Developmental Anatomy of the Normal Cervical Spine
Anthony S. Lapinsky
Mark S. Eskander
Henry H. Sherk
GENETICS
The in utero development of the spine is governed by a single family of genes. These genes were first discovered in the chromosomes of fruit flies, which had developed mutations manifested by two sets of wings. These genes, known as the homeobox or Hox genes, regulate the differentiation processes of the axial and appendicular skeletons. They are responsible for the embryonic differentiation and segmentation of the craniocaudal axis via activation and repression of DNA sequences that encode the transcription factors and proteins affecting the order and direction of development of the axial skeleton. Mutations of the homeobox genes may be responsible for congenital anomalies of the cervical spine. Each homeotic gene product controls a domain of 60 amino acids, known as the homeodomain, and the coding sequence is known as the homeobox. In the fruit fly, normal sequential expression of the homeobox genes results in the development of the head and proboscis, then the antennae, and then the thorax and attached wings, followed by the development of the abdomen. The genes that control this orderly development are activated in sequence, and the timing of the expression of each gene allows for the normal development of the head, thorax, abdomen, and related structures.
Mammals possess gene clusters similar to those of the fruit fly. These clusters, known as the Hoxa, Hoxb, Hoxc, and Hoxd genes, are found on chromosomes 7, 17, 12, and 2, respectively, in mice. As in the fruit fly, these genes are activated sequentially and are expressed maximally in neighboring segments of the developing individual. In mammals, however, the expression of the genes overlaps to a degree, so that a single genetic defect may not result in observable deformity of the spine or appendicular skeleton. In the fruit fly, the genetic control appears to be more structurally specific.
The homeobox genes continue to be the focus of research on normal and flawed development of mammals and other species, and new knowledge continuously becomes available. The processes of development, however, have been studied and well understood. The remainder of this chapter focuses on the developmental anatomy of the spine, particularly the cervical spine, during embryonic, fetal, and postnatal life.
EMBRYONIC DEVELOPMENT
The spinal column develops largely through the process of endochondral ossification, which is the culmination of a chain of events beginning in the 3rd week of gestation when mesenchyme differentiates out of the mesoderm into segmented somites. These structures develop on either side of the notochord; they distend the thin overlying ectoderm and are clearly visible as uniform blocks of tissues on either side of the developing individual (Fig. 2.1). During the fourth week of embryonic life, the somites differentiate into the sclerotome and myodermatomes. The former structure gives rise to the spinal column. The mesenchymal cells of the sclerotome migrate dorsally to give rise to the vertebral arches that eventually surround the spinal cord as the pedicles and laminae. Sclerotomal cells also migrate ventrally to form the vertebral bodies, disks, and costal processes (Fig. 2.2). At about 6 weeks of embryonic life, the sclerotomes undergo a metamorphosis, which heretofore has been termed the process of resegmentation. This event involves vertebration of the notochord and formation of disks to maintain flexibility and motion as well as to transmit the stresses of weight bearing (Fig. 2.3).
In resegmentation, the caudal half of one sclerotome is said to fuse with the cranial half of the adjacent sclerotome (Fig. 2.4), occurring at the same time that the mesenchyme of the sclerotomes migrates ventrally to surround the notochord. The combination of events results in formation of a vertebral body with intervertebral disks. This hypothesis focuses on the vertebral body as the major developmental spinal structure and implies that the intervertebral disk is a secondary element that arises from a splitting apart of the sclerotomes to permit motion of the spinal column. Resegmentation as a theory of development made it possible to explain the spanning of the disks by muscles of the myodermatome to provide for voluntary motion. The
muscles at each myodermatome, according to this theory, are related developmentally to the caudal half of the upper vertebra and the cranial half of the lower vertebra, crossing the intervertebral disk between the two halves.
muscles at each myodermatome, according to this theory, are related developmentally to the caudal half of the upper vertebra and the cranial half of the lower vertebra, crossing the intervertebral disk between the two halves.
The reality of spinal development is probably more complex. The intervertebral disk is probably the essential feature phylogenetically as well as ontologically, and the central portion of each sclerotome gives rise to the disk, which permits motion between vertebral segments. The vertebral body originates from a continuous mass of cells that surrounds the notochord to form a recognizable centrum. The caudal half of the more distal somite gives rise to a portion of the neural arch and the transverse process at each level, and the caudal half gives rise to the costal process. In the thoracic spine, this structure becomes a rib, but in the cervical and lumbar spine it forms part of the transverse process. The costal process component of the sclerotome also gives rise to the hypochordal bow. In the neck, this structure becomes the ventral arch of the atlas, but more distally it forms the thick portion of the anterior longitudinal ligament at each level. The costal process and the hypochordal bow are the vestiges of the hemal or ventral arch in other vertebrate species such as birds and reptiles. Voluntary motion activated by contraction of muscles is made possible by the spanning of the segments by muscles that link the transverse processes of the proximal vertebra with the transverse processes of the subjacent one (Fig. 2.5).
OSSIFICATION
Once the embryo has metamorphosed, the structure of the spinal column is established. The vertebral components are initially mesenchyme, but they rapidly begin the process of chondrification and then ossification. The former begins in the upper-level somites of the occiput and proceeds distally. Mesenchymal vertebral anlagen chondrify in dual centers on either side of the notochord and in the base of the laminae. The process of ossification begins at the cervicothoracic junction and proceeds caudally and cranially. Ossification in utero takes place in five centers, replacing the cartilage of the chondrified vertebral anlagen. These five centers are the centrum of the vertebral body, the two neural arches, and the two costal processes. At birth, in the cervical spine, the centrum, neural arches, and the costal processes at each level have ossified, but these structures have not fused (Figs. 2.6 and 2.7).
The formation of the upper cervical spine results in the development of the occipitoatlantoaxial complex, which although uniquely different from subaxial vertebrae reflects only a variation of the developmental process described earlier. The basiocciput arises from the fusion of the proximal occipital sclerotomes. These structures are perforated by the foramina of the roots to the twelfth (hypoglossal) cranial nerve. The apex of the dens and its ligaments arise from the fusion of the “cervical zero” (C0) sclerotome and the C1 sclerotome. The central portion of the dens reflects the fusion of the C0 and the C1 sclerotomes. The ventral arch of the atlas is the enlarged hypochordal bow of the C0 sclerotome; with the ventral aspect of the lateral mass of the atlas, it is the first contribution of the costal process to the structure of the mature atlas. The dorsal arch of the atlas arises from the C0 and C1 sclerotomes. The atlas vertebra at birth is ossified only in the lateral masses, but ossification proceeds rapidly in the ventral and dorsal arches on each side. These ossification centers usually fuse both dorsally and ventrally to complete the mature structure of the atlantal ring by late childhood (about 10 years); occasionally, however, a secondary ossification center develops in the ventral arch at age 6 years.
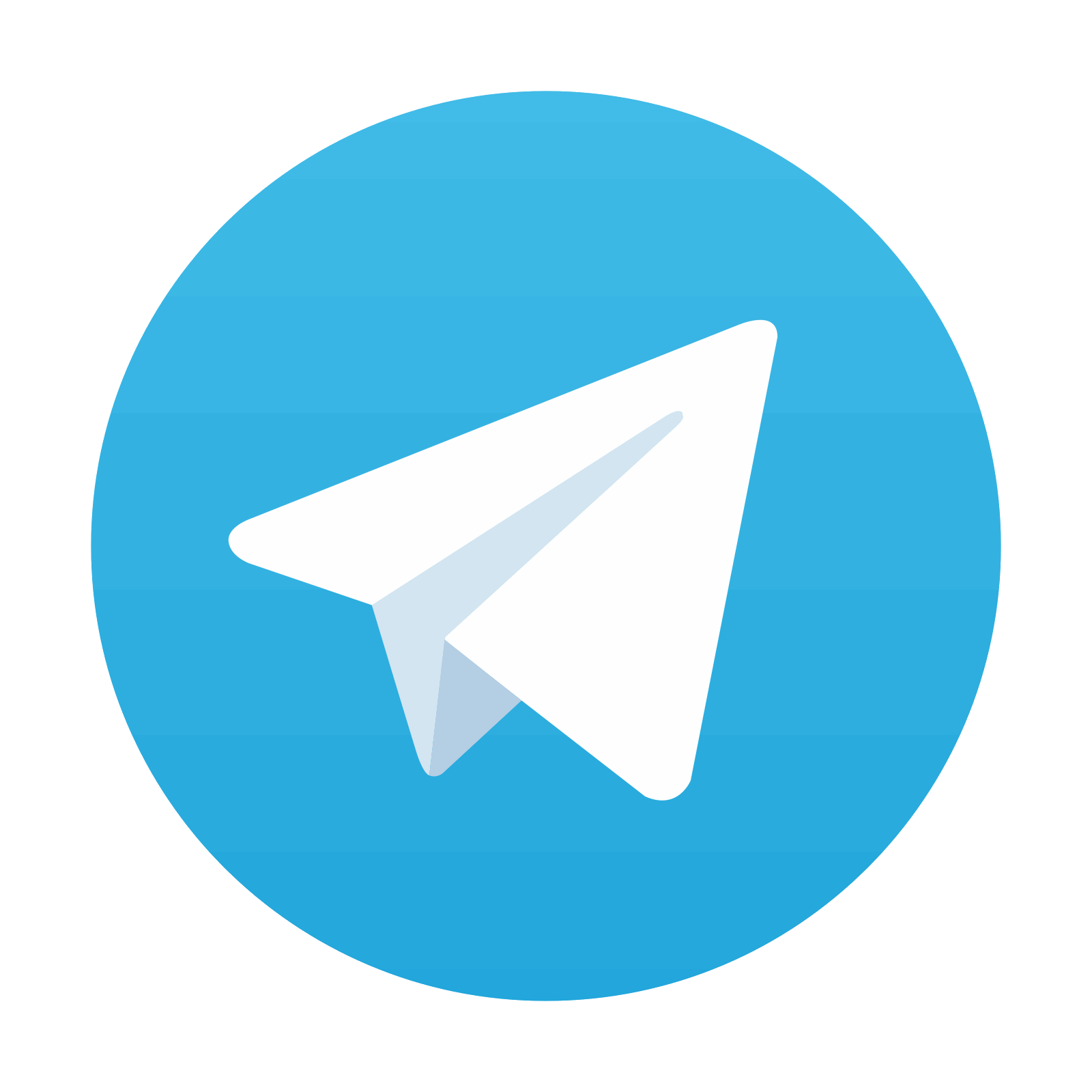
Stay updated, free articles. Join our Telegram channel

Full access? Get Clinical Tree
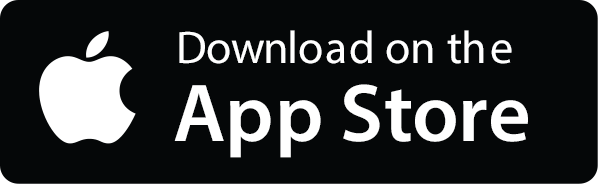
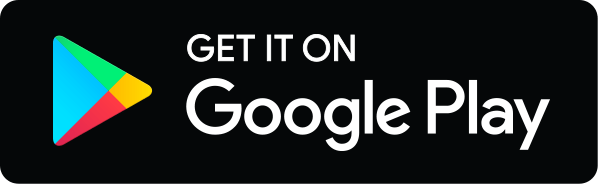