Introduction
In times past, tribal medicine men jealously guarded their secret knowledge, typically passing it from father to son, thereby protecting the family’s status and importance. Over the centuries mankind has come to realize that broadly sharing this critical knowledge benefits society. As a result, in today’s society more than 1 million people worldwide study medicine. Interestingly, the learning process is not radically different today than in years past: students apprentice under those with greater experience and learn by reading and practicing the taught skills.
Although this process is quite adequate to teach skills, it falls short with the ability to produce devices or medicines. Consider the carnage that may ensue if people were provided a recipe to make aspirin, or a pacemaker, or a monitor rather than being able to buy one whose efficacy, or functionality, is well understood. The key word is “buy,” which implies the existence of an entity willing to sell, possibly on a large scale. This is the focus of this chapter, namely, how to translate an idea for a medical product from a notion to a salable product.
Because this textbook is about monitoring, the focus of this chapter is on medical devices rather than pharmaceutical compounds. Although much of the material presented applies equally to both, the time and expense associated with drug development are typically considerably greater than that for medical devices. In addition, the business models may be different. It is not uncommon for a pharmaceutical start-up to be acquired before market introduction but after certain milestones are achieved, whereas medical device companies typically are not acquired until the product has successfully launched.
Much of the material in this chapter discusses the business and financial aspects of bringing a product to market, because most medical practitioners do not have a business background. By contrast, many medical practitioners have a scientific or engineering background and so only a general overview of the product development life cycle and some key development activities are discussed. However, the medical device development process has become more complex over the years in large part because of regulatory requirements, business decisions (reimbursement), and technology advances. This development process has recently been detailed ( Table 38.1 ) and practical ways to address specific challenges have been detailed in workshops of academia, government, and industry representatives. Several universities around the world also have developed dedicated educational programs in life science technology innovation. In addition, most books that address medical device development focus on the technical aspects of the process. The more business-oriented focus of this chapter complements these other sources.
|
Developing Medical Products
For new or established companies, the decision to pursue the development of a new product is never taken lightly because a significant commitment of the company’s resources is required. To bring a new drug to market, for example, may require the better part of a decade and cost more than $500 million. Therefore one of the most important aspects of the product development process is to determine if there is a need for the product. The need assessment is revisited frequently during the development process, whose steps are (1) idea generation, (2) conceptual design, (3) detail design, (4) clinical trials, and (5) manufacturing. This section outlines the activities that occur during each step of this process.
Idea Generation
All products available for purchase start as an idea in someone’s head. The original idea may have sourced from an engineer who realized that some technology could be of value to a medical practitioner or the idea may have sourced from a clinician who identified a need during the course of practice. These two camps are referred to as “technology push” and “market pull.” Technology push typically requires more time and effort to fully develop, but often may lead to a broad range of products, often more than imagined by the original investor. Market pull ideas typically are accepted more quickly into the marketplace, but usually do not lead to a broad suite of follow-on products.
Once a product idea has been formed, the concept must be tested. For medical devices the first tests performed are risk analyses—does the use of the product pose greater risk to the user than the problem it is intended to address? Assuming that the product does not pose unreasonable risk, the next task is to determine if the product meets customer needs. There are several methods to perform this assessment.
The easiest method, but the one that provides the least valuable information, is to perform secondary research, that is, to analyze published documents to understand the market. Although secondary research is important, it cannot truly answer the question, “If I make it, will they buy it?” To learn the answer to this question, the best approach is to ask potential customers, in particular, those with purchase authority. To maximize the value of the information obtained from potential customers, a structured approach, such as quality functional deployment (QFD) is recommended. The reason that this process is so powerful is that it is a well-defined process to translate customer needs to product features and keep them evident throughout the design process.
The final step in this phase of the design process is to thoroughly document the product requirements. Though there is some disagreement, requirements should state only what the product should do and not how it does it. This approach provides the broadest palette for conceptual designs.
Conceptual Design
Conceptual design is the process of structuring a solution to a design problem. During this stage the process is mostly qualitative, not quantitative. For instance, if one were designing a robotic surgical assistant, a choice between electric and hydraulic actuators would be decided during this phase. This decision may require some quantitative analysis. For example, “Can a motor fit within the space allotted and still provide sufficient motive force without requiring the user to specify part numbers, bolt patterns, and so on.”
For engineers, the conceptual design phase is usually the most enjoyable. Conceptual design requires great creativity, as a number of widely disparate approaches should be developed to ensure that the best ones are considered for further development. Methods used to generate ideas include (1) literature searches, (2) biomimicry, (3) reverse engineering of related products, (4) study of analogous systems, and (5) brainstorming, among others. Once a number of concepts have been developed, they are assessed against the requirements previously developed to determine those that best meet the specified needs.
At this juncture it is important to note several issues that are relevant to design of medical devices rather than other product types. In the United States, the Food and Drug Administration (FDA) regulates medical devices. Although entire books are written on the subject of complying with FDA requirements, the following two (and potentially three) issues greatly affect the development process.
Record Keeping
The Safe Medical Devices Act of 1990 added design validation requirements to the Good Manufacturing Practices (GMP) requirements. Fundamentally, the entity that designs a medical device must document the procedures used to ensure that the specified requirements are met. Procedures should be generic rather than detailed. That is, they should include major activities and assignments for subsystems, as opposed to detailing responsibility for component selection. It also is understood that design procedures change, thus the standard requires that an entity’s procedure be regularly reviewed and updated.
Device Class
All medical devices are categorized into one of three device classes:
Class I: These are non–life-sustaining devices whose failure poses no risk to life. Examples of class I devices include bandages, stethoscopes, and examination gloves. Most class I devices are exempt from the premarket notification or GMP regulation.
Class II: These devices are non–life sustaining; however, the controls in place for class I devices are insufficient to ensure safety and effectiveness. Examples of class II devices include catheters, powered wheelchairs, and many clinical chemistry test systems. Although some class II devices are exempt from premarket notification, others may require clinical studies as rigorous as an investigational device exemption (IDE) for premarket approval (PMA).
Class III: These devices are typically those that support or sustain human life and whose failure is life threatening. Examples of class III devices include heart valves, implanted electrical devices, and joint implants. The vast majority of class III devices require PMA, a process that includes laboratory testing, animal testing, failure mode analysis, manufacturing standards, and safety and efficacy testing as determined in a human clinical trial. Needless to say, to obtain PMA can be a long, costly process.
When setting out to develop a medical device, the design team should determine the device class, as early as possible so that the planning process can incorporate the appropriate activities into the overall project plan. For new devices for which precedent is unclear, the design team should submit a Request for Determination to the FDA.
Extent of Business Activities
In the grossest sense, the activities needed to bring a device to market are conception, design, production, distribution, and support. A key decision for a new business entity is to determine which of these activities are to be performed in-house and which are outsourced. This decision has two major impacts: first, the amount of investment needed, and second, the FDA regulations that must be addressed. For many companies, outsourcing manufacturing offers a good blend of activities, allowing the company to minimize capital costs, earn a reasonable rate of return, and reduce FDA regulatory issues (though the manufacturing partner must comply with FDA requirements).
The conceptual design phase ends with a design review that presents the requirements, the concepts developed, and the concept(s) selected for further development.
Detail Design
Most novel designs includes one, and sometimes more, elements that have not been previously realized. For example, lab-on-chip devices replace manual fluid introduction and mixing with microfluidic components. Before the design for the final product is completed, the development team typically performs a series of experiments to validate the functionality of the novel component. Staying with the example, do the microfluidic devices deliver sufficient head and flow to provide sufficient agitation?
Once all of the subsystems are known to work, it is common to couple all of them together in a bench-top prototype to assess overall system functionality. This prototype may bear little physical resemblance to the final device. However, the manner in which it operates is analogous. Depending on the nature of the product, the bench-top prototype may be used to perform in vitro or in vivo testing, again providing further evidence of the device’s safety and efficacy.
Once this testing is completed, the team should have sufficient information to design the final device. This detailed design consists of all of the information necessary to reliably and repeatedly produce the device. Often, methods such as Design for Six Sigma are used to help ensure that changes in performance due to manufacturing variations do not result in performance falling outside the specified bounds. This design phase ends with a review that presents the results of the experiments performed, the detail design, the bill of materials, and how these perform together to meet the design specifications.
Clinical Trials
Certain classes of medical device must undergo clinical investigation before the FDA provides marketing approval. Animal model testing often is performed before human trials, especially in those cases for which safety and efficacy are unknown. Animal use to test a device is a privilege and numerous controls exist to ensure that no more than the minimum number of animals are used, that they are properly housed and cared for, and that upon the conclusion of the experiment, they are humanely euthanized.
The institutional review board of the facility that oversees the trial must approve all devices that undergo human clinical investigation. In addition, the FDA must approve trials for significant risk devices through submission of an IDE. The IDE exempts the manufacturer from certain portions of the Food, Drug, and Cosmetic (FD&C) Act during the trial phase. However, the manufacturer is still required to comply with all design controls and all necessary manufacturing controls to ensure patient safety and compliance with the manufacturer’s quality claims.
The goal of a clinical trial is to determine the safety of a device and its effectiveness in terms of the intended claims. This requires concise and specific definition of the study’s objectives. The objectives must consider factors such as comparison to approved modalities of treatment or whether it treats a symptom or an underlying cause. Once these factors are known, details of the trail design, such as study size, candidate pool, and controls, among others can be formulated. In addition, trials must be carefully designed to minimize errors and biases. From a business perspective, trial design is critical because the claims that the business wishes to make about its device must be supported by clinical outcomes.
Manufacturing
Once the design is completed, a pilot run is performed to validate manufacturing processes. This is the first build of the device in its final form using the manufacturing documentation. This part of the process also includes assembly workforce training, validation of supplier plans, and checking that manufacturing objectives have been achieved.
A critical aspect of this phase is product packaging and labeling. Packaging must be designed to ensure that the product reaches the end-user in an appropriate condition (which typically means that the product maintains sterility) and that the package can be easily opened. Labeling includes all printed or graphic material that accompanies a product and advertising. Depending on the type of product, labeling requirements may include the manufacturer’s contact information, intended uses, and directions for use, to name a few.
Once all of this information is available, it is submitted to the FDA, either as a 510(k) premarket notification submission or a PMA submission. Approval from the FDA is required to legally offer the product for sale in the United States. Other regulations apply in other countries. However, FDA approval is not necessarily sufficient for the product to gain acceptance. In the United States, much of the cost for medical products and procedures is paid for by third-party insurers. Underlying these payments is a list of codes that source from the Centers for Medicare & Medicaid Services (CMS). Because codes are defined in terms of services provided, and not specific devices, new products may be covered by existing codes. However, novel devices may require the development of new CMS codes, and without these codes, many practitioners may be hesitant to adopt a new device.
Financing Product Development
A corollary to Murphy’s law states, “The first 90% of the task takes 90% of the money, and the last 10% takes the other 90%.” Based on the previous discussion about medical device design and development, this statement may, in fact, be overly optimistic. Depending on the type of device, the development process can require years and tens of millions of dollars. Because most medical device inventors are not independently wealthy, outside investment often is required to bring a new medical device to market.
The world of outside investment can be broadly divided into two categories: those who invest primarily to obtain a (hopefully very high) return on their investment and all others. The former are typified by angel investors and venture capitalists; the latter by universities, federal and state governments, family, and others. Angel investors and venture capitalists play such an important role in medical device development that the entire next section is devoted to them. This section starts with some general guidance about business practices and then discusses non-equity investment opportunities.
The founder of a business focused on developing a new medical device is convinced of the value and importance of the invention. However, Ralph Waldo Emerson’s adage that if one “build[s] a better mousetrap and the world will beat a path to your door” is not always true. It takes considerable effort to convince others that the potential product will both address an important medical need and produce a profit. Simply providing experimental data is insufficient because the inventor will be asking potential investors to trust him or her with millions of dollars.
To access outside funding of most any sort, a company must have a business plan. There are innumerable books about writing business plans (see further suggestions later in this chapter). Two cautionary notes: first, most business plan software programs should be avoided, because they typically do not add much value. Second, the writing of the plan should not be outsourced because the entrepreneur must know the entire plan, the sources used to develop it, and so on. Contained within the plan is a financial model, arguably the single most important aspect of the plan. Attention should be focused on building a rational model, because potential investors will be more interested in the assumptions that underlie the model rather than the actual number presented.
As a final cautionary note, inventors who intend to seek outside investment should engage accountants and attorneys. Although it is true that forming a business requires little more than completing a simple form, the business’s rules of operation (referred to as an operating agreement for limited liability companies [LLCs] and bylaws for corporations) dictate how the business is run. If these rules of operation are not professionally written, they may impede potential investments. Similarly, an accounting system that does not adhere to generally accepted accounting principles (GAAPs) will make it very difficult for a proper financial analysis to be performed, which again may impede potential investments. As a technology-focused business, the most valuable assets are the inventor’s intellectual property. Although it is certainly possible to apply for patents and trademarks independently, engaging an intellectual property (IP) attorney is strongly recommended to ensure that the protection sought is as broad as possible. Finally, an attorney should review all dealings that can materially affect the business, such as grants of corporate ownership, licensing agreements, and partnering deals, among others, before being executed.
University Research
If the developer is a university employee when the medical device idea is first invented, the first stop is the school’s office of technology transfer. Since the passage of the University and Small Business Patent Procedures Act (also known as the Bayh-Dole Act) in 1980, most universities have created offices that deal exclusively with licensing and IP. For a university inventor, working with the office of technology transfer provides several important benefits. First, as dictated by the Bayh-Dole Act, if the research was funded by the federal government, the university has the right to retain ownership of the IP created. As such, should the technology be commercialized, the inventor will typically receive financial remuneration as a percentage of the licensing revenue earned by the university. Second, the inventor of the IP is often granted first right-of-refusal with regard to licensing the technology for commercial purposes. Often, for faculty-founded start-ups, the university will provide favorable terms, such as defraying patent prosecution costs until the company has revenue. Third, the office of technology transfer may have specialists whose role is to help with company formation. These individuals can provide guidance with business plan writing, recommendations for local service providers, and identification of funding opportunities.
The passage of the Bayh-Dole Act also has motivated universities to commercialize technology. Universities with active technology transfer offices can generate tens of millions of dollars annually in license fees, royalty, and equity. To capitalize on this opportunity, a number of states and universities have created funding sources for university inventors to assist them to bring their inventions to market. Examples of such programs include the following:
Boston University Ignition Award and Launch Award : The former helps bridge the gap between basic science and the product development and the latter is designed to help faculty members start new companies based on technologies that they invented at the university.
Michigan Universities Commercialization Initiative : A collaboration designed to complement and enhance the technology transfer at Michigan academic and research institutions by supporting commercialization of IP.
University of Utah Technology Commercialization Project : Focused on further developing novel technologies that are near commercialization in all areas of technology.
For the university inventor, such initiatives offer great value. First, the competition for funds is limited to a small pool of applicants, thus the odds of funding are relatively high. Second, the peer-review process typically used provides some validation of the commercial potential of the technology. Third, the funds often can be used for non–technology-focused activities, such as engaging business and marketing professionals from outside the university, which helps to accelerate the growth of the business. The only downside to these programs is that the funding available is typically limited.
Government Research Funds
Growing a technology-focused start-up from inception to profitability is a very high-risk proposition. Though exact figures are not available, it is estimated that less than one quarter of all businesses are still operational after 10 years—for technology-focused start-ups, the number is smaller. On the other hand, new products and companies account for a disproportionate fraction of a country’s gross domestic product (GDP). As such, all levels of government are incentivized to support early-stage companies because of the potential return on investment. In the United States, one federally mandated program that supports small businesses dominates all others: the Small Business Innovation Research (SBIR) program (and the very closely related Small Business Technology Transfer [STTR] program).
Originally authorized by Congress in 1982, key objectives of the programs are to stimulate U.S. technologic innovation, create new opportunities for small businesses to participate in federally sponsored research and development, and increase private-sector commercialization of innovations derived from federal research and development (R&D). As legislated, all federal agencies whose extramural research and development budgets exceed $100 million have a mandated set-aside of 2.5% of their budget to fund SBIR projects. In addition, all federal agencies whose extramural research and development budgets exceed $1 billion have a mandated set aside of 0.3% of their budget to fund STTR projects. In fiscal year (FY) 2007, 12 agencies participated in the SBIR/STTR program, with total funding of $2.315 billion.
Of the 12 participating agencies, the Department of Defense (DOD) accounted for 54.9%, the National Institutes of Health (NIH) accounted for 28.1%, and the National Aeronautics and Space Administration (NASA), Department of Energy (DOE), and National Science Foundation (NSF) combined accounted for 14.2% of the of the total funding, respectively. Developers of medical devices would typically focus on applications to the NIH; however, the DOD and NSF occasionally seek medical device applications as well.
SBIR/STTR programs are three-phased programs, as outlined in Figure 38.1 . The durations and dollar figures are statutory guidelines—each agency sets its own rules.

Full access? Get Clinical Tree
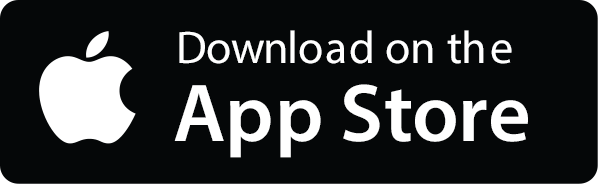
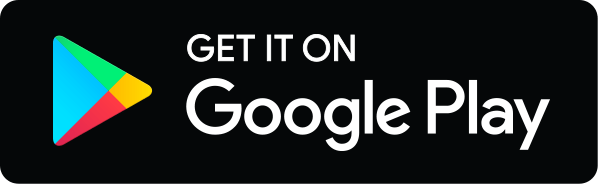