Chapter 23 Drives and Emotions: The Hypothalamus and Limbic System
The limbic system is the name given to the portions of the brain primarily concerned with such responses and behaviors. As discussed in some detail later in this chapter and in the next one, it includes the cingulate and parahippocampal gyri (see Fig. 3-13), the amygdala, and the hippocampus. In view of the preceding discussion, it is not surprising that the components of the limbic system appeared early in vertebrate phylogeny and that, in terms of its connections, the limbic system is interposed between the hypothalamus and the neocortex. That is, limbic structures serve as bridges between autonomic and voluntary responses to changes in the environment. Our response to a chilly room provides a simple example. There are autonomic responses to the chill, coordinated by the hypothalamus, such as cutaneous vasoconstriction and shivering. We also become consciously aware of the chill and may choose to put on more clothes or throw another log on the fire.
The Hypothalamus Coordinates Drive-Related Behaviors
The hypothalamus (Fig. 23-1) is a small portion of the diencephalon (weighing only about 4 g), but it is important as a nodal point in pathways concerned with autonomic, endocrine, emotional, and somatic functions (Fig. 23-2)* that are generally designed to maintain our internal environment in a physiological range (i.e., to promote homeostasis). For example, stimulation of appropriate hypothalamic areas in experimental animals can cause vasodilation, feeding behavior, or alterations of pituitary function. Its functions extend beyond this, however, into more complex interactions involved in drives and emotional behaviors; stimulation of overlapping hypothalamic areas can elicit responses such as rage, sleep, or sexual behavior. Participation in these multiple functions is based on a widespread set of connections that fall into three principal categories: (1) interconnections with various components of the limbic system, (2) outputs that influence the pituitary gland, and (3) interconnections with various visceral and somatic nuclei, both motor and sensory, of the brainstem and spinal cord. The hypothalamus is divided into a number of nuclei and areas, as described shortly. Each of these different nuclei and areas has more or less distinctive connections, but for the sake of simplicity, most of these connections are discussed here as though the hypothalamus were by and large a uniform structure.
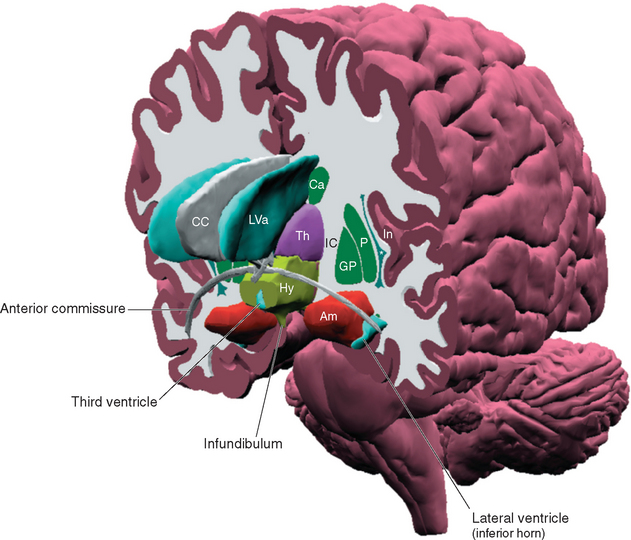
Figure 23-1 Three-dimensional reconstruction of the hypothalamus and surrounding cerebral structures. The hypothalamus has been rendered with a flat anterior surface because the preoptic area (see Fig. 23-4), which envelops the anterior end of the third ventricle but is in front of the plane sometimes used to separate the diencephalon and telencephalon, was not included. *, claustrum; Am, amygdala; Ca, caudate nucleus; CC, corpus callosum; GP, globus pallidus; Hy, hypothalamus; IC, internal capsule; In, insula; LVa, anterior horn of the lateral ventricle; P, putamen; Th, thalamus.
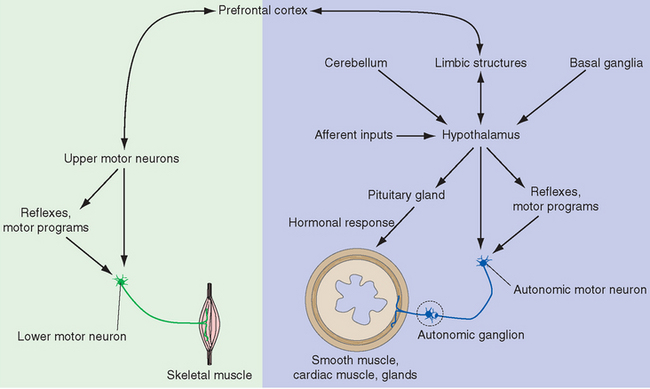
Figure 23-2 Overview of the pivotal role of the hypothalamus in drive-related activities. The hypothalamus can affect autonomic motor neurons both directly and through visceral motor programs in the brainstem and spinal cord, and it can influence visceral structures through its control over the pituitary gland (see Fig. 23-10). It can also stimulate somatic responses through connections with limbic structures that interconnect the hypothalamus and neocortex. The latter are two-way connections, providing us with a degree of voluntary control over responses that may be physiologically desirable but do not fit the current circumstances in some other way (e.g., “grin and bear it”). The cerebellum and basal ganglia also have connections with the hypothalamus, but their roles in the planning and coordination of drive-related activities are still poorly understood and are not discussed in this chapter.
The Hypothalamus Can Be Subdivided in Both Longitudinal and Medial-Lateral Directions
The inferior surface of the hypothalamus (see Figs. 3-16 and 3-17), exposed directly to subarachnoid space, is bounded by the optic chiasm, the optic tracts, and the posterior edge of the mammillary bodies. This area, exclusive of the mammillary bodies, is called the tuber cinereum (Latin for “gray swelling”; Fig. 23-3A). The median eminence protrudes from the surface of the tuber cinereum and is continuous with the infundibular stalk, which in turn is continuous with the posterior lobe of the pituitary. The infundibular stalk and posterior lobe together constitute the neurohypophysis.
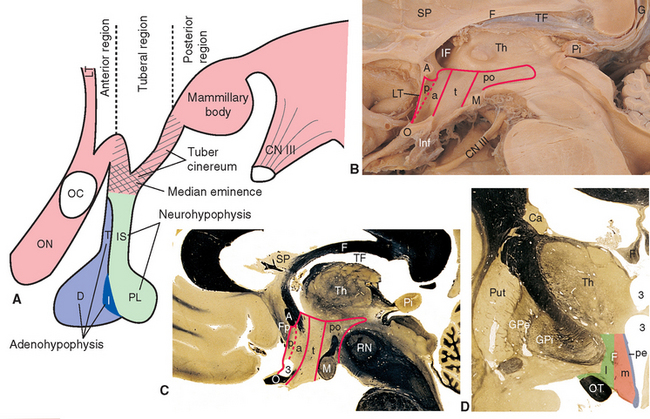
Figure 23-3 A, Regions of the hypothalamus and pituitary in midsagittal view. The entire area filled with diagonal lines is the tuber cinereum. The crosshatched portion of the tuber cinereum is the median eminence. B, The medial surface of the hypothalamus. C, Myelin-stained parasagittal section of the diencephalon, near the midline. D, Myelin-stained coronal section of the diencephalon and basal ganglia, showing the medial-lateral subdivision of the hypothalamus. The dashed red lines in B and C indicate the transverse plane sometimes used as the diencephalon-telencephalon boundary; the area between this plane and the lamina terminalis (the preoptic area) is considered here to be part of the hypothalamus. 3, third ventricle (optic recess in C); A, anterior commissure; a, p, po, and t, anterior, preoptic, posterior, and tuberal regions of the hypothalamus; Ca, caudate nucleus; D, distal part of the adenohypophysis; F, fornix; Fp, precommissural part of the fornix (see Fig. 24-17); G, great cerebral vein (of Galen); GPe, external segment of the globus pallidus; GPi, internal segment of the globus pallidus; I, intermediate part of the adenohypophysis; IF, interventricular foramen; Inf, infundibulum; IS, infundibular stalk; l, m, and pe, lateral, medial, and periventricular regions of the hypothalamus; LT, lamina terminalis; M, mammillary body (part of the posterior hypothalamus); O or OC, optic chiasm; ON, optic nerve; OT, optic tract; Pi, pineal gland; PL, posterior lobe of the pituitary; Put, putamen; RN, red nucleus; SP, septum pellucidum; T, tuberal part of the adenohypophysis; TF, transverse fissure; Th, thalamus.
The medial surface of the hypothalamus (Fig. 23-3B) extends anteriorly to the lamina terminalis, superiorly to the hypothalamic sulcus, and posteriorly to the caudal edge of the diencephalon. As in the case of the brainstem, transverse planes are sometimes used to define the anterior and posterior boundaries—in this case, a plane passing through the posterior commissure and the posterior edge of the mammillary bodies, and another passing through the anterior edge of the optic chiasm and the posterior edge of the anterior commissure. Also as in the case of the brainstem, however, functionally related neural tissue continues through both these planes. For example, the neural tissue immediately in front of the anterior plane is structurally and functionally continuous with the hypothalamus (Fig. 23-3B and C). Therefore this region (the preoptic area) is treated instead as part of the anterior hypothalamus.
The hypothalamus can be subdivided from front to back into anterior, tuberal, and posterior regions. The anterior region is the part above the optic chiasm, the tuberal region is the part above and including the tuber cinereum, and the posterior region is the part above and including the mammillary bodies. In addition, the entire hypothalamus of each side is divided into three longitudinal zones (Fig. 23-3D). The thin periventricular zone, in the wall of the third ventricle, is a rostral continuation of the periaqueductal gray. The lateral zone, lateral to the fornix as this fiber bundle traverses the hypothalamus, is a rostral continuation of the reticular formation. The periventricular and lateral zones include collections of neurons and are also avenues through which ascending and descending axons enter, leave, or traverse the hypothalamus; the medial zone between them is populated by a series of hypothalamic nuclei (Fig. 23-4; Table 23-1).
The periventricular zone is traversed by the dorsal longitudinal fasciculus, a bundle of hypothalamic afferents and efferents (see Fig. 23-6B and C). It also contains a small suprachiasmatic nucleus and a larger arcuate nucleus. The suprachiasmatic nucleus is tiny—less than 1 mm3 and fewer than 10,000 neurons on each side—but it is the “master clock” for our circadian rhythms (Fig. 23-5A). The free-running period of cells in the suprachiasmatic nucleus is typically about 25 hours (Fig. 23-5B), but it receives direct projections from the retina which entrain it to the actual day length. Its neurons also contain numerous melatonin receptors, and the nighttime rise in pineal melatonin secretion is thought to provide an additional signal that helps “set” the circadian clock. The arcuate nucleus, as described later in this chapter, is critically involved in feeding behavior.
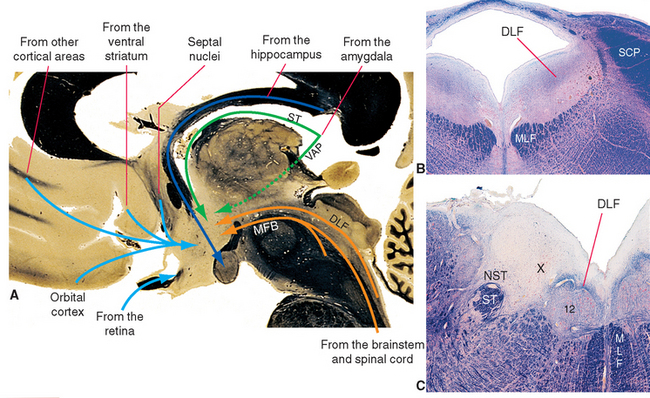
Figure 23-6 A, Major inputs to the hypothalamus. DLF, dorsal longitudinal fasciculus; MFB, medial forebrain bundle; ST, stria terminalis; VAP, ventral amygdalofugal pathway (see Fig. 23-20). B and C, Location of the dorsal longitudinal fasciculus (DLF) in the rostral pons and rostral medulla. 12, hypoglossal nucleus; MLF, medial longitudinal fasciculus; NST, nucleus of the solitary tract; SCP, superior cerebellar peduncle; ST, solitary tract; X, dorsal motor nucleus of the vagus.
The lateral zone consists mainly of scattered cells interspersed among the longitudinally running fibers of the medial forebrain bundle. Anteriorly, it is continuous with the lateral preoptic nucleus, an important sleep-promoting area (see Fig. 22-34); caudally, it is continuous with the midbrain reticular formation. Part of the supraoptic nucleus intrudes into it, as do clumps of cells called lateral tuberal nuclei. It also contains the small tuberomammillary nucleus, the source of histaminergic fibers that project widely to the cerebral cortex and thalamus and participate in the sleep-wake cycle (see Fig. 22-31). Otherwise it is undivided.
The medial zone contains a number of nuclei (Table 23-1). Anteriorly, these include two distinctive nuclei containing large neurosecretory cells: the supraoptic and paraventricular nuclei. The supraoptic nucleus sits astride the optic tract, extending into the lateral hypothalamic zone; the paraventricular nucleus is higher up in the wall of the third ventricle, near the anterior commissure. Most cells of the supraoptic nucleus and many cells of the paraventricular nucleus secrete hormones that travel down the axons of these cells and are released in the neurohypophysis. The hormones involved and the pathway traversed by them are discussed later in this chapter (see Fig. 23-10). The medial tuberal region is sub-divided into dorsal and ventral portions called the dorsomedial and ventromedial nuclei, respectively. In addition, clusters of orexin-containing neurons near the fornix, extending into the lateral and posterior hypothalamus, are the source of a second set of widespread wakefulness-promoting projections (see Fig. 22-31). The medial mammillary region contains the mammillary body (actually a complex of several nuclei) and the posterior hypothalamic nucleus, part of which is continuous with the periaqueductal gray matter of the midbrain.
Hypothalamic Inputs Arise in Widespread Neural Sites
Neural inputs to the hypothalamus arise in two general areas (Fig. 23-6): (1) various parts of the forebrain, particularly components of the limbic system, and (2) the brainstem and spinal cord. Afferents from the brainstem and spinal cord convey visceral and somatic sensory information, whereas those from limbic structures convey information relevant to the role of the hypothalamus in mediating many of the autonomic and somatic aspects of affective states. The connections of limbic components with one another and with the hypothalamus are discussed later in this chapter and are mentioned only briefly here.
Most Inputs from the Forebrain Arise in Limbic Structures
The septal nuclei (see Fig. 25-2), prominent components of the limbic system located adjacent to the septum pellucidum, project fibers to the hypothalamus through the medial forebrain bundle. The medial forebrain bundle is built like a frayed rope, with fibers entering and leaving it at many levels as it traverses the lateral hypothalamic zone and extends into the brainstem tegmentum. This is a bidirectional bundle that also contains afferents from the brainstem to the hypothalamus, hypothalamic efferents passing both rostrally and caudally, fibers interconnecting different hypothalamic levels, and fibers passing through the hypothalamus on their way to someplace else.
The major output from the hippocampus is contained in the fornix. This fiber bundle arches around under the corpus callosum and through the hypothalamus, where many of its fibers reach the mammillary body (see Fig. 24-17).
The amygdala projects fibers to the hypothalamus by two different routes. Some travel through the stria terminalis, a long, curved fiber bundle that accompanies the caudate nucleus. Others take a shorter course and pass under the lenticular nucleus directly to the hypothalamus (see Fig. 23-20).
Inputs from the Brainstem and Spinal Cord Traverse the Medial Forebrain Bundle and Dorsal Longitudinal Fasciculus
An assortment of sensory inputs (in addition to those from the retina) reaches the hypothalamus by several routes. Some involve synapses in various portions of the reticular formation and periaqueductal gray; others arrive directly from sites such as the solitary and parabrachial nuclei. Some of these afferents travel in the medial forebrain bundle; others are contained in the dorsal longitudinal fasciculus (Fig. 23-6B and C), a collection of thinly myelinated fibers that pass through the periventricular and periaqueductal gray of the brainstem and then fan out in the hypothalamic wall of the third ventricle. Still other afferents enter the hypothalamus as collaterals of fibers in other pathways such as the spinothalamic tract.
Ascending axons from brainstem monoaminecontaining neuronal groups—locus ceruleus, raphe nuclei, and the ventral tegmental area—also traverse the medial forebrain bundle on their way to innervate the cerebral cortex (see Figs. 11-24, 11-26, and 11-27). Along the way, some terminate in the hypothalamus.
The Hypothalamus Contains Intrinsic Sensory Neurons
In addition to receiving various types of visceral and somatic information through the brainstem pathways just mentioned, the hypothalamus contains neurons that are directly responsive to physical stimuli. Some of these cells are sensitive to the temperature of the hypothalamus itself, whereas the activity of others is sensitive to such things as blood osmolality (Fig. 23-7) or the concentration of glucose or certain hormones in blood passing through the hypothalamus.
Hypothalamic Outputs Largely Reciprocate Inputs
Some efferent pathways from the hypothalamus reciprocate the afferent pathways (Fig. 23-8). Thus the hypothalamus projects to the septal nuclei, hippocampus, amygdala, and the brainstem and spinal cord by way of the same fiber bundles that carry afferents to the hypothalamus. Efferents to the cerebral cortex, rather than focusing on limbic areas, blanket the cortex with widespread projections in a manner similar to that of the monoamine-containing fibers from the brainstem; the two most prominent examples are histaminergic fibers from the tuberomammillary nucleus and orexinergic fibers from the tuberal and posterior hypothalamus. A few pathways are totally or predominantly efferent in nature. The prominent mammillothalamic tract, for example, passes from the mammillary body to the anterior nucleus of the thalamus. Shortly after leaving the mammillary body, many of these same axons send branches to the midbrain reticular formation through the mammillotegmental tract.
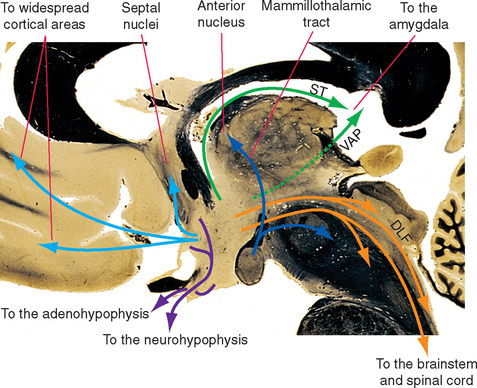
Figure 23-8 Major outputs from the hypothalamus. DLF, dorsal longitudinal fasciculus; ST, stria terminalis; VAP, ventral amygdalofugal pathway (see Fig. 23-20).
The Hypothalamus Controls Both Lobes of the Pituitary Gland
The final efferent pathways from the hypothalamus are of great functional importance because they control the pituitary gland (or hypophysis). The pituitary is a two-part gland (Fig. 23-9), with a posterior lobe that develops as a downward outgrowth from the diencephalon and an anterior lobe that develops from the roof of the mouth; the anterior lobe, together with its upward extension around the infundibular stalk, constitutes the adenohypophysis. The hypothalamus has a separate control system for each lobe: a neural projection to the neurohypophysis and a vascular link with the adenohypophysis.
The supraoptic and paraventricular nuclei, as mentioned previously, contain large neurosecretory cells. (The paraventricular nucleus also contains numerous smaller cells, many of which project as hypothalamic efferents to the dorsal motor nucleus of the vagus nerve, the intermediolateral cell column of the spinal cord, and other sites.) The large neurosecretory cells of the supraoptic and paraventricular nuclei produce two peptide hormones, each nine amino acids in length; a given cell produces only one of the two hormones. The first is antidiuretic hormone (ADH, or vasopressin), whose principal physiological function as a hormone* is to increase the reabsorption of water in the kidney and thereby decrease the production of urine. The second is oxytocin (from Greek words meaning “rapid birth”), a similar peptide that causes contraction of uterine and mammary smooth muscle and is important in parturition and milk ejection. Both hormones travel down the axons of their parent cell bodies by axoplasmic flow, bound to a carrier protein (neurophysin). These neurosecretory cells are electrically excitable, and the passage of action potentials down their axons causes release of their hormones from bulbous endings adjacent to capillaries in the median eminence, infundibular stalk, and posterior lobe of the pituitary (Fig. 23-10A). Most of the axons arise in the supraoptic nucleus, so this pathway is called the supraopticohypophyseal tract.
The adenohypophysis secretes a multitude of hormones whose discussion is beyond the scope of this book. It has long been known that electrical stimulation of certain areas of the hypothalamus can modulate the rates of secretion of these hormones, but there are no neural connections that can explain this modulation. This drew attention to the hypophyseal portal system as a vascular connection between the hypothalamus and the adenohypophysis (Fig. 23-10B). The superior hypophyseal artery, a branch of the internal carotid, breaks up into a capillary bed in the median eminence and proximal part of the infundibular stalk. Blood in these capillaries then re-collects into hypophyseal portal vessels, which travel down the infundibular stalk and break up into a second capillary bed in the adenohypophysis. Small peptides,* called hypothalamic releasing hormones and inhibiting hormones, are secreted by cells of the arcuate nucleus and nearby sites in the wall of the third ventricle,† travel down the axons of these cells, and are released into the bloodstream in the first capillary bed. (The median eminence is one of the circumventricular organs [see Fig. 6-29], and its capillaries are fenestrated [see Fig. 6-30].) From there the releasing and inhibiting hormones travel down the hypophyseal portal vessels to the adenohypophysis, where they act. As their names imply, releasing hormones promote the release of particular hormones, whereas inhibiting hormones prevent this release. The entire collection of axons carrying these releasing and inhibiting factors is called the tuberoinfundibular or tuberohypophyseal tract.
The same small hypothalamic peptides are also found in other neurons in widespread areas of the central nervous system (CNS) and in other cells of the body as well. This is reminiscent of the situation with enkephalins and endorphins, briefly discussed in Chapter 11. A slowly emerging picture is that, in some respects, the brain is a much more “distributed” organ than it is normally considered to be—that is, it may use the same chemical both as a neurotransmitter acting locally on a postsynaptic neuron and as a hormone acting at a distance, both actions working toward the same physiological goal.
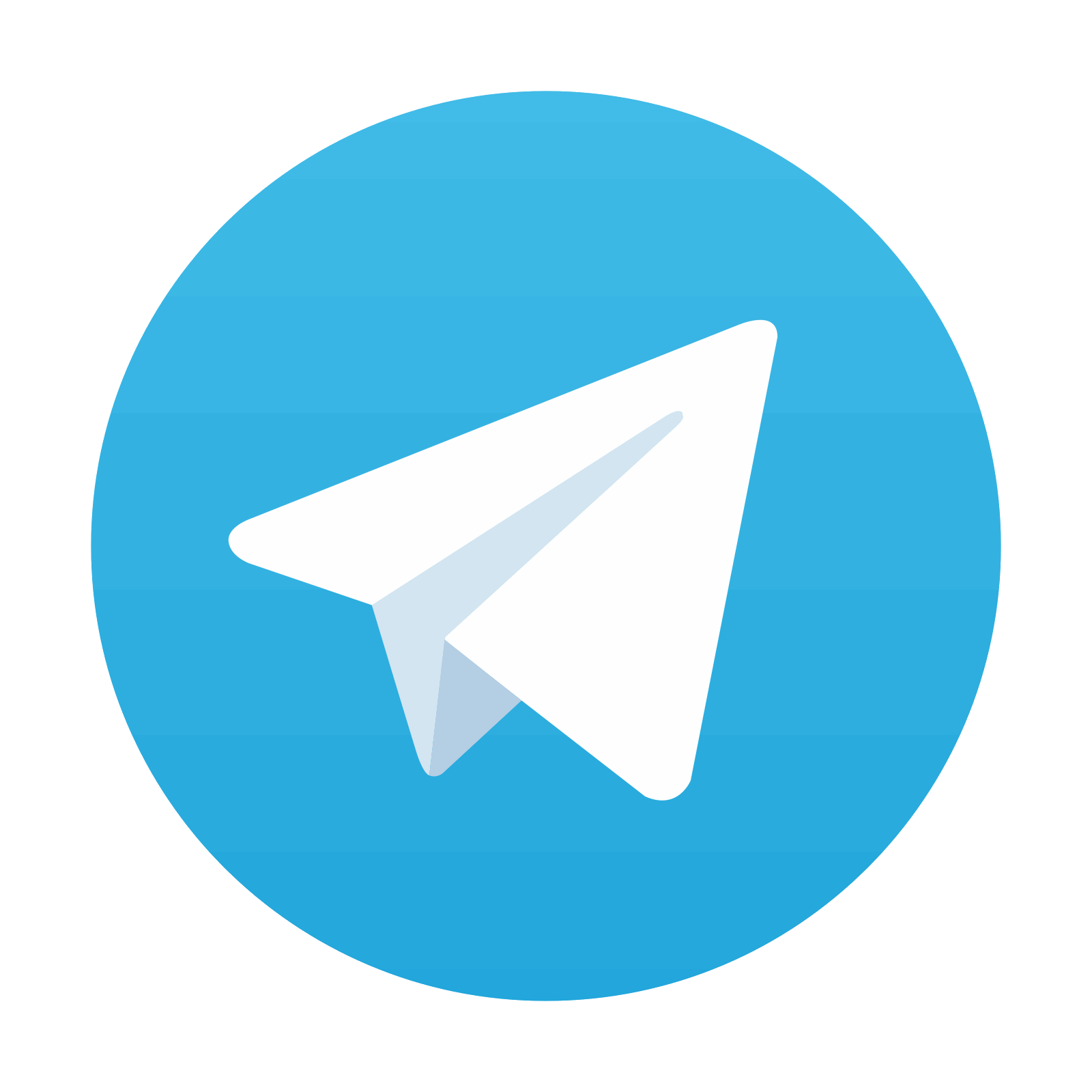
Stay updated, free articles. Join our Telegram channel

Full access? Get Clinical Tree
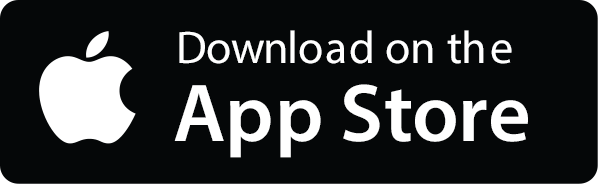
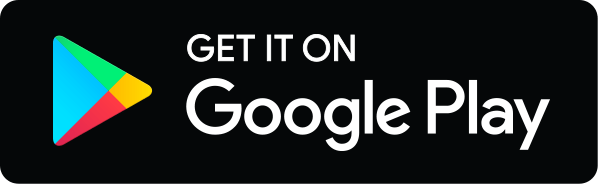