Introduction
The neuroendocrine system is important for body homeostasis but can undergo significant changes in response to critical illness. During the acute phase of critical illness or trauma, anabolic hormonal pathways are down-regulated in favor of fulfilling more immediate needs. With prolonged critical illness, the hypothalamic-pituitary axis (HPA) is subject to reduced pulsatile secretion of pituitary hormones, reduced peripheral activity, and global hormonal suppression. If unrecognized, HPA dysfunction can lead to problems in both the short and long term. However, monitoring the endocrine status of critically ill patients can be a challenge. First, pituitary dysfunction often can mimic deficits associated with the neurologically injured patient ( Table 21.1 ). In addition, critical illness may induce endocrine dysfunction that is not observed outside the intensive care unit (ICU). Therefore a high index of suspicion or a screening protocol is necessary. Second, many of the signs and symptoms of endocrine dysfunction are subtle, may be delayed in onset, or may be masked by interventions common in the acute setting. Third, assays used in healthy patients may underestimate hormone levels in the acutely ill, and standards used to define appropriate normal values may not be applicable. Although there are few widely adopted management guidelines, protocol-driven screening of the at-risk patient may avoid the potential for misdiagnosis and so influence outcome. There are, however, two important questions in management: (1) does endocrine intervention improve outcome when there is critical illness–induced endocrine dysfunction, and (2) does endocrine intervention help critically ill patients without endocrine dysfunction (e.g., steroid use) to improve hemodynamics?
Hormone Deficiency | Symptoms | Signs |
---|---|---|
Adrenocorticotropic hormone (ACTH) | Weakness, nausea, fever, shock, abdominal pain, headache, adrenal crisis | Hypotension, hypoglycemia, hyponatremia, myopathy, anemia, eosinophilia |
Thyroid hormone | Anergy, neuropsychiatric symptoms, cold intolerance, memory problems, weight gain | Bradycardia, hypotension, neuropathy, myopathy |
Growth hormone | Anergy, poor quality of life | Osteoporosis, reduced lean body mass, dyslipidemia |
Follicle-stimulating and luteinizing hormone | Oligomenorrhea or amenorrhea, sexual dysfunction, mood disorders | Reduced muscle mass and exercise tolerance, osteoporosis, infertility, loss of secondary hair |
This chapter reviews endocrine deficiencies frequently encountered in patients in the neurocritical care unit (NCCU) and discusses the endocrine consequences of traumatic brain injury (TBI) and subarachnoid hemorrhage (SAH). This is important because nearly all endocrine axes have close links with the central nervous system including regulation of pituitary hormone production and direct innervation of endocrine glands. Glycemic control and nutrition are addressed in Chapter 14 .
Practicalities of Endocrine Assessment in the Critical Care Unit
Several practical difficulties are associated with endocrine assessment in the critical care unit. First, patients in the NCCU, particularly those with severe neurologic disorders, sepsis, or multiorgan dysfunction, are likely to have physiologic changes rarely, if ever, seen in patients in an outpatient setting. Second, it is unclear whether standard tests of endocrine function that primarily are designed to assess patients in the outpatient setting (i.e., not under “stress”) are valid in critical care. For example, basal levels of hormones and regulation of hormone responsiveness (i.e., feedback regulation) cannot be assumed to be the same in the typical patient undergoing outpatient evaluation and one in the ICU. Third, although hormone levels in the circulation depend in part on synthesis, they also are influenced by levels of binding proteins and tissue-specific metabolism, both of which can be altered by critical illness. Fourth, it is unclear whether adequate serum levels equate with tissue levels. For example, how some hormone receptors respond to their ligands can be altered during sepsis and hypoxia, and hormone metabolism, in particular that of thyroid and cortisol, is altered at the tissue level in critically ill patients. Fifth, endocrine assessment depends on measurement of basal hormone levels and often dynamic tests (i.e., stimulation or inhibition of hormone synthesis). These various tests normally are performed under standard conditions such as fasting without the interference of other medications, and at a particular time. These conditions may be difficult to replicate in the ICU. Furthermore, several dynamic tests (e.g., insulin tolerance test or metyrapone test) used in the outpatient setting may be dangerous to use in the critical care patient. Sixth, there can be a delay in turnaround of hormone assay results, and hence empiric treatment may be needed. Seventh, any critical illness is a dynamic process, and physiologic responses vary and evolve over time. Consistent with this, cortisol levels are well known to vary in the same patient during a 24-hour period. This implies that frequent samples may be needed but with that comes an increased risk of false-positive results. Finally, various medications and therapies given to patients in the ICU may alter the accuracy of laboratory testing of endocrine function ( Table 21.2 ). In addition, results may differ across laboratories because standardization of assays may vary. Together these limitations mean that the clinician in the NCCU must pay careful attention to the whole patient rather than simply interpreting individual hormone levels.
Adrenal Dysfunction | Thyroid Dysfunction |
---|---|
Heparins | Heparins |
Warfarin and other anticoagulants | Iodinated contrast dye |
Phenobarbital, phenytoin | Phenobarbital, phenytoin, carbamazepine |
Azole antifungals | Amiodarone |
Etomidate | Corticosteroids |
Glucocorticoid therapy | Dobutamine, dopamine |
Opiates and benzodiazepines | Furosemide |
Statins | Octreotide |
Rifampin | Lithium, atypical antipsychoticsRifampin |
The challenges facing laboratory staff, including preanalytic, analytic, postanalytic and those associated with assay methodology are reviewed elsewhere. A key issue, despite considerable effort, is that units used for reporting assay results and assay standardizations vary around the world. Furthermore, there can be variation in assay performance based on the manufacturer. Consequently there are international scientific efforts to standardize assays in which assays are compared against a candidate reference measurement procedure. This means that a given substance, for example, free cortisol, measured in a given system such as serum is collected under defined conditions, and the units used to report the levels are traceable to a reference measurement procedure and a reference preparation that are certified by such organizations as the Joint Committee for Traceability in Laboratory Medicine.
Anterior Pituitary Dysfunction
Anatomy
The pituitary gland is divided into two functionally, physiologically, and developmentally distinct units: the anterior pituitary, or adenohypophysis, and the posterior pituitary, or neurohypophysis. The anterior pituitary develops from ectodermal cells that overlie the pharynx that invaginate to form Rathke’s pouch. The posterior wall of Rathke’s pouch also forms a small intermediate lobe that secretes melanocyte-stimulating hormone in the fetus, but this regresses in the adult. The pituitary gland derives its blood supply from two branches of the internal carotid artery: the inferior and superior hypophyseal arteries. The anterior pituitary contains glandular cells that respond to inhibitory and releasing factors secreted by the arcuate, periventricular, medial preoptic, and paraventricular nuclei from the hypothalamus. The axons of the neurons located within these nuclei project to the median eminence, where they release secretory and inhibitory factors into a capillary plexus. This median eminence capillary plexus then drains into a second capillary plexus within the anterior pituitary via the hypophyseal portal veins. The hypothalamic factors then stimulate or inhibit the glandular cells of the anterior pituitary and modify secretion of hormones into the secondary capillary plexus. Ultimately, the hypophyseal portal vein drains to the cavernous sinus and to the systemic circulation through the internal jugular vein.
Corticotroph Dysfunction
Etiology
Decreased or inappropriate cortisol secretion is the hallmark of adrenal insufficiency. Adrenal insufficiency may be due to processes that affect: (1) the adrenal gland itself (primary adrenal insufficiency), (2) the pituitary gland and secretion of adrenocorticotropic hormone (ACTH) (secondary adrenal insufficiency), or (3) the hypothalamus and its secretion of corticotropin-releasing hormone (tertiary adrenal insufficiency).
Acute primary adrenal insufficiency may result from bilateral adrenal hemorrhage or infarction secondary to trauma, coagulopathy, thromboembolic disease, or sepsis (e.g., Waterhouse-Friderichsen syndrome). Waterhouse-Friderichsen syndrome was first described in the setting of meningococcemia, but also has been reported in several other systemic bacterial infections including those caused by Pseudomonas aeruginosa, Streptococcus pneumoniae, Neisseria gonorrhoeae, Escherichia coli, Haemophilus influenzae, and Staphylococcus aureus. Several drugs can cause adrenal insufficiency by inhibiting cortisol biosynthesis (e.g., etomidate, ketoconazole, metyrapone, suramin) or by accelerating its metabolism (e.g., phenytoin, barbiturates, rifampin).
Any disease process or medication that interferes with ACTH secretion can cause secondary adrenal insufficiency. Infection, TBI, tumors, infiltrative diseases, hemorrhage, and infarction each may damage the pituitary gland. Glucocorticoid use is the most common cause of drug-induced secondary adrenal insufficiency. Exogenous steroids suppress the HPA that leads to atrophy of ACTH-producing cells. Secondary insufficiency can occur when glucocorticoid therapy is tapered or when physiologic stress exceeds the maintenance dose. Consequently, patients who have been on chronic steroids require a “stress-dose” perioperatively or when admitted to the NCCU ( Table 21.3 ).
Degree of Surgical Stress | Dose * | Duration |
---|---|---|
Minor (e.g., inguinal herniorrhaphy) | 25 mg hydrocortisone daily | 1 day |
Moderate (total knee replacement, abdominal hysterectomy, open cholecystectomy) | 50-75 mg hydrocortisone daily | 1 to 2 days |
Major (pancreaticoduodenectomy, cardiac surgery with bypass) | 100-150 mg hydrocortisone daily | 2 to 3 days |
* Hydrocortisone supplementation should be administered in divided daily doses.
Any process that inhibits the hypothalamic production of corticotropin-releasing hormone (CRH), such as tumors, infiltrative diseases, e.g., sarcoidosis, cranial irradiation, and chronic high-dose glucocorticoid therapy will result in tertiary adrenal insufficiency. Without the ACTH-secretagogue action of CRH on the anterior pituitary, ACTH levels decline, endogenous cortisol levels fall, and the adrenal glands atrophy.
Dysfunction of the HPA in the critically ill patient in the absence of glucocorticoid treatment or structural damage to the adrenal glands, pituitary, or hypothalamus is an increasingly recognized phenomenon. The condition has been described as relative adrenal insufficiency (RAI), or critical illness-related corticosteroid insufficiency (CIRCI). These disorders are common in sepsis and are the basis of trials of glucocorticoids in septic shock. The diagnosis is based on plasma cortisol profiles, but there are currently no tests that clearly identify which patient is truly glucocorticoid “deficient” at a cellular level. A consensus statement defined CIRCI as inadequate cellular corticosteroid activity for the severity of the patient’s illness. The pathophysiology of this form of adrenal “insufficiency” is not well defined, but critical illness may alter the HPA and down-regulate its feedback responses resulting in impaired cortisol production and tissue resistance to glucocorticoids. Although the stress of critical illness is associated with high circulating cortisol levels, there often is a blunted response to ACTH. For example, supplemental hydrocortisone may improve hemodynamic stability in septic shock, suggesting a relative shortage of steroids or a down-regulation of available receptors.
Clinical Presentation
Symptoms of acute adrenal insufficiency are often nonspecific and include vomiting, lethargy, abdominal pain, fever, and mental status changes. Severe hypotension and shock then may develop. This presentation can be confused with, or exacerbate preexisting, septic shock. In some patients, the degree of cardiovascular collapse is associated with mineralocorticoid rather than glucocorticoid insufficiency—patients who receive glucocorticoids may still develop an adrenal crisis if their mineralocorticoid requirements are not met. Outcome can be poor if glucocorticoid and mineralocorticoid insufficiency is not diagnosed and treated.
Monitoring the Hypothalamic-Pituitary-Adrenal Axis in the ICU
Considerable controversy surrounds the utility, optimal method, and interpretation of hypothalamic-pituitary-adrenal axis testing in the critically ill patient. In the healthy, non-ICU patient the initial diagnostic tests for adrenal insufficiency include morning plasma ACTH and serum cortisol levels. In general, primary adrenal insufficiency can be distinguished from secondary disease by an elevation in plasma ACTH levels. A cortisol level less than 100 nmol/L (or 3.6 µg/dL) indicates adrenal insufficiency, whereas a value greater than 500 nmol/L (or 18 µg/dL) suggests an intact hypothalamic-pituitary-adrenal axis. However, in the ICU patient a normal or even elevated cortisol does not mean there will be an appropriate response to the stress of surgery or critical illness. The appropriate cortisol level for critically ill patients is unknown, and tests used to diagnose adrenocortical dysfunction have not been validated in the ICU population. Consensus guidelines suggest a random cortisol of less than 275 nmol/L (10 µg/dL) or a change in cortisol levels of less than 250 nmol/L (9 µg/dL) following 250 µg of cosyntropin are the best tests available to diagnose adrenal insufficiency in the critically ill patient.
Several factors make diagnosis in critical care more challenging. First, cortisol immunoassays measure protein-bound cortisol. Both cortisol-binding globulin and albumin are negative acute-phase reactants and their levels decrease in the setting of stress. This makes the relationship between total and free cortisol unpredictable and may give the appearance of adrenal insufficiency, even though free cortisol levels may be appropriate. Second, saturation of the corticotropin receptor, with a corresponding limit of cortisol release, may confound dynamic measurements of glucocorticoid function. Consequently a low, unstimulated free plasma cortisol level has been proposed as a better marker of adrenal insufficiency. Although this makes free cortisol an attractive target for testing, the assay is not routinely available. Furthermore, total cortisol has been shown to correlate with free cortisol in treatment-resistant hypotension in critical illness.
Hyponatremia and hyperkalemia develop in acute adrenal insufficiency, so assessment of electrolytes can be a useful adjunct in the patient who develops hypotension or other symptoms of acute adrenal insufficiency.
Adrenal Insufficiency Management
An acute adrenal crisis can rapidly progress to death; therefore, suspected cases should be immediately treated with 100 mg of intravenous (IV) hydrocortisone followed by 100 to 200 mg per day. Because there is relatively little risk associated with short-term steroid use, treatment of acute adrenal insufficiency should not be delayed pending diagnostic evaluation. Hydrocortisone provides both glucocorticoid and mineralocorticoid supplementation without sustained suppression of the HPA and is the preferred agent to provide “stress dose” steroids. Fluid resuscitation with isotonic fluid and vasopressors also are often required. Electrolyte abnormalities and hypoglycemia should be corrected.
Treatment of CIRCI in severe sepsis may be beneficial but remains controversial. In patients with severe septic shock, Annane et al. demonstrated corticosteroid replacement reduced the duration of vasopressor support and improved survival in patients with RAI. However, in another randomized clinical trial, corticosteroid therapy of septic shock (CORTICUS), steroid use was associated with improved hemodynamic stability but had no effect on overall survival. In addition, the cosyntropin stimulation test could not help differentiate who would benefit from steroid supplementation. Several factors including differences in patient acuity, timing of enrollment, and the addition of fludrocortisone may explain the different outcome results in these two trials. The 2008 Surviving Sepsis Campaign guidelines do not recommend using a cosyntropin stimulation test to diagnose relative adrenal insufficiency but suggest hydrocortisone (<300 mg/day) may be used in any patient with septic shock that is refractory to fluid and vasopressor support. Similarly, the consensus statements from an international task force by the American College of Critical Care Medicine recommend treatment of critically ill patients based on clinical criteria rather than the results of adrenal function testing.
Because hydrocortisone has mineralocorticoid activity, the benefit of adding fludrocortisone as described by Annane et al. remains unclear. Nonetheless, oral fludrocortisone (50 µg daily) is considered an optional therapy in the Surviving Sepsis Campaign guidelines. Supplemental steroids should be tapered when vasopressors are no longer required to minimize side effects including the risk of secondary adrenal suppression.
Thyrotroph Dysfunction
Physiology
Thyroid hormone secretion is tightly regulated by the hypothalamic-pituitary-thyroid axis. Thyrotropin-releasing hormone (TRH) is released from the hypothalamus and stimulates the synthesis and secretion of thyroid-stimulating hormone (TSH). TSH, in turn, controls the synthesis and secretion of the thyroid hormones, thyroxine (T4) and triiodothyronine (T3). More than 99.5% of T4 and T3 are protein bound in the serum and are metabolically inactive. The small percentage of free T4 and T3 influence metabolic function and modulate the release of both TRH and TSH using a negative feedback system. The thyroid gland primarily produces T4, a biologically inactive hormone that must be converted to the active hormone T3 in peripheral tissues. Because thyroid hormone is critical for cellular metabolism, both overproduction and undersecretion can affect all organ systems.
Hyperthyroidism and Thyroid Storm
Excess T 4 levels characterize hyperthyroidism. An overproductive thyroid nodule or gland is the most common etiology, and TSH production is suppressed. Less commonly, excess TSH causes elevated thyroid hormone levels. Thyrotoxic crisis, or thyroid storm, is a rare but potentially life-threatening state that most frequently occurs in patients with untreated or partially treated hyperthyroidism. Trauma or an acute illness can precipitate this transition from stable hyperthyroidism to a decompensated crisis. Thyroid function tests are not helpful to distinguish the two states. Instead the diagnosis of thyroid storm is made on clinical grounds. Signs and symptoms of thyroid storm are exaggerated features of hyperthyroidism and include fever (>38.5° C), tachycardia, hypertension, agitation, delirium, lethargy, seizures, coma, nausea, vomiting, diarrhea, and, in severe cases, jaundice.
Treating Thyrotoxicosis
The therapeutic goals of treating thyroid storm are to: (1) decrease hormone production and secretion, (2) block the conversion of T4 to T3, and (3) antagonize the catecholaminergic effects of thyroid hormone. Thionamides such as propylthiouracil and methimazole can block new thyroid hormone synthesis within hours of administration. Propylthiouracil is preferred because it also inhibits the peripheral conversion of T4 to T3. These agents, however, do not prevent the release of stored hormone. High-dose iodine (e.g., Lugol’s solution or saturated solution of potassium iodide) can acutely block the release of T4 and T3, but should be given only after thyroid synthesis has been blocked. If synthetic function is not adequately suppressed, the iodine bolus will enhance thyroid hormone synthesis and thus exacerbate the thyrotoxicosis. Glucocorticoids can reduce the peripheral conversion of T4 to T3 and, given a high risk of concomitant adrenal insufficiency, are considered standard of care in severe thyrotoxicosis. Beta-adrenergic blockade, in particular propranolol that has antiadrenergic effects and inhibits the peripheral conversion of T4 to T3, remains a mainstay of therapy. Alternatively, esmolol can be used when rapid titration is needed to reduce potential side effects. Clinical improvement usually is rapid following the initiation of β-blocker therapy. In addition to the expected cardiac effects, there is marked improvement in agitation, confusion, psychosis, diaphoresis, diarrhea, and fever.
Myxedema Coma
Etiology and Presentation
Myxedema coma is the result of severe, decompensated hypothyroidism that can occur insidiously as the result of severe long-standing hypothyroidism or it can be precipitated by an acute event such as infection, myocardial infarction, head injury, cold exposure, or certain medications (e.g., opiates, lithium, amiodarone). Patients with myxedema coma demonstrate the classic features of severe hypothyroidism including dry skin, thin hair, periorbital swelling, nonpitting edema of the hands and feet, a hoarse voice, macroglossia, and delayed deep tendon reflexes. Progression to myxedema coma, however, is characterized by a depressed mental status, hypotension, and hypothermia. Focal and generalized seizures also have been reported. Depressed myocardial contractility and bradycardia result in low cardiac output, profound hypotension, and diminished cerebral perfusion. Mortality can be high (30%-60%) even with early diagnosis and appropriate therapy and is associated with the degree of hypothermia. A core temperature less than 32° C is associated with a worse prognosis.
Laboratory Findings in Myxedema Coma
Hyponatremia and hypoglycemia are common. Low serum sodium results from excessive vasopressin secretion and impaired free water excretion. Significant hyponatremia (≤125 mEq/L) is common and may contribute to mental status changes. Decreased gluconeogenesis and hypoglycemia may occur with hypothyroidism alone or with adrenal insufficiency. Infection is frequently a precipitating cause of myxedema coma. However, an elevated white blood cell (WBC) count often is absent, although a left shift may be observed.
Management of Myxedema Coma
When clinically suspected, therapy should be instituted without waiting for laboratory confirmation. However, thyroid function tests (serum TSH and free T4) should be drawn before starting therapy. A cortisol level also should be obtained to investigate the possibility of concurrent adrenal insufficiency. Appropriate hormonal supplementation normalizes the basal metabolic rate and reverses all symptoms and signs of hypothyroidism. Hydrocortisone should be given with thyroid replacement for several days and then tapered based on clinical improvement and assessment of adrenal function because thyroid replacement may unmask coexisting adrenal insufficiency and precipitate an adrenal crisis.
The deiodinase conversion of T4 to T3 is impaired in severe hypothyroidism and so IV T3 may be preferable given its greater biologic availability. T3 rapidly achieves effective tissue levels and crosses the blood-brain barrier more readily than T4 and can hasten the improvement of neurologic symptoms. The rapid onset of T3, however, also may increase the risk of treatment-associated complications such as myocardial infarction or arrhythmias. Treatment with IV T4 is associated with a slower onset of action because the patient’s inherent tissue deiodinase activity is required to convert T4 to T3 and clinical improvement may take 1 to 3 days. However, the slower onset of action may decrease the likelihood of cardiac complications. A third treatment option is to supplement both T4 and T3. This provides T3 at a subtherapeutic dose for immediate action and a loading dose of T4. T3 is given as an initial IV dose of 10 µg, followed by 10 µg every 8 to 12 hours until there is clinical improvement and the patient is able to take maintenance oral doses of T4. In addition, a loading dose of 200 to 300 µg of T4 is given intravenously, followed by 100 µg 24 hours later, and then a daily dose of 50 µg. The daily maintenance dose should then be adjusted based on laboratory findings and clinical improvement. Initially, TSH and free T4 levels should be closely followed to prevent overtreatment. After a stable daily dose is adopted, patients should have their thyroid function reevaluated in 4 to 6 weeks.
Nonthyroidal Illness Syndrome
Abnormal circulating levels of thyroid hormone are associated with many acute illnesses and do not necessarily indicate pathology at the level of the hypothalamus or pituitary ( Figure 21.1 ). Under normal conditions, T4 is converted by tissue 5′-monodeiodinase to T3, the metabolically active thyroid hormone. During periods of carbohydrate deprivation or illness, an alternative pathway of deiodination predominates and rT3, a biologically inactive hormone, is created. The clearance of rT3 also may be impaired because of the inhibited 5′-monodeiodinase activity. High circulating levels of proinflammatory cytokines and increased serum cortisol concentrations may promote this shift in deiodination. Medications frequently used in the ICU (e.g., amiodarone, glucocorticoids, propranolol) also can contribute to depressed T3 concentrations by inhibiting tissue 5′-monodeiodinase activity. With mild illness, only T3 levels decline but with increasing severity, a decrease in both T3 and T4 is observed without a concomitant elevation in TSH. These changes once were considered an adaptive response and the term euthyroid sick syndrome was adopted. Increasing evidence suggests that the thyroid abnormalities seen in critical illness reflect a state of central hypothyroidism. The designation of euthyroid sick syndrome has been abandoned in preference of the more metabolically neutral term nonthyroidal illness syndrome (NTIS) , which also is referred to as the low T3 syndrome .

Full access? Get Clinical Tree
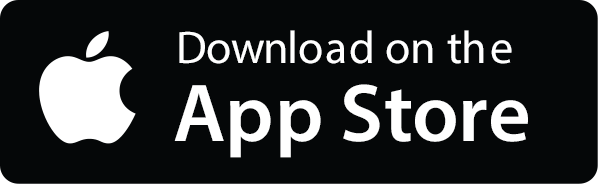
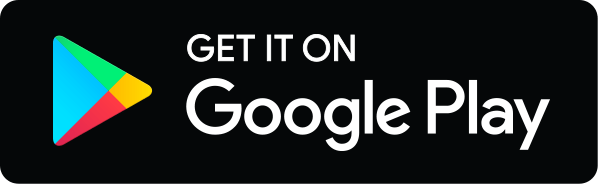