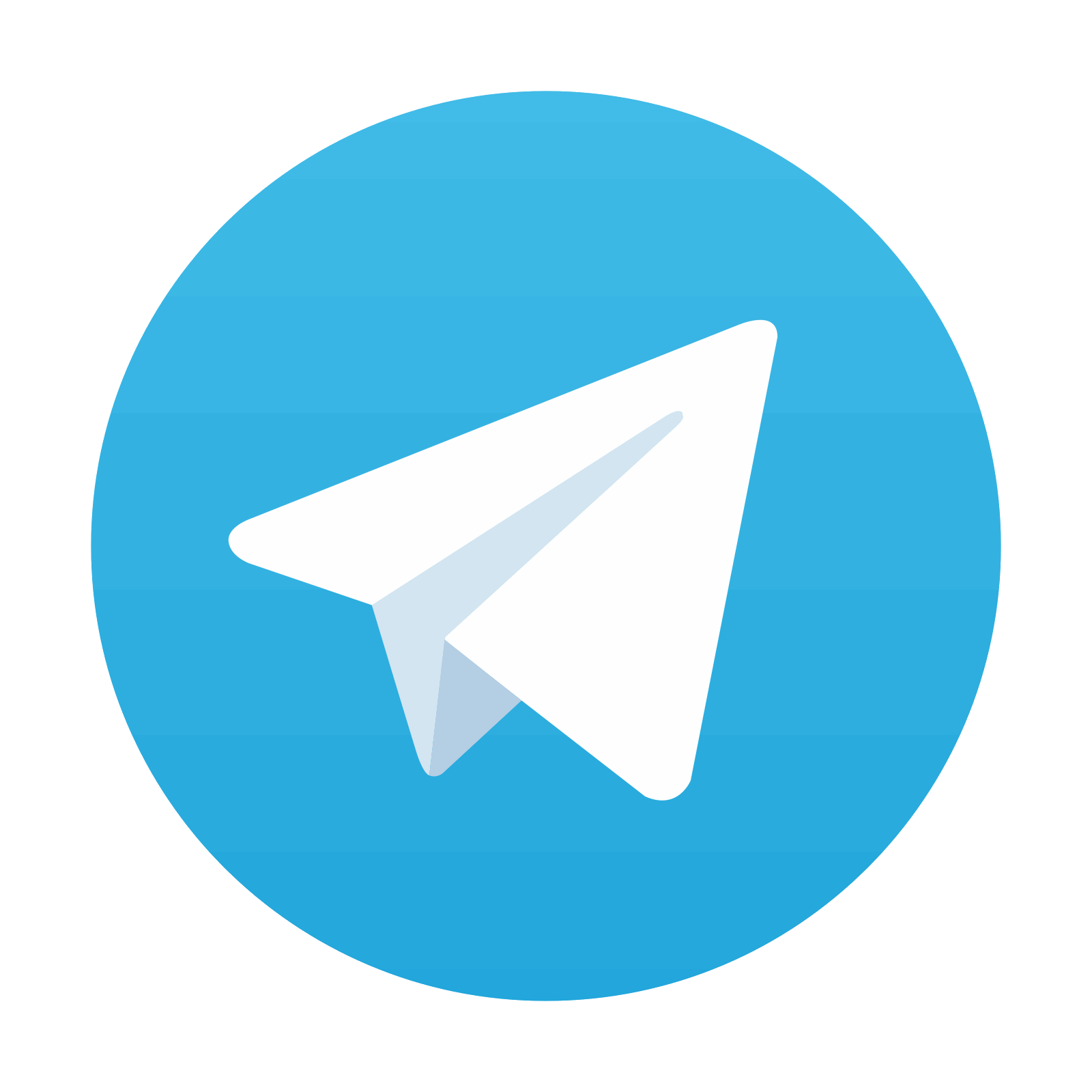
Stay updated, free articles. Join our Telegram channel

Full access? Get Clinical Tree
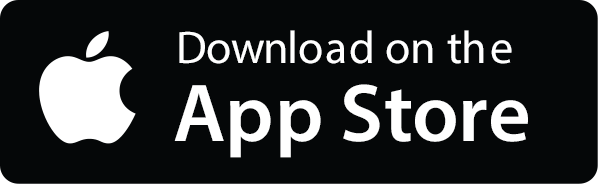
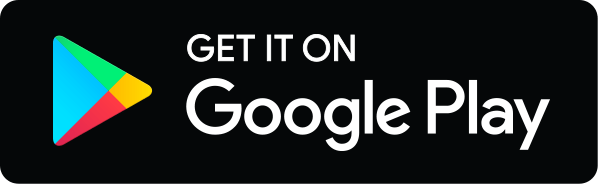
