The concept of percutaneous access via small tubular or cylindrical portals is fundamental to the concept of minimally invasive and endoscopic spinal surgery.1 The tubular configuration of these access cannulas allows for the use of progressive, tissue-sparing dilation, as well as for a minimal amount of retraction pressure on adjacent vital tissues during the surgical procedure. Extensive literature has documented the less-invasive nature of portal access for a variety of spinal procedures with significant improvements in the degree of postoperative pain and disability.2–5 These portals come in a variety of shapes and sizes, and they can range from metallic to plastic and from rigid to flexible. As a principle, these tubular cannulas require some type of trocar device for insertion through the outer musculoligamentous wall to provide access and visualization of the target pathology and spinal column. Whereas standard retraction systems can often induce regional ischemia within the adjacent soft tissues, tubular retractors, by definition, exert minimal pressures on the tissue, thereby significantly improving tissue perfusion during prolonged procedures.6 Endoscopic Surgical Access Selection of the appropriate access portals will vary according to the specific nature and location of the operative procedure. For thoracoscopic procedures, simple small-diameter working portals are all that are required to provide access sheaths for the long-working instruments required for the procedure. In general, thoracoscopic portals are designed to accommodate only one instrument at a time, with subsequent ports placed for visualization and additional working instruments (Fig. 2–1A).4,7 As such, soft, flexible portals can be used during thoracoscopy because maintenance of a corridor of direct visualization through the port is not essential. This offers the benefit of less pressure on the intercostal neurovascular bundle at the edge of the rib and thus a decreased incidence of postoperative intercostal neuralgia.8,9 Like all portals, flexible thoracoscopic portals are available in a variety of working diameters, with the length being customizable by simply cutting off the excess. The presence of screw-type ridges on the portal walls helps to facilitate placement, stabilize it in the soft tissue, and decrease minor run-down-type venous bleeding that can often obscure intraoperative visualization. All thoracoscopic and laparoscopic portals typically have a widened cuff base to maintain their position on the chest or abdominal wall. This cuff can be sutured or stapled to the skin surface to further secure them during the procedure. Rigid portals used for laparoscopic procedures generally have a valve mechanism incorporated to seal the cannula, thereby allowing for carbon dioxide (CO2) insufflation that is typically integral to these abdominal procedures. Insufflation is usually not used, however, for the majority of thoracic procedures where simple deflation of the ipsilateral lung provides more than an adequate working space. Similar to the variety of portals available, the trocars used for their introduction are also available in a wide range of designs. For thoracoscopic procedures, initial blunt dissection with a tonsil into the intercostal space is typically followed by introduction of a simple rigid plastic trocar integral within the working portal.8–10 After proper placement within the intercostal space, the trocar is removed with the portal, then secured at the chest wall (Fig. 2–1B). Optical portals optionally allow for direct illuminated, endoscopic visualization during passage of the trocar tip to prevent inadvertent injury to the subjacent organs during initial placement of the working portals. These optical portals are of particular value in laparoscopic access because the abdominal contents are easily perforated during percutaneous cannulation. Once placed, these optical trocars can also be used to insufflate the working space, thereby pushing the visceral contents away from the abdominal wall and facilitating safe placement of subsequent working portals. When CO2-positive pressure insufflation is used, it is critical that the portals be vented intermittently and that a surveillance system is present to monitor intra-abdominal pressures. Self-venting, integrated laparoscopic control systems are critical in such procedures to prevent accidental vascular embolism and visceral injury. Careful attention to technique during portal placement will similarly help to maintain the seal required within the abdominal cavity for laparoscopic procedures. Specialized optical portals with integrated balloon-dilating mechanisms for preperitoneal and retroperitoneal exposures are available to facilitate entrance and dissection of these potential anatomic spaces.11 FIGURE 2–1 (A) Multiple access portals are typically placed during anterior thoracoscopic or laparoscopic procedures. Separate channels are used for the working instruments, suction, and camera, allowing for triangulation at the working target area. (B) Blunt plastic trocars are needed to introduce thoracoscopic portals into the thorax and to avoid inadvertent injury to the underlying parenchyma. (C) Serial tubular dilators are sequentially introduced through a dorsal approach to displace the posterior musculoligamentous complex gradually with a minimum of neurovascular and direct cautery injury. (D) Coaxial endoscopicassisted visualization is employed during small diameter approaches to the dorsal spine because the direct line of sight is greatly impaired through the small working portal. (E) Whereas rigid tubular portals are often adequate for simple decompressive procedures, such expandable cannulas as the Endius Flexposure portal (Plainville, MA) allow for wide surgical exposures at depth through a small dorsal incision. For endoscopic posterior spinal procedures, a series of metallic sequential tubular dilators is often substituted for a single introductory trocar. This allows for targeted placement of a small Steinmann pin or Kirschner wire to the region of interest and subsequent confirmation with fluoroscopy or image guidance. Progressive dilation with integral dilators gradually creates a working channel by splitting the muscular fibers along their natural orientation, thereby minimizing the amount of iatrogenic injury. After dilation to the desired diameter, a final working portal is placed, then secured to a bedmounted retractor (Fig. 2–1C). Unlike thoracoscopic or laparoscopic procedures, where the optical camera is placed through a separate portal, posterior spinal endoscopic procedures typically utilize a coaxial endoscopic camera to provide illumination and magnification of the working cannula (Fig. 2–1D). Surgical instruments and fixation are all applied directly through this same port. Examples of commercial access systems include the Access Port (Spinal Concepts; Austin, TX), the METRx Minimal Access System (Medtronic Sofamor Danek; Memphis, TN), the Nuvasive system (Nuvasive; San Diego, CA), and the ATAVI system (Endius; Plainville, MA). The central mechanism of each of these systems is still fundamentally that of tubular dilation and a cylindrical working portal. For more multilevel decompressive or fusion procedures, more extensile percutaneous exposure is provided by specialized cannulas that can be expanded after standard progressive tubular dilation. These include the Xpand system (Medtronic Sofamor Danek) and the ATAVI Flexposure cannula (Endius). With expansion, ultimate working diameters of up to 40 to 60 mm can be achieved with these systems (Fig. 2–1E). Operative Guidance During endoscopic spinal procedures, working within a confined space with a limited number of anatomical landmarks often leads to significant intraoperative disorientation. This leads to problems with localization, adequate decompression, complete lesion resection, proper correction of the preoperative deformity, and proper placement of spinal instrumentation. For example, correct localization of a thoracic herniated disk is often a daunting intraoperative task without clear anatomical landmarks within the thoracic cavity (Fig. 2–2A). As such, intraoperative fluoroscopy is essential to confirm the working level prior to decompression (Fig. 2–2B). Fluoroscopic confirmation of Steinmann pin placement is similarly essential during initial targeting for endoscopic posterior spinal procedures (Fig. 2–2C). To facilitate spinal fixation through very constrained working portals, significant improvements in implant design and materials over the last decade have resulted in improved strength, ergonomics, ease of use, and performance. Despite these advances, the safety and efficacy of screwbased spinal instrumentation continue to be limited by anatomical constraints. Whereas the lumbar spine pedicles are typically capacious, other fixation corridors (e.g., the high thoracic pedicles and the C1–C2 transarticular pathway) are often diminutive in caliber. Image guidance thus plays an essential role in improving the accuracy of screw placement in such anatomically challenging areas. Plain radiographs, fluoroscopy, and virtual multiplanar fluoroscopy have all been used for guidance during decompression and instrumentation for endoscopic spinal surgery. Although each modality provides significant operative value, none has been able to provide completely accurate axial or three-dimensional information to the surgeon (Fig. 2–2D). Although classic stereotactic volumetric image guidance systems such as these are able to provide some of this information, their use has been limited by the need for intraoperative coregistration of the preoperative dataset to the operative field (Fig. 2–2E). With the introduction of three-dimensional fluoroscopy, rapid acquisition of an intraoperative computed tomographic (CT) scan of the bony vertebral column is now possible (SireMobile Iso-C Fluoroscopic System; Siemens, Munich, Germany). This imaging technology represents a significant stride in the quest for an ideal image guidance modality for spinal instrumentation. Such intraoperative CT data provide for accurate, up-to-date anatomical information that can be reformatted in numerous projection planes (Fig. 2–2F). Thus, the need for coregistration to preoperative volumes is eliminated, thereby increasing the speed and cost effectiveness of operative cases. When merged with a frameless stereotactic image guidance system, targeting, decompression, and screw insertion can be planned, visualized, and tracked virtually according to the patient’s unique anatomy, thereby greatly improving the surgical efficacy of each component of the endoscopic procedure. FIGURE 2–2 Operative surgical access. (A) Frequent preoperative and (B) intraoperative localization of the target level and pathology is critical in planning and execution of minimally invasive portal-type approaches because there is little leeway for “cheating” up or down during the procedure. (C) During dorsal tubular approaches, the surgical approach is typically defined, to a large extent, by the trajectory of the initial Steinmann pin placement. As such, careful fluoroscopic confirmation of the initial location of the pin will greatly facilitate the overall success and ease of the procedure. (D) Stereotactic three-dimensional image guidance systems allow for real-time axial information, which helps the surgeon facilitate safe decompression and placement of instrumentation. In addition, 3-D “virtual” views can be provided thereby helping the surgeon compensate for the narrow minimally invasive access portals and their constrained field of view. (E) Intraoperative registration and the need for direct line-of-sight tracking of the working tools, however, greatly constrain the ergonomic facility of these technologies. (F) Use of intraoperative CT scans as provided by the Siemens Iso-C unit, which serves a dual function as a standard fluoroscopic arm and a simple motorized CT scan unit, can provide real-time intraoperative 3-D information and also avoid registration-type problems. (G) Real-time electromyography stimulation of pedicle screws with such systems as Neurovision (Nuvasive; San Diego, CA) helps to facilitate safe placement by detecting cortical breaches and potential injury to adjacent neural structures. In addition to these visual image guidance systems, neurologic surveillance and guidance are a potentially important aspect of endoscopic and minimally invasive spinal procedures. Because endoscopic procedures are often two-dimensional in their view, additional surveillance with somatosensory evoked potentials (SSEP) and stimulated electromyography (EMG) can provide useful adjuncts to prevent inadvertent neurologic injury during decompression and instrumentation. Whereas an abnormal SSEP is sometimes correlative with neurologic injury, a negative elicited EMG during pedicle cannulation strongly indicates intraosseous placement.12,13 Such commercial systems as Neurovision (Nuvasive; San Diego, CA) provide such intraoperative EMG thresholds to facilitate accurate pedicle screw placement during minimally invasive spinal instrumentation (Fig. 2–2G). Endoscopic Visualization Endoscope Considerations The operating endoscopes and cameras used during endoscopic and minimally invasive spinal procedures are used in a similar fashion to that of a standard operating microscope. Like microscopes, they provide illumination and magnification. Furthermore, they provide illumination at depth and can be steered directly over the working area to provide an unobstructed, clear visualization of the surgical field far superior to the long line of sight afforded by a microscope. For laparoscopic and thoracoscopic procedures, the surgical endoscopes used are typically rigid, glass-rod-type, 1 cm diameter endoscopes that provide a clear, high-resolution picture. For such procedures, there usually are no working ports in the endoscope because the surgical instruments are introduced through separate, additional working portals in the chest and abdominal wall. The surgeon works through a process of triangulating these operative instruments with the optic or endoscope positioned between them directly over the operating site (Fig. 2–3A). Endoscopes give the surgeon a view that is free from the obstruction that surgical instruments would cause in a standard line of sight viewed with loupes or a microscope. In posterior endoscopic-assisted procedures, the endoscope is mounted on a ring on the working portal that keeps it coaxial with the surgeon’s view and the trajectory of the operative instruments (see Fig. 2–1D,2–1E). By steering the endoscope downward, the surgeon is able to “see around” his or her own hands, thereby gaining an unobstructed field of view. Whereas the aforementioned procedures are endoscopically assisted, some spinal procedures truly work “through” the endoscope. The most common of these are the posterolateral lumbar diskectomy techniques popularized by Kambin, Yeung, and Tsou.14–16 With these techniques, dissection, decompression, cautery, and disk removal are often achieved directly through the endoscope, thereby necessitating a working channel of appropriate caliber that the surgical instruments can pass through (Fig. 2–3B). In addition, irrigating ports are needed in combination with appropriate valves on the endoscopes to manage the flow of fluid. Most hospitals today carry high-quality surgical endoscopes in their inventory for a variety of procedures, including cardiac, thoracic, esophageal, gastric, hepatic, urological, and gynecological procedures. The necessary system components of a digital camera, light cable, illumination source, signal processor, video monitor, image capture, video recorder, and image printer are also usually readily available as well. The spinal surgeon fundamentally should ensure that the telescope used will be a large (1 cm) diameter scope that can provide a broad field with variable illumination, zoom ability, adjustable focus, high-definition image and resolution, accurate color reproduction, and multiple offset angle choices. The advent of three-dimensional (3-D) dual-chamber endoscopes can provide the surgeon the additional benefits of depth perception during particularly complex or microsurgical-type tasks. The majority of surgical telescopes used today are still based on the time-proven rigid Hopkins lens system that couples air and multiple quartz rod lenses to provide a bright accurate image with minimal distortion. Flexible, low-resolution, or small-diameter endoscopes (e.g., ventriculoscopes) should be avoided for spinal procedures. A distal objective, a relay, and a proximal eyepiece lens are typically used in combination. For most telescopes, a working field of view subtends an 80- to 90-degree arc. Telescopes with varying angles of views or offsets ranging from 0 to 60 degrees are available (Fig. 2–3C). Whereas 0-degree endoscopes afford the least disorientation by providing a direct ahead view, off-axis telescopes provide the surgeon the ability to look around corners or edges at the depths of the operative field. The surgeon can also look around the entire thorax or abdomen by simply spinning the scope around its long axis. Finally, offaxis scopes can be placed well away from the working surgical instruments to avoid collision in the cavity.2,7,9,10,17, Disadvantages of offset telescopes include slightly less intense illumination and mild disorientation on the assistant’s behalf. We have found that 30-degree endoscopes are particularly versatile for spinal endoscopic-assisted procedures.4 Facility with both straight and offset telescopes is ultimately desirable. FIGURE 2–3 (A) Triangulation through multiple working portals allows for improved ergonomics and visualization during anterior thoracoscopic and laparoscopic procedures. Such triangulation is not possible during uniportal dorsal approaches. (B) Multiple working channels are required through the single portal to allow for simultaneous passage of the instruments, suction, and optic. (C) Multiple choices are available with regard to the working angle of the surgical endoscope to facilitate optimal intraoperative visualization. (D) The majority of endoscopic procedures are accomplished by the surgeon watching the procedure on large video monitors mounted throughout the operative suite visible to the entire surgical team. Transformation of the analog optical signal seen at the tip of the endoscope to a digital, transmissible image is accomplished through the use of a charged coupling device. Digital Imaging Whereas the original surgical endoscopes used an eyepiece for direct viewing and required that the surgeon place his or her face on or near the end of the sterile telescope, modern endoscopic spinal surgery uses lightweight digital cameras connected to video monitors for large, bright, magnified intraoperative visualization (Fig. 2–3D). The images can also be routed to other devices, including multiple video monitors for the rest of the surgical team and anesthesiology, video recording devices, still image digital capture devices, and photo printers. All this equipment is typically modular and can be mixed and matched for integration into a single ergonomic cart (Fig. 2–4A). A significant advance in surgical endoscopy was made with the advent of the charged coupling device (CCD), which made possible the conversion of an analog optical image into a clear, accurate, high-resolution digital signal via silicon optical chips. These specialized silicon chips contain a grid of thousands of photosensitive detectors, or pixels, that emit an electronic signal when struck by a photon. These signals are integrated and reconstructed by an in-line digital image processor. The CCD chip can be located either directly at the tip of the endoscope (i.e., “chip on a stick”) or, more commonly, on the extracavitary proximal end of the telescope. The processor then uses a series of analog transformations to reassemble the images into a television-type image that can be transmitted out to the operative viewing monitors. With advances in signal processing and color encoding, the images provided by such CCD telescopes far exceed those afforded by classical optical or fiberoptic methods. Furthermore, high-resolution flexible and three-dimensional endoscopes are now available thanks to breakthroughs in CCD technology. A brief overview of CCD technology is needed to understand the options available. CCD-type cameras originally employed a single chip with a single detector grid whose pixels differentially sensed red, green, or blue light. Although efficient, the color reproduction and resultant clarity of these images were not optimal, especially in the often reddish environs of the operative field. This led to the advent of the alternating singlechip CCD that utilized a 30-Hz strobe light to allow the sensing grid to cycle between sensing green, red, and blue light in ??? sec intervals. This color separation allowed for significantly improved clarity and color reproduction in the final reintegrated video image. The highest-quality images come from those cameras that simply use three-chip CCD to detect each color spectrum separately. Although the three-chip devices clearly provide the best images, they are also substantially more expensive than the single-chip alternating CCD-type cameras. Finally, it is crucial that the digital processor/integrator unit that is used to couple the camera to the monitor also be up-to-date to ensure the highest quality image processing and reconstruction. For the novice, one of the most dreaded hurdles of endoscopic spinal surgery is the lack of three-dimensional information. With advances, true 3-D endoscopic visualization is now possible by incorporating two separate optical channels into a single telescope. Much like real-life human binocular vision, such systems use two separate cameras that then transmit their respective images to the digital processor. Other techniques include a single lens with two full-color CCD images at slightly different angles in a single shaft and a splitting lens that separates a left and right image from a single main objective lens. All these techniques ultimately result in separate binocular images being sent to the digital video processor. Viewing of these separate images typically requires either a head-mounted visor display that yields two separate images to the respective eyes or special viewing glasses that flicker as the left and right images are alternately projected on a single-monitor screen (Fig. 2–4B). There is a special infrared emitter located at the top of the video screen that alternately “blacks out” either the right or left eyepiece of the glasses, depending on which image is being presented on the screen. Because of the discomfort, headache, and ergonomic limitations presented by both systems, popularity of 3-D visualization systems has been limited.2 Illumination The type and source of illumination available during the procedure are as important as the camera in many respects. Most modern operating rooms use either xenon or halogen illumination sources mounted via either liquid-filled or fiberoptic cables. Whereas liquid-filled cables are more durable, they conduct and heat up more rapidly, thereby requiring early connection to the endoscope. By contrast, fiberoptic cables remain cooler, but they are much more susceptible to breaking, with a gradual decrease in intensity as more fibers become damaged. The light sources typically range from 250 to 400 W and must be viewed with caution. The intensity of the light can be varied via automated or manual controls. Whereas xenon provides brighter illumination, halogen is often more useful operatively because the light is not as hot as that provided by the xenon source. Particular caution must be paid to not placing the tip of the endoscope or the light cable on the operative field because there have been documented cases of intraoperative ignition of paper drapes.2,9,10, Although brief contact with the patient’s tissues is associated with minimal risk of thermal injury, the surgeon and team should be cautious to avoid directly touching the tip of the endoscope upon removal from the body, except with a moist gauze to clean the lens. FIGURE 2–4 Digital imaging. (A) Because endoscopic procedures are digital-image based, numerous additional equipment options are available for archival and visualization purposes. The surgeon may choose to combine the monitors, camera, CCDs, printers, and recorders into a single cart to conserve space. (B) To provide true 3-D visualization from appropriate dual-chamber endoscopes, special heads-up binocular liquid crystal display projection systems are available (Vista; Salt Lake City, UT). (C) Robotic holding and endoscopic platforms provide additional ergonomic assistance, if so desired. Intraoperative Considerations Regardless of the offset angle of the endoscope, it is crucial that the surgeon rotate the camera at the end of the telescope to the proper orientation prior to beginning the operation. A surgical team typically should establish a convention (i.e., a standard view) such that every member of the surgical team should be oriented the same way. As such, the assistant holding the telescope and camera should know to keep the view so that the surgeon is oriented at all times. Because the endoscope and camera are significantly cooler and less humid than the body’s environment, intraoperative fogging of the lens is a primary concern during endoscopic spinal procedures. Prewarming the endoscope in a bucket or warm body-temperature saline for several minutes prior to introduction within the working cavity or portal can cut down on this fogging. In addition, sterile defogging solution, or FRED (Fog Reduction and Elimination Device; U.S. Surgical, Norwalk, CT), should be applied to the endoscope tip on the lens as a surfactant to reduce fogging. FRED can also be reapplied by soaking a small sponge stick and wiping the tip of the endoscope while it is still in the wound to reduce time needed for removing and reintroducing the optic each time it needs to be cleaned. Several proprietary systems exist for intracavitary cleaning of the endoscope as well. Some telescopes have an integrated washing and cleaning mechanism available for additional cost. A stable but adjustable view of the surgical field is critical for facilitating endoscopic spinal surgery. During the initial phases of the operation, when the orientation of the images is changed frequently, a surgical assistant can be devoted to holding the endoscope to maintain a relatively stable perspective; however, an assistant usually fatigues easily and poorly stabilizes images for long procedures. A moving image is difficult to follow and unnecessarily distracts the surgeon; therefore, mechanical endoscope holders are extremely useful for reliable fixation of the position of the endoscope in relation to the patient’s chest and spine. These devices use mechanical or pneumatic arms that are mounted onto the operating table (see Fig. 2–1E). Robotic, voice-activated computerized endoscope holders are also available (Fig. 2–4C). Endoscope holders simplify the operation, provide stable images, and free the assistant’s hands for other tasks. Image Capture and Recording Numerous options exist with regard to the capture and storage of intraoperative images during spinal endoscopic procedures. Images can be captured as still shots, brief video motion sequences, or continuous video recording. Archival format options include standard photographic images, digital images (JPEG, TIFF), S-VHS analog, 8 mm digital, regular or minidigital video (DV), and DVD digital disc. In the case of standard still-photo film photographs, a regular high-quality camera must be integrated into the telescope or camera prior to digital processing. Such capture devices are limited in their ease of use. With the advent of digital cameras, however, this process has been greatly simplified, and images can simply be fed from the digital video integrator and processor to a printer. Such devices can provide extremely highresolution capture of 3 to 5 megapixels in size. More limited, however, is the ability to print these images because most high-quality photo printers still produce images at or under 1200 × 1200 resolution. For video capture, most hospitals still routinely use S-VHS or 8 mm digital formats that are actually beneath the resolution of newer CCDs and processors. For maximal clarity, it is recommended that routine capture of video be accomplished through such true digital formats as that of the mini-DV tape or DVD disc recorders. These formats provide exceptionally crisp playback on standard home and office player units. Furthermore, they provide the ability for postproduction enhancement and editing on most computer laptops and workstations with only a minimal amount of additional equipment. Expensive proprietary archival systems available for image and video capture provide elegant in-line mechanisms to acquire, date, log, and store images in conjunction with demographic data, the operative timeline, and the surgeon’s annotations. Endoscopic Surgical Instruments On first glance, the operative instruments used during endoscopic spinal procedures are familiar to most spine surgeons; however, careful inspection soon reveals several significant differences. During thoracoscopic and laparoscopic procedures, the working length is substantially longer than that of standard open transcavitary surgery. The working distance can range from 14 to 30 cm, thereby necessitating modification of standard instruments. Endoscopic instruments used for these anterior spinal procedures are thus much longer and also have etched depth markings to facilitate visualization and orientation during two-dimensional dissection of the regional anatomy (Fig. 2–5A). Because of the long lever arm between the portal and the tip of the tools, the surgeon’s hand movements are often amplified. Surgeons must therefore adopt new strategies for manipulating tools precisely. The surgeon must often use both hands to anchor and guide the handle and shaft of the tools. The tool may also need to be steadied by stabilizing the shaft against the endoscopic portal within the chest wall. Lightweight tools (e.g., suctions, bipolar cauterization devices, endoscopic scissors, and forceps) can usually be controlled adequately with one hand. The surgeon can then work simultaneously with tools in both hands for dissection; however, to gain precise control of heavier tools (e.g., Kerrison rongeurs and curettes) or tools used close to the dura, the surgeon often must use both hands. FIGURE 2–5 Endoscopic surgical instruments. (A) For endoscopic procedures, modifications of standard surgical instruments are needed to improve the ergonomics and ease of use. Graduation with depth markings is invaluable to avoid accidental plunging and appropriate assessment of depth during such procedures. (B) For single-portal access procedures where line-of-sight considerations are limiting, bayoneting of the instruments and changes in their working angle and length are needed. (C) Integration of cautery into the surgical instruments helps reduce the number of objects placed into the portal at one time as well as the number of exchanges needed to accomplish a given surgical maneuver. Dissection Tools In their design, the majority of endoscopic spinal tools are very similar to open instruments. As mentioned earlier, they are typically much longer, with mechanisms that allow for shaft rotation to facilitate dissection at depth. Furthermore, beveling or angling of the working tip slightly off the main axis is frequently seen in endoscopic spinal instruments to allow for improved visualization of the working tip during surgery. These curvedtip instruments and dissectors are particularly useful to incise and dissect the fascia, pleura, or peritoneum away from the segmental vessels, spine, and chest wall. The working angle allows for such dissection with simple turns of the surgeon’s wrist during these long-distance manipulations. Some instrument systems (e.g., METRx; Medtronic Sofamor Danek) provide bayoneted instruments that are useful to keep the hands away from the central working corridor of a single working portal as well as to improve line-of-sight issues when direct vision (e.g., loupes or microscopes) is used (Fig. 2–5B). The hand grip interfaces are at first unfamiliar to spinal surgeons because they are often ring forceps or pistol grips or have some type of locking mechanism. Because placement of multiple instruments is often limited by only one access portal, many endoscopic spinal instruments have integrated irrigation, suction, and cautery mechanisms to obviate the need for placement of additional instruments through an often-constrained working corridor. Of particular use during thoracoscopic and laparoscopic procedures are integrated dissecting scissors (straight and curved), Debakey-type tissue forceps, right-angled clamps (e.g., Maryland clamp), pleural dissectors, and bipolars. Thus, dissection and cautery can be achieved with maximal ergonomic efficiency (Fig. 2–5C). Nerve root retractors with integrated suction are especially useful during endoscopic-assisted posterior spinal procedures. Many of these multipurpose instruments are available in disposable form for single use. Retractors During endoscopic procedures, retraction of adjacent structures is often challenging because of limited access afforded by the portals. As such, thoracoscopic and laparoscopic procedures routinely employ tissue-friendly forceps (e.g., lung forceps, Allis clamps, and Babcock clamps) to hold and move important structures away from the operative field. The fan-bladed retractors that are used to mobilize both lung and visceral structures away from the spine are especially important. These are available in a variety of designs, but all incorporate blunt-tipped, broad-surfaced blades that “fan” out after placement through the portal to allow for a broad, gentle retraction surface (Fig. 2–6A). These retractors frequently have an additional joint that allows for angling and towing in without additional manipulation of the working shaft angle, which is constrained by the access portal. With proper patient positioning and rotation of the patient, the atelectatic lung often can be encouraged to fall away from the spine, thereby minimizing excessive retraction of the lung and viscera to avoid inadvertent injury. FIGURE 2–6 (A) Such specialized tools as the fan-bladed design for thoracoscopic surgery are required to retract visceral structures during these procedures. (B) Integration of suction and cautery into an ergonomically friendly device is necessary to provide a clear operative field during endoscopic procedures. (C) Subtle changes in the working length and angle of endovascular clip appliers are needed to provide proper ligation and hemostasis during minimally invasive surgeries. Irrigation and Suction Like retraction issues, suction and irrigation during endoscopic procedures are also both critical and technically challenging to accomplish. Because the tools are often long, strong suction can be difficult to maintain. Excessive blood, cautery burn, and clot tend to absorb light, thus decreasing the illumination provided by the endoscope. To clear these undesirable elements, long Frazier-tipped or trumpet-type suction tools with integrated suction, irrigation, and cautery are needed. These specialized suction devices require an integrated means of delivering forceful irrigation to dislodge blood and clots from tissue surfaces (Fig. 2–6A–C). Pressurized bags of saline or intravenous fluid are typically mounted in-line with the suction tip to accomplish this goal. A cheaper alternative to such commercially available devices is a simple 50 cc syringe with a long central venous introducer sheath attached to it for irrigating within the thoracic or abdominal cavity. Hemostasis and Cautery Whereas the fundamental techniques of endoscopic hemostasis are the same as that of conventional open spinal surgery, the tools used, as with all instruments, require modification. As mentioned earlier, cautery is commonly integrated into many of the dissection, suction, and irrigation tools for ergonomic reasons. Both monopolar and bipolar cautery options are typically available, depending on the type of instrument being used. Dedicated cautery devices are also available in thoracoscopic, laparoscopic, and posterior endoscopic instrument sets. Because normal bipolars are useless in these procedures, longer, pistol-grip shafts are used. In addition, beveled bipolar tips are often helpful to reach underneath structures or beyond the immediate field of view. Ultrasonic or harmonic scalpels (Ethicon Endosurgery; Somerville, NJ) can be used as a combined cutting and cautery tool that allows for a shallow depth of penetration with a minimum of thermal damage, smoke, and adjacent tissue injury. With its blade that oscillates at 55 kHz and amplitude of 60 to 80 µ, it is able to denature collagen and effectively seal vessels smaller than 1 mm in diameter; however, it is not effective for hemostasis of larger vessels. In a similar fashion, hemoclip appliers must be modified for use in endoscopic procedures. Automated, disposable, multiuse clip appliers with rotating shafts allow for variable angle of placement or several clips without having to reload each time. These endoscopic clip appliers are available in a variety of working angles to facilitate dissection and placement underneath vascular structures. Because secure hemostasis of large-caliber segmental vessels often necessitates multiple clip placements, such rapid-fire devices are invaluable. With regard to hemostatic agents, such standard surgical agents as Gelfoam (Upjohn, Kalamazoo, MI), Avitene (C. R. Bard, Inc., Murray Hill, NJ), and Nu-Knit (Johnson & Johnson, Arlington, TX) can be used via specialized delivery systems. Bone wax applied on the long end of cherry cotton dissectors or endoscopic peanuts can be used in a standard fashion. Delivery of Gelfoam with thrombin or Avitene can be accomplished via long delivery cylinders with central plungers. The roll of Avitene is then expressed in the working field and delivered to the bleeding site via manipulation with a small cottonoid or dissector. The advent of powdered or gelatin granules of Gelfoam impregnated with thrombin (e.g., Floseal; Baxter Healthcare, Deerfield, IL) allows for delivery through a syringe and a long endoscopic tip directly into such bleeding sites as the epidural gutters. As the granules swell, local hemostasis is readily achieved. After a few minutes, moderate irrigation removes loose granules, leaving the clot in place (Fig. 2–7A). In our experience, the use of such agents has resulted in significant decrements in our operative blood loss. This experience echoes that of the cardiothoracic endoscopic experience as well. FIGURE 2–7 Endoscopic hemostatic and drills. (A) In addition to modified surgical instruments, modification of hemostatic agents with regard to form and shape help to facilitate hemostasis during minimally invasive procedures. Floseal (BaxterHealthcare) allows for local hemostasis through enlarging thrombin-soaked gelatin granules that allow for local tamponade and are not washed off during gentle irrigation as compared with standard pieces of thrombin-soaked Gelfoam. (B) For endoscopic procedures, additional length and smaller spin diameters are required in the drills used. (C) Because of the two-dimensional view and the inability to see proximal to the working area, shielded drill bits allow for additional safety during thoracoscopic and laparoscopic procedures. Endoscopic Drills As with standard open spinal procedures, the use of a modern, low-torque, high-speed drill is essential for the majority of endoscopic spinal procedures. High-speed pneumatic devices were preferred for most endoscopic bone dissection applications; however, such newer highspeed electric drills as the TPS system (Stryker, Kalamazoo, MI) are now able to deliver similar power and efficiency to that of pneumatic devices. The long bits and guiding shafts of endoscopic drills require additional stabilizing elements such as a pistol grip. This allows the operative surgeon stable, three-point control of the drill by bracing the pistol grip along the chest wall, thereby allowing for more precise control of the distal working tip. The Midas Rex drill system (Midas Rex Pneumatic Tools, Inc., Fort Worth, TX) has bits that are 25 cm (R-or L-attachments) or 40 cm long (Rx attachment), both of which are useful for thoracoscopic spine work (Fig. 2–7B). These attachments have a long, protective sheath that prevents injury to the lung and paraspinal soft tissue. A telescoping, adjustable sheath allows the surgeon to carry the length of the exposed drill tip to protect the soft tissues of the thorax. Various drill bits are available for endoscopic use (Fig. 2–7C). Coarse diamond burrs are useful for endoscopic drilling of the vertebral body but do create significant heat around neural structures. The coarse diamond burrs create bone dust slurry that fills the interstices of the cancellous bone and reduces bone bleeding without generating excessive debris within the chest cavity. Technological advances in endoscopic equipment and instrumentation have greatly expanded the indications for minimally invasive spine surgical procedures. Developments in imaging technology with better resolution of endoscopic images, greater use of working channels, and improved 3-D imaging will allow for improved surgical outcomes. Integration of endoscopic equipment with image-guidance and robotic systems presents an exciting promise for further refinement of endoscopic procedures. REFERENCES
2
Endoscopic Surgical Equipment
< div class='tao-gold-member'>
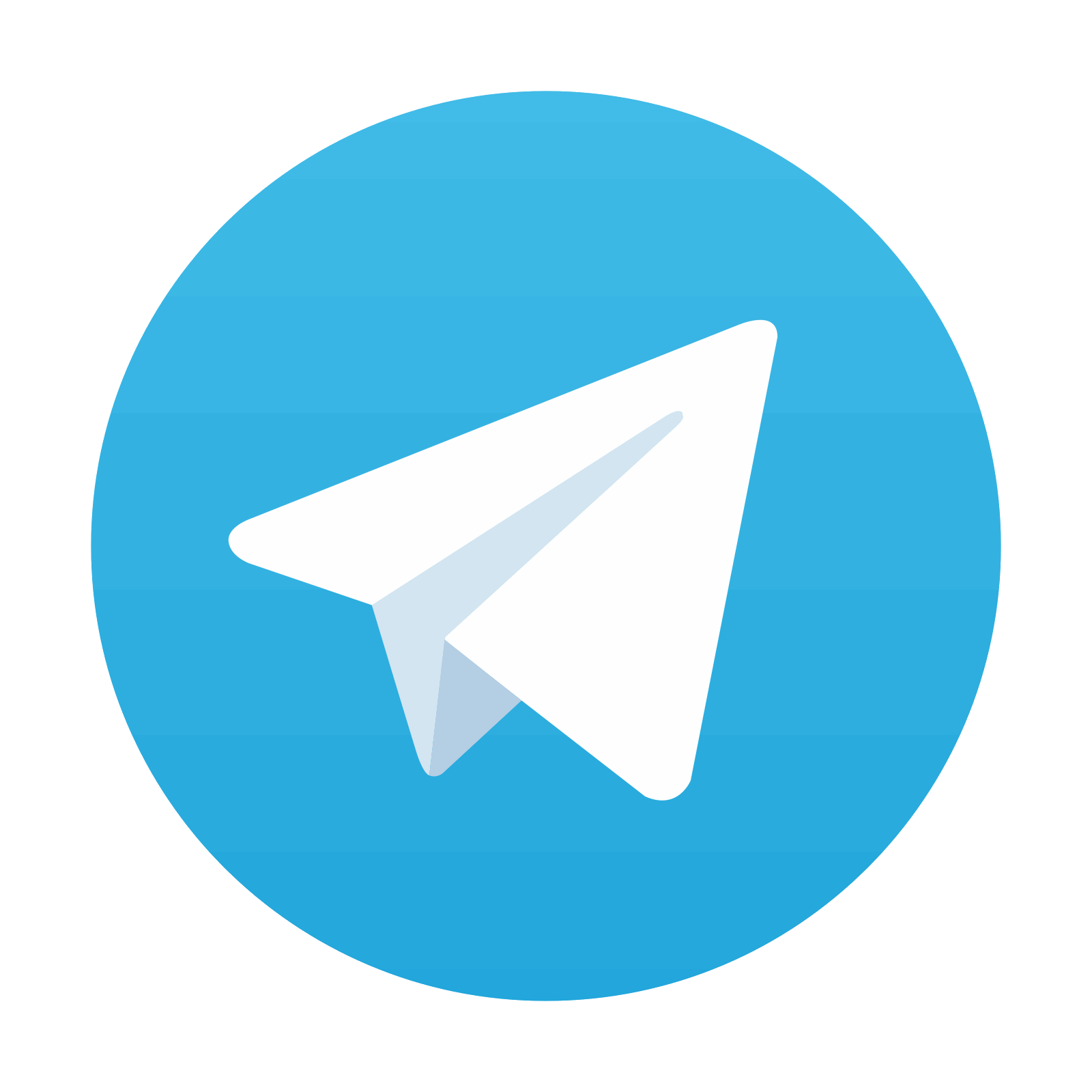