Fig. 9.1
Chiari 0 malformation and congenital atlanto-occipital fusion. T1-weighted midsagittal MRI in a 62-year-old woman with headache, dizziness and back pain (a) demonstrates that the cerebellar tonsils extend 4 mm inferior to the foramen magnum and that the odontoid is retroflexed 7 mm. A syrinx is seen (long white arrows, a, b). Fusion of the lateral mass of C1 and the occipital condyle is seen (short white arrow, c). MRI 3 months after suboccipital craniectomy, C1 laminectomy and duraplasty shows that a CSF space was created dorsal to the cerebellar tonsils (black arrowheads, d) and that the syrinx has resolved (long white arrow, d, e). Axial CT cuts (h–q) through the craniocervical junction demonstrate the foramen magnum (black arrowhead, h), vestigial right side of the arch of C1 (black arrow, j–l) and congenital atlanto-occipital fusion on the right side (o). Reconstructed 3-dimensional CT (f) confirms spina bifida occulta of the dorsal arch of C1 (black arrow). MR angiogram demonstrates a dominant left vertebral artery (LVA, g) and a normal left internal carotid artery (LICA) and RICA (right internal carotid artery)
CSF pathway narrowing across the subarachnoid space at the level of foramen magnum, shortened posterior fossa bones, decreased posterior fossa height and caudal brainstem displacement can be noticed in both Chiari type 0 and 1 malformations (Bogdanov et al. 2004; Tubbs et al. 2001), suggesting a similar pathophysiological mechanism for both types of malformations, in which posterior fossa underdevelopment and caudal hindbrain displacement impair CSF dynamics and lead to syringomyelia development. Although phase-contrast cine MRI (Figs. 9.2 and 9.3) has helped clarify the pathophysiology of syringomyelia, anatomical MRI alone usually provides sufficient information to diagnose and make appropriate treatment decisions about syringomyelia (Enzmann and Pelc 1991; Heiss et al. 1999; Oldfield et al. 1994).
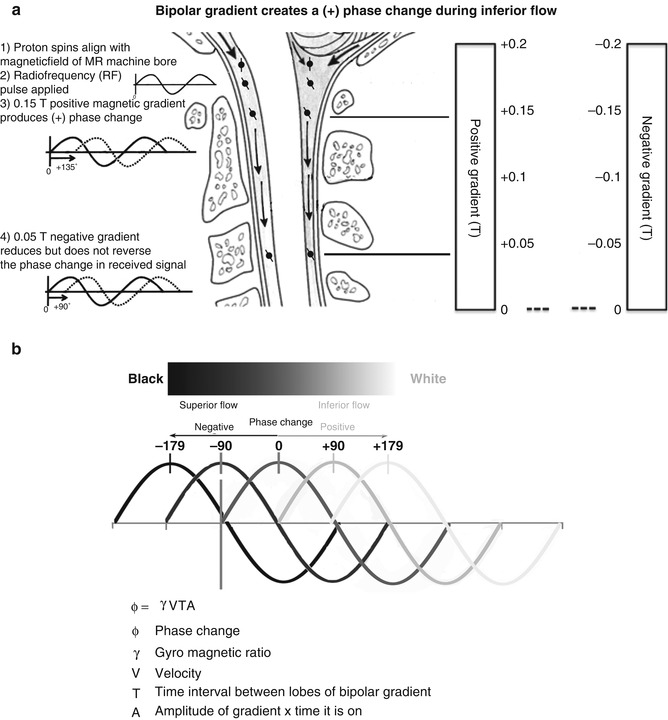
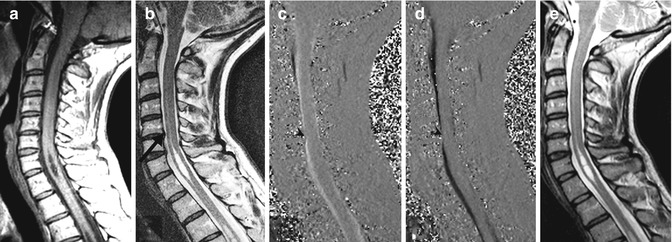
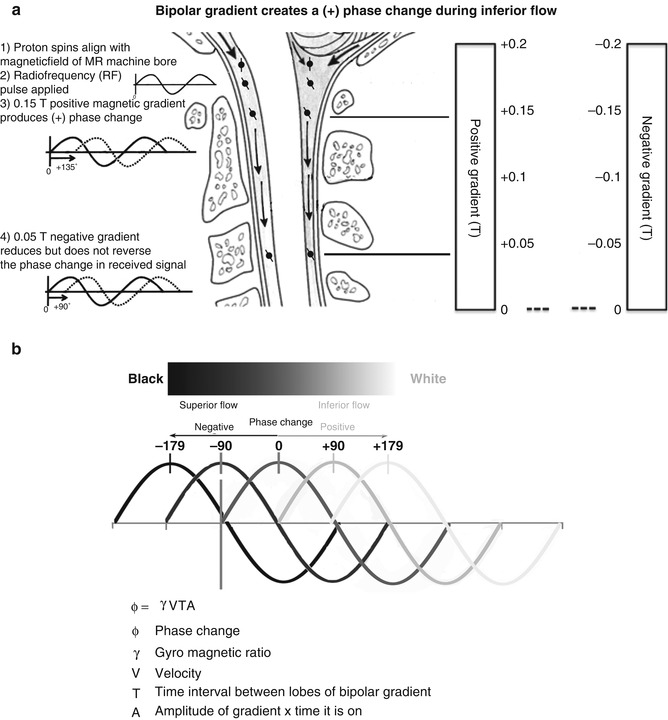
Fig. 9.2
Drawing of the craniocervical junction annotated to explain detection of CSF motion using phase-contrast cine MRI . In (a), protons in the water molecules of CSF align with the static magnetic field of the MRI machine (1). A radio-frequency pulse is delivered, which resonates with the protons (2). Two opposing magnetic field gradients, positive (3) and negative (4), are delivered. The inferior motion of the proton results in a greater positive than negative magnetic field being applied to the proton, resulting in a change in the phase of the radio-frequency signals that are released as the protons relax and realign with the static magnetic field. In (b), the phase change results in black (superior flow) and white (inferior flow) areas on the phase-contrast cine-MRI images. The intensity of phase changes is directly proportional to velocity
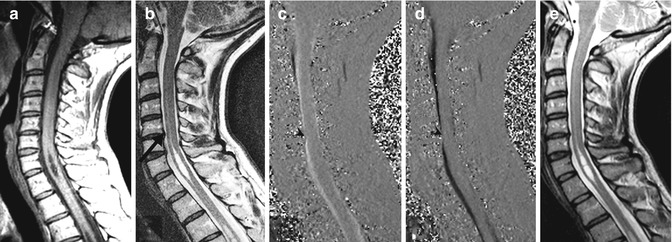
Fig. 9.3
Lack of syrinx fluid motion in a patient with stable cervical syrinx. A 41-year-old woman with moderate neck pain and no neurological deficit. T1-weighted and T2-weighted MRI (a, b) on presentation demonstrate mild central protrusion of the C5–6 intervertebral disc (arrow, b). Phase-contrast cine MRI during systole (c) or diastole (d) shows reduced CSF flow in the anterior subarachnoid space adjacent to the C5–6 disc protrusion (arrowheads) and absence of syrinx fluid motion. On re-evaluation 1 year later, neck pain persisted, but she remained free of neurological deficit. T2-weighted MRI at that time (e) demonstrates stable syrinx and disc size
Primary spinal syringomyelia is suspected when anatomical MRI scanning detects syringomyelia in the absence of pathology at the craniocervical junction, particularly if there is a history of antecedent trauma or meningitis (Batzdorf 2005). Anatomical MRI can evaluate for a spinal deformity or an extramedullary tumour associated with this type of syringomyelia (Byun et al. 2010; Holly et al. 2000), but obstructions in the spinal subarachnoid space, caused by focal arachnoiditis or arachnoid cysts, may not be detected by anatomical MRI, and additional testing with myelography, CT myelography and phase-contrast cine MRI may be required (Fig. 9.4). Sometimes, syringomyelia is not associated with any imaging abnormality and may be declared idiopathic (Lee et al. 2002; Nakamura et al. 2009). This topic is dealt with in Chap. 12.
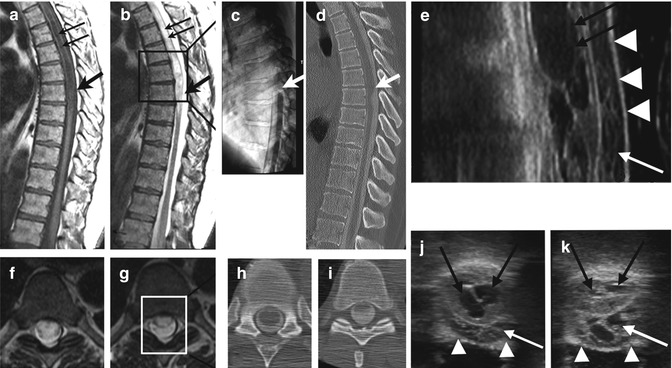
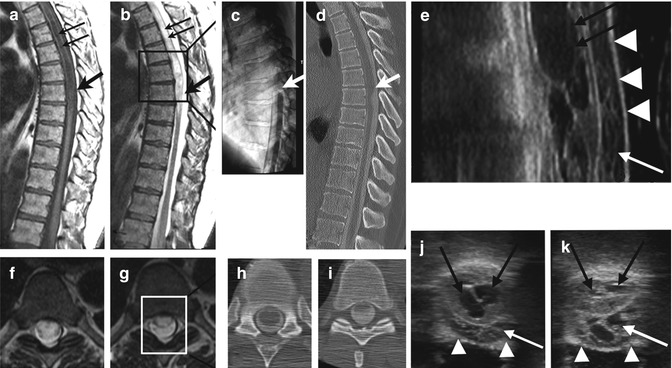
Fig. 9.4
A 63-year-old man with complaints of lower body numbness and tingling. Midsagittal T1-weighted (a) and T2-weighted MRI (b) demonstrates a thoracic syrinx (thin black arrows) and the dorsal subarachnoid space (thick black arrow). Axial T2-weighted MRI demonstrates expansion of the thoracic spinal cord by the syrinx (f) and signal changes in the dorsal subarachnoid space (g). A myelogram (c) demonstrates a block to the superior flow of contrast (thick white arrow) that proved incomplete on the CT-myelographic images (d, h, i). Note flattening of the dorsal surface of the spinal cord (i). Intraoperative ultrasonography in the lateral and axial planes (e, j, k) performed after laminectomy shows the dorsal dura (white arrowheads), an intricate web of membranes within the dorsal subarachnoid space typical of focal arachnoiditis (white arrows), and the multiple compartments of the syrinx (thin, black arrows). Lysis of adhesions and duraplasty resulted in stabilisation of symptoms and syrinx resolution (not shown)
9.2 Plain Films
Plain radiographs are no longer routinely used to evaluate syringomyelia but may still detect an associated scoliosis and may identify skeletal deformity from spinal trauma that may be associated with post-traumatic syringomyelia (Rossier et al. 1985). Although plain films may also discover basilar invagination , they do not show the resulting effects on adjacent neural elements. Flexion-extension plain films of the cervical spine are still performed if instability or hypermobility of the craniovertebral junction or cervical spine is suspected.
9.3 Computerised Tomography (CT)
Computerised tomography is used when bony malformation, fracture or deformity is suspected in association with syringomyelia (Fig. 9.1). Contiguous two-dimensional CT images can be reformatted into a 3-dimensional volume, which can be examined in any plane to give a better understanding of the integrity of the bony elements of the spine and craniocervical junction. Such reconstructions can be used in complex cases to decide whether spinal instability is present or might be induced by a proposed surgical procedure. They can be used to plan the placement of instrumentation for fusion of unstable craniospinal or spinal segments.
9.4 Myelography
Myelography is performed by injecting a contrast medium into the spinal subarachnoid space and following its movement through the spinal canal, using fluoroscopy and capturing radiograms of the silhouette created around the spinal cord and nerve roots (Fig. 9.4). Water-soluble contrast media are presently used exclusively, and the use of both air- and oil-based contrast media in myelography has long been abandoned. This is because air produced inferior contrast and higher rate of headache and oil-based myelography can evoke spinal arachnoiditis. The development of myelography in the pre-CT, pre-MR era nevertheless makes for interesting reading and is the subject of Chap. 21.
Contrast medium is typically injected via lumbar puncture and advanced superiorly in the spinal subarachnoid space by tilting the fluoroscopy table. Because water-soluble contrast medium is a liquid solution that is denser than CSF, declining the myelography table to lower the head in relation to the lumbar spine results in the contrast moving superiorly in the subarachnoid space, outlining the conus medullaris, followed by the thoracic spinal cord, cervical spinal cord and cervicomedullary junction. A lesion completely obstructing the subarachnoid space prevents contrast from moving superiorly, thereby demarcating the inferior margin of the lesion. Lesions associated with syringomyelia, however, usually, allow some contrast to pass, and the X-ray table can then be inclined, to run the contrast inferiorly once again, in order to outline the superior margin of the lesion. In cases in which CT myelography via lumbar puncture cannot define the superior margin of a spinal lesion, contrast can be injected additionally via cervical (C1–2) puncture and passed inferiorly to this level.
When myelography is followed immediately by a spinal CT scan, in the region of the partial or complete obstruction, images obtained provide greater spatial resolution and more conclusive identification of tonsillar ectopia associated with Chiari I malformation than provided by myelography (Grigorian and Lisianskii 1988; Yamada et al. 1981) (Fig. 9.4h, i). Thin-cut axial CT-myelographic images can then be reconstructed and viewed in any plane.
Myelography and CT myelography are still used in the diagnostic evaluation of patients with primary spinal syringomyelia in whom obstructive lesions cannot be identified using anatomical MRI or phase-contrast cine MRI (Fig. 9.4). In such cases, myelography often localises surgically remediable syringomyelia caused by a lesion, which partially or completely obstructs the subarachnoid space. Careful observation of dye movement during myelography is essential to localise a partial blockage of CSF flow by focal arachnoiditis, as CT-myelographic images in such cases may appear normal if dye passes the partial block and opacifies the subarachnoid space above a partial blockage (Mauer et al. 2008) (Fig. 9.4). CT myelography is often helpful in outlining arachnoid webs, pouches and cysts associated with syringomyelia and in demonstrating distortion of the spinal cord by these processes (Mallucci et al. 1997).
9.5 Magnetic Resonance Imaging (MRI)
9.5.1 The Physics of MRI
The human body is mostly made up of the elements hydrogen, carbon and oxygen, including 60 % as water and variable amounts of fat (lipids). The nucleus of each hydrogen atom is a proton, which is positively charged, has the quantum property of spin and has a magnetic moment. Placing protons in the static magnetic field of the MRI unit aligns the protons so they precess, or “wobble”, about the main axis of the magnetic field. The precession frequency is directly proportional to the strength of the magnetic field according to the gyromagnetic ratio .2 In magnetic resonance imaging, radio-frequency (RF) pulses, delivered at this specific precession frequency (the Larmor frequency ), are absorbed by protons. After the RF pulse stops, the protons relax to their resting alignment by emitting the absorbed RF energy. The emitted RF signal varies according to the local tissue environment and various other physical parameters (e.g. T1 weighted, T2 weighted, diffusivity and proton density).
Three orthogonal magnetic field gradients (magnetic fields that change in intensity linearly over the body), known as the slice-select, phase-encoding and frequency-encoding gradients, are used to localise the received RF signal in space. These gradients alter the strength of the magnetic field over the body, and consequently, the Larmor frequency will vary across these regions. The bandwidth of RF delivered to the protons, in the imaged structure, must therefore be sufficient to span these frequencies. After applying the RF pulse, the relaxation of protons in the varying magnetic fields results in the emission of different frequencies of released RF energy from the imaged structure. These are used to encode the RF signal in three planes (X, Y and Z). The released RF signal is received by an antenna (coil), converted from analogue to digital form and then changed mathematically, using Fourier transformation, from an intensity-and-time function to an intensity-and-frequency function. A powerful computer analyses the digital signal and calculates intensity values for each spatial location in the imaging volume. It then creates and displays grey-scale images that represent the magnetic resonance characteristics of normal and abnormal anatomical structures within the imaging volume (Hashemi et al. 2010).
Phase-contrast cine MRI of cerebrospinal fluid velocity is often referred to as a CSF flow study . This phase-contrast MRI technique delivers an RF pulse followed by sequential positive and negative magnetic gradients. These produce a net positive or negative phase change in the RF emission from moving protons but no net phase change from stationary protons (Fig. 9.2). The sign of the phase change (positive or negative) indicates the direction of fluid (proton) movement. The magnitude of the phase change indicates the velocity of moving fluid (Fig. 9.2b). Between 16 and 32 images are produced, displaying CSF velocity throughout the cardiac cycle , with each image being labelled with its temporal relation (ms) to the R wave of the electrocardiogram. When this set of images is viewed as a cine loop, the CSF oscillation, which repeats every cardiac cycle, is visualised, with CSF moving inferiorly during systole and superiorly during diastole (Enzmann and Pelc 1991) (Fig. 9.3c, d). Phase-contrast cine MRI can be used to identify regions of reduced CSF movement at the level of the foramen magnum or within the spine which may be associated with syrinx formation (Heiss et al. 1999; Oldfield et al. 1994).
9.5.2 Anatomical MRI
T1-weighted images are anatomically accurate and clearly identify fluid within a syrinx cavity and CSF pathways (Fig. 9.3a). Measurements of the width of the CSF channels are more accurate using T1-weighted, as compared with T2-weighted, imaging (Fig. 9.3b). Although uncommon, communications between the fourth ventricle and syrinx and between the subarachnoid space and syrinx may be detected with T1-weighted imaging (Beuls et al. 1996; Bogdanov et al. 2000, 2006). Postoperative T1-weighted MRI can also reveal the location of a shunt tip within a syrinx (Schwartz et al. 1999a).
The presence of an intramedullary tumour associated with a syrinx is detected by giving intravenous gadolinium contrast, which escapes from permeable tumour capillaries and produces high signal within the lesion.
T2-weighted imaging is more sensitive to the presence of excessive fluid within CNS tissues than is T1-weighted imaging. Fluid can form and expand both the extracellular and the intracellular spaces of the CNS (Table 9.1). T2-weighted imaging may detect, in particular, a “pre-syringomyelia” state, which is spinal cord oedema from a chronic condition. If left untreated, this may eventually result in syrinx formation (Fischbein et al. 1999; Jinkins et al. 1998; Levy et al. 2000).
Table 9.1
Abnormal accumulations of water in CNS tissues
Increased pressure within a CSF space: e.g. transependymal movement of CSF from the lateral ventricles to the brain, caused by hydrocephalus |
Opening of the blood–brain barrier or blood–spinal cord barrier: caused by an intra-axial brain tumor or intramedullary spinal tumor (vasogenic oedema) |
Increased venous pressure: caused by an arteriovenous fistula or malformation |
Cytotoxic ooedema: fluid can accumulate within the intracellular space of the CNS when ischemia results in swelling of neural cells |
Fast imaging employing steady state acquisition (FIESTA )3 MRI produces high contrast between CSF and structures contained within the subarachnoid space, such as nerve roots. It uses short acquisition times to reduce motion artefacts (Chavez et al. 2005). It is particularly useful in studying syringomyelia, outlining arachnoid webs and cysts lying within the subarachnoid space.
Diffusion-weighted MRI (DWI) identifies diffusion of water molecules, which is less restricted within the extracellular than the intracellular space. This technique and its related sequences, diffusion tensor imaging (DTI) and fractional anisotropy (FA), have been used primarily as research tools in syringomyelia, to evaluate the integrity of white matter tracts (Roser et al. 2010a; Ries et al. 2000) and to identify injury in the spinal cord earlier than is possible with T1-weighted and T2-weighted images (Schwartz et al. 1999b). DWI of the spinal cord has lower resolution than brain DWI because of the smaller size of the spinal cord and the artefacts created by CSF flow and cardiac and respiratory motion around the spinal cord (Clark et al. 2000). In a patient with multiple sclerosis , a large cervical syrinx and a stable neurological deficit, DTI confirmed preservation of the white matter tracts around the syrinx (Agosta et al. 2004). In a study comparing 28 patients, with cervical syringomyelia and sensory dysfunction, to 19 normal volunteers, the patients had lower fractional anisotropy, which correlated with loss of pain and temperature sensation in their hands and prolongation of their spinothalamic conduction time on electrophysiological studies (Hatem et al. 2009).
Other MRI studies are mostly of research interest. These include volume imaging, using extremely thin (1 mm thickness) MRI slices, to improve anatomical detail and appreciation of communications between the fourth ventricle and syrinx and between the subarachnoid space and syrinx.
9.5.3 MRI Flow Studies
Phase-contrast cine MRI measures CSF motion that arises from the brain pulsations that occur during the cardiac cycle (Fig. 9.5). Brain expansion during cardiac systole results in CSF being driven caudally, from the intracranial subarachnoid cisterns into the more compliant spinal subarachnoid compartment. Relaxation of the brain then results in the cephalad movement of CSF, back across the foramen magnum, during diastole. Syrinx fluid also moves during the cardiac cycle, in response to spinal CSF pressure waves and spinal cord motion.
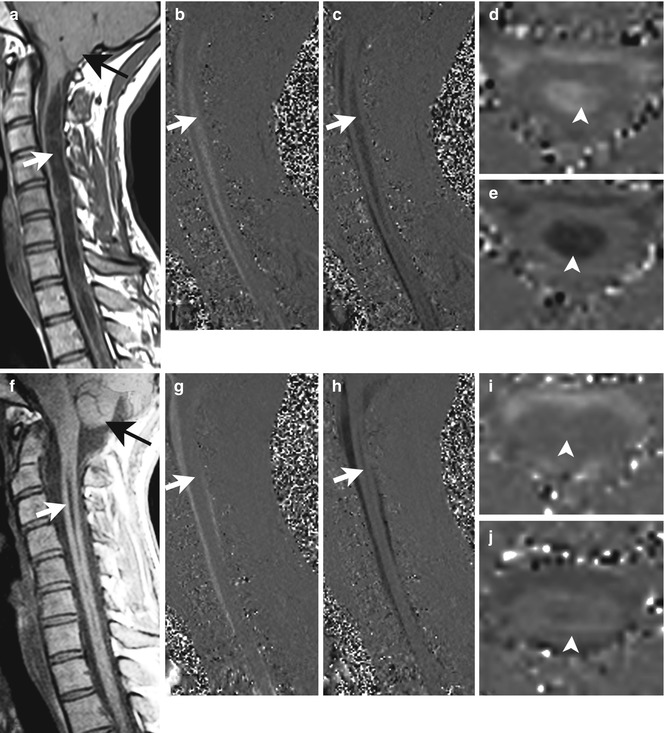
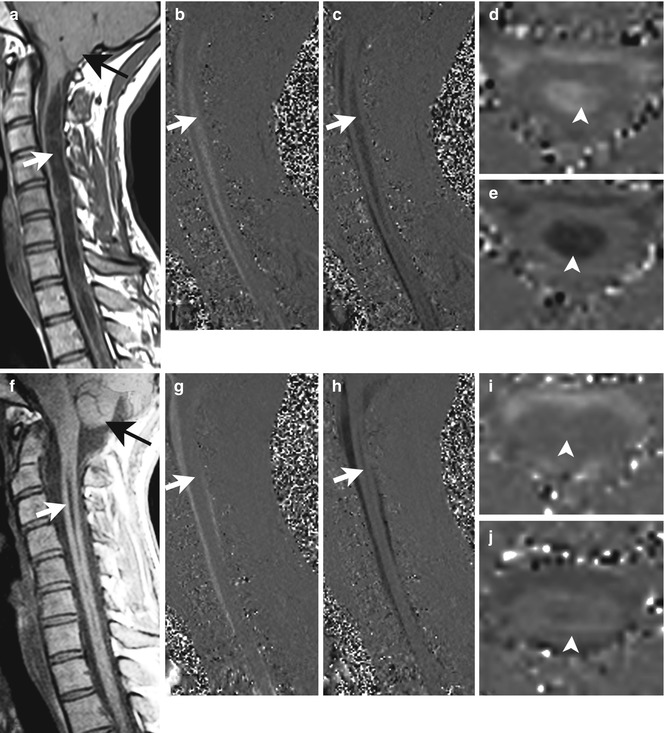
Fig. 9.5
Phase-contrast cine MRI . A 29-year-old woman with weakness, sensory loss and pain in both upper extremities. Before surgery, T1-weighted midsagittal MRI (a) shows the cerebellar tonsils (black arrow) to be located 11 mm below the foramen magnum, and a distended syrinx (white arrow) is present. Phase-contrast cine MRI in the midsagittal plane demonstrates flow in the syrinx (white arrow) in systole (b) and diastole (c). Phase-contrast cine MRI in the axial plane confirms syrinx fluid motion (d, e, arrowheads). Three months after surgery, the cerebellar tonsils have ascended several millimetres (f, black arrow), and the syrinx has become much smaller (f, white arrow). Syrinx fluid velocity is decreased compared to before surgery (white arrow, g, h; arrowheads, i, j)
Cine imaging can measure both CSF and syrinx fluid velocities in a defined region of interest. In patients with hindbrain-related syringomyelia, preoperative cine phase-contrast MRI demonstrates a reduction in CSF flow at the level of foramen magnum, in both Chiari 0 and Chiari I malformations. This improves after posterior fossa decompressive procedures (Iskandar et al. 1998) (Fig. 9.5). Progressive neurological symptoms are more likely when cine MRI demonstrates movement of syrinx fluid. Stable or absent symptoms are associated with lack of fluid flow within the syrinx (Tobimatsu et al. 1991) (Fig. 9.3). Cine imaging can also be helpful in diagnosing spinal CSF flow obstructions in patients with primary spinal syringomyelia (Mauer et al. 2008) (Fig. 9.4).
9.6 MRI Protocols to Investigate Chiari and Syringomyelia
Radiographic evaluation of syringomyelia should include T1-weighted and T2-weighted MRI studies of the brain and entire spine, performed with and without contrast. Brain MRI will detect the presence of unsuspected hydrocephalus , which may contribute to the pathogenesis of some cases of syringomyelia (Krayenbuhl and Benini 1971), a posterior fossa mass producing tonsillar herniation, Chiari I malformation (Fig. 9.5) and basilar invagination . Lumbar spine MRI evaluates for cord tethering by the filum terminale , although the conus medullaris is usually in normal position in patients with Chiari I malformation (Milhorat et al. 2009; Royo-Salvador 1997). Tethering of the cervical or thoracic spinal cord has been associated with syringomyelia (Kitahara et al. 1995), and untethering of the spinal cord has been reported to result in syrinx resolution in these patients (Cusick and Bernardi 1995; Erkan et al. 1999; Levy 1999; Milhorat et al. 2009; Ragnarsson et al. 1986; Royo-Salvador 1997; Takahashi et al. 1999).
On T1-weighted and T2-weighted MRI, syringomyelia appears as a well-circumscribed intramedullary fluid-filled mass. In cases in which the cerebellar tonsils are in a normal position and the syrinx is located within the cervical or cervicothoracic spinal cord, as in Chiari 0 malformation (Fig. 9.6) (Iskandar et al. 1998), FIESTA images should be obtained and examined closely for evidence of membranes occluding the foramen of Magendie or the subarachnoid space at the foramen magnum. Anatomical images should also be evaluated for narrowing of CSF pathways at the foramen magnum and reduced posterior fossa volume (Bogdanov et al. 2004). Phase-contrast cine MRI of the cervical spine in the sagittal plane may be performed, to confirm any narrowing of CSF pathways at the foramen magnum seen on anatomical MRI studies. Axial plane images are able to measure CSF flow as well as velocity but have little clinical value because the length of their view of the subarachnoid space is restricted to the thickness of the axial sections.
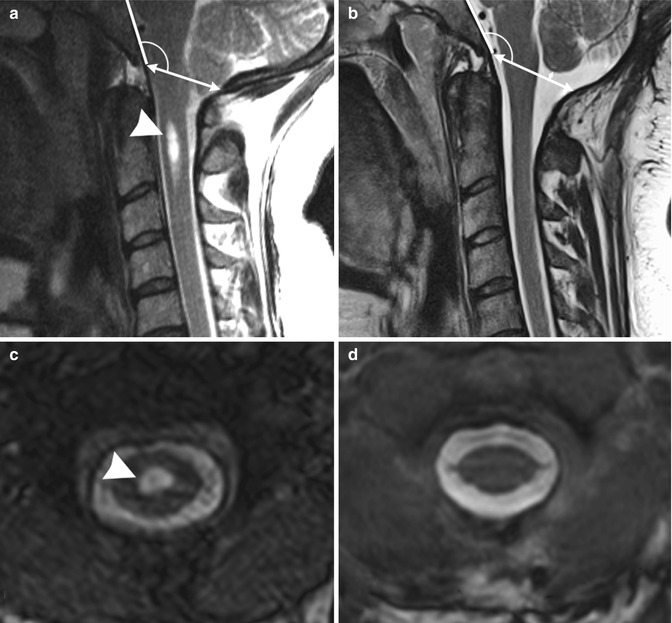
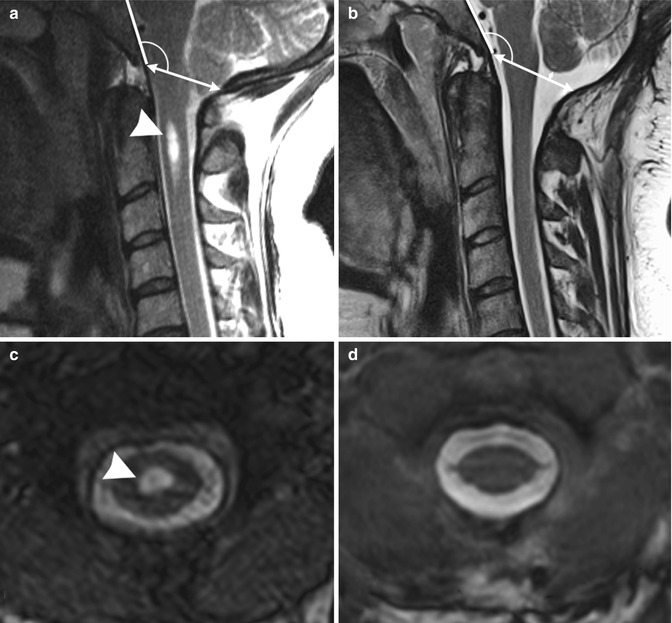
Fig. 9.6
Chiari 0 malformation causing headache. T2-weighted magnetic resonance imaging in a 35-year-old woman with occipital headache. Note Chiari 0 malformation with 3.0 mm of tonsillar ectopia before surgery (a). The CSF pathways at the foramen magnum are obliterated. Tonsillar ectopia resolved on MRI performed 1 year after craniocervical decompression and duraplasty (b), ascending 4 mm above its preoperative position. An associated small syrinx located in the C2 segment before surgery (arrowhead, a, c) resolved after surgery (d)
On T1-weighted MRI, following intravenous administration of gadolinium contrast medium, enhancement within the spinal cord indicates the presence of an associated intramedullary tumour .
Certain findings on the spinal MRI predict more rapid neurological progression in patients with syringomyelia, particularly distension of the spinal cord by a syrinx of large diameter (over 5 mm) and the presence of associated spinal cord oedema (Levy 2000). On the other hand, a narrow syrinx that does not distend the spinal cord may no longer be associated with an active pathophysiological process and is less likely to cause neurological progression. Some patients, with long-standing symptoms of syringomyelia, will have a small-diameter syrinx and an atrophic spinal cord. In such cases, a previously distended syrinx has probably collapsed after a Chiari I malformation has spontaneously disimpacted at the foramen magnum or the syrinx has created a fistula to the spinal subarachnoid space (Santoro et al. 1993; Williams 1994). Prominence of the central canal of the spinal cord that does not distend the spinal cord is a normal anatomical variant, does not produce neurological deficit and does not require treatment (Holly and Batzdorf 2002).
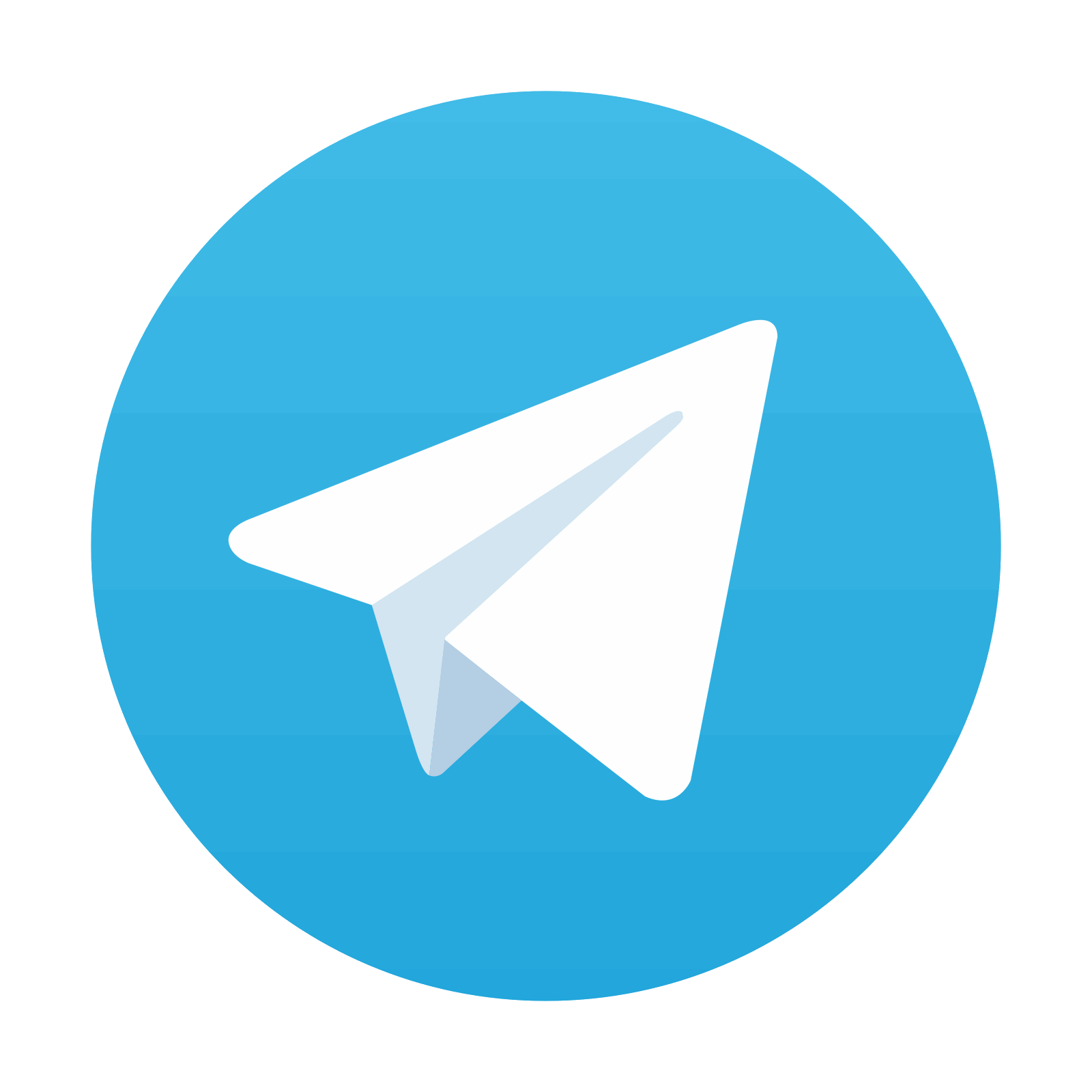
Stay updated, free articles. Join our Telegram channel

Full access? Get Clinical Tree
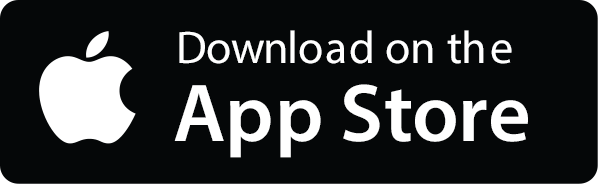
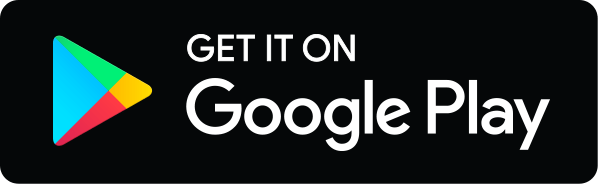