Epilepsy in the Setting of Inherited Metabolic and Mitochondrial Disorders
Linda D. Leary
Douglas R. Nordli Jr.
Darryl C. De Vivo
There are more than 11,000 well-recognized and well-characterized inherited disorders in humans, with nearly 200 of these associated with seizures and epilepsy. The daunting task for the clinician is to recognize these important diagnoses in the patient with epilepsy so that optimal medical treatment, family counseling, and prognosis can be provided. Often, the presentation is not distinct enough to allow precise identification of the disorder on the basis of clinical criteria alone. Instead, the physician must observe a patient over a period of time and begin screening tests to detect abnormalities suggestive of the underlying disorder. These abnormalities point the way toward further diagnostic evaluations, which may culminate in the definitive diagnosis of the inherited disorder and actual detection of the defective gene. In other circumstances, important clues are present when a child is first seen, but these features can be easily overlooked if the clinical data are not synthesized and analyzed in an orderly way. In general, the scalp electroencephalogram has low specificity but high sensitivity for diagnosing, determining severity, and monitoring brain dysfunction over a period of time in children of all ages. Although various electroencephalographic (EEG) patterns are reported in the literature as being typical of an inborn error of metabolism, rarity of metabolic disorders, ascertainment bias, and limited repertoire of possible EEG findings in the face of enormous variability in spectrum and severity of metabolic disorders reduce the usefulness of EEG in suggesting a specific diagnosis. EEG may, however, supplement clinical assessment and other test results in shortening the list of possible diagnoses.
How can clinicians mentally organize this wealth of material? One method is to group diseases according to categories on the basis of the subcellular organelle involved: mitochondrial, lysosomal, peroxisomal, and so on. Another is to group diseases according to metabolic or catabolic pathways, such as organic aciduria, aminoaciduria, and fatty acid oxidation. However, the clinical presentation within these groups may be diverse and dissimilar. Another method of grouping is to organize diseases according to their clinical presentation. This can be performed by age, but many different disorders are responsible for seizures and epilepsy within any defined age-group. Diseases may also be organized on the basis of specific characteristics of the seizures and the epilepsy syndromes. As each metabolic and mitochondrial disorder may present along a biologic spectrum, with more severe involvement presenting earlier and in a more devastating fashion, various epilepsy syndrome presentations are consistent with the same metabolic disorder. Metabolic and mitochondrial diseases can masquerade as forms of cryptogenic epilepsy. Once the etiology is established, the epilepsy classification will change to symptomatic, generalized epilepsy caused by a specific disorder (e.g., secondary to phenylketonuria [PKU]).
In the organization of this chapter, we group the various disorders by their age at onset and list, in tabular form, the various cryptogenic or symptomatic epilepsy syndromes that might be mistaken for these disorders. In addition, we
discuss clinical and EEG features of certain disorders that may provide clues to the underlying etiology. We also discuss the appropriate screening tests that may be performed, where applicable, followed by more definitive diagnostic procedures. For each condition in which a gene location is known, this is listed in parentheses with the appropriate chromosome and gene location.
discuss clinical and EEG features of certain disorders that may provide clues to the underlying etiology. We also discuss the appropriate screening tests that may be performed, where applicable, followed by more definitive diagnostic procedures. For each condition in which a gene location is known, this is listed in parentheses with the appropriate chromosome and gene location.
METABOLIC DISORDERS IN THE NEWBORN
Seizures are a clinical feature of a number of metabolic disorders presenting in the first month of life. Although the following section does not contain a comprehensive listing of all the disorders that should be considered in this age-group, it does include those in which seizures are a prominent feature.
Nonketotic Hyperglycinemia
In the inborn error of amino acid metabolism of nonketotic hyperglycinemia (NKH), large amounts of glycine accumulate in body fluids because of a defect in the multienzyme complex for glycine cleavage. The enzyme system is confined to the mitochondria and is composed of four protein components. Defects in three of these proteins have been identified. The most common defect, affecting the P protein (a glycine decarboxylase), is encoded by a gene localized to 9p22. The majority of cases present within the first 48 hours of life with lethargy, respiratory difficulties, and seizures that are often myoclonic or characterized as infantile spasms. Cortical malformations or corpus callosum defects may be present. A burst-suppression electroencephalogram pattern is characteristic, and hypsarrhythmia has also been seen (1).
Laboratory testing reveals elevations of glycine in the plasma, urine, and cerebrospinal fluid (CSF). The absence of ketones in the blood and urine, and of organic acids in the urine, helps to differentiate NKH from other conditions associated with hyperglycinemia, such as propionic acidemia and methylmalonic acidemia. The ratio of CSF to serum glycine can also be helpful, as it is significantly elevated in patients with NKH.
The pathophysiology of NKH has not been fully elucidated, but the elevated glycine is believed to impact the central nervous system (CNS) via its role as an inhibitory transmitter in the brainstem and spinal cord, and as an excitatory transmitter in the cortex (2).
There is no long-term effective treatment for this disorder. Valproic acid should be avoided because it induces hyperglycinemia. Strychnine, an antagonist to glycine, and diazepam have been reported to blunt seizures, but have not influenced the long-term outcome (3). Using high doses of benzoate to treat patients with NKH may reduce CSF glycine and improve seizure control, but it does not appear to stop the development of mental retardation (4,5). Because benzoate treatment may deplete carnitine levels, carnitine supplementation is recommended if benzoate is used (6). Prognosis is poor, with progressive microcephaly and intractable seizures. Death often occurs within the first days to months of life.
A transient form of NKH exists with similar early clinical and biochemical findings. In this condition, however, glycine concentrations normalize between 2 and 8 weeks of life, and prognosis is favorable.
Pyridoxine Dependency
In pyridoxine dependency, refractory seizures typically develop within the first several days of life. These may be characterized by infantile spasms or a variety of partial, myoclonic, and atonic features. The disorder results from a defect on the binding site of glutamic acid decarboxylase for which pyridoxine is a cofactor. This leads to insufficient γ-aminobutyric acid (GABA) formation, which can be corrected with administration of high doses of pyridoxine. Two different genes code for glutamic acid decarboxylase in mammalian brain, GAD1 (2q31) and GAD2 (10p11.23) (7,8). Genetic linkage analysis of five affected families excluded these genes as mutation sites for this disorder (9). In this study, a linkage to 5q31.2-q31.3 was identified.
The EEG is characterized by generalized bursts of high-voltage delta activity interspersed with spike and sharp waves and periods of asynchronous attenuation. Treatment with high doses (100 to 200 mg intravenously) of pyridoxine can result in cessation of seizures, conversion of the EEG to a burst-suppression pattern, and later normalization of the EEG with subsequent doses (10). In some patients, doses as high as 500 mg may be necessary to elicit a response. Early treatment is critical, as it improves developmental outcome, and maintenance therapy with pyridoxine is necessary. Onset of disease may extend beyond the newborn period, making this disorder a consideration in older infants with refractory seizures as well (11,12). Therefore, a trial of pyridoxine is recommended in all infants up to 18 months of age who have refractory seizures of unclear etiology.
Molybdenum Cofactor Deficiency and Sulfite Oxidase Deficiency
The rare conditions of molybdenum cofactor deficiency and sulfite oxidase deficiency present shortly after birth with a progressive encephalopathy, feeding difficulties, hypotonia, and refractory partial, myoclonic, or apparently generalized seizures. Dysmorphic features, lens dislocation, and hepatomegaly are all characteristic findings (13).
Multifocal paroxysms and a burst-suppression pattern have been described on the electroencephalogram. Neuroimaging may show poor differentiation between the gray
and white matter, severe cerebral and cerebellar atrophy, and multiple cystic cavities in the white matter.
and white matter, severe cerebral and cerebellar atrophy, and multiple cystic cavities in the white matter.
The relatively more common molybdenum cofactor deficiency results from an absence of the hepatic cofactor leading to a combined enzyme deficiency in the three molybdenum-dependent enzymes: sulfite oxidase, xanthine dehydrogenase, and aldehyde oxidase (14). This may be caused by mutations in the gene (MOCS1, 6p21.3) that encodes the two enzymes for synthesis of a precursor. Mutations in molybdopterin synthase (MOCS2, 5q11), which converts the precursor into the organic moiety, are responsible for a second form of the disorder (15). A third type of molybdenum cofactor deficiency is caused by a mutation in the gephyrin gene (GEPH, 14q24) whose gene product plays a role in biosynthesis of the cofactor (16). Molybdenum cofactor deficiency and isolated sulfite oxidase deficiency both result in high levels of urinary sulfites that can be screened for by sulfite dipstick on a fresh urine sample (17,18). The two disorders can be distinguished on laboratory testing as elevations of urine xanthine and hypoxanthine, and depressions of serum uric acid seen with molybdenum cofactor deficiency that are not present with isolated sulfite oxidase deficiency (19). The enzyme deficiencies can be demonstrated in cultured fibroblasts and liver tissue. No effective treatment has been identified and prognosis is poor, with death occurring within the first days to weeks of life.
Peroxisomal Disorders
Disorders of the peroxisome have been divided into three categories: (a) disorders of peroxisomal biogenesis (Zellweger syndrome [ZS], neonatal adrenoleukodystrophy, infantile Refsum disease); (b) disorders of a single peroxisomal enzyme (X-linked adrenoleukodystrophy, acylcoenzyme A [acyl-CoA] oxidase deficiency); and (c) disorders with deficiencies of multiple peroxisomal enzymes (rhizomelic chondrodysplasia punctata). The discussion that follows is limited to ZS, acyl-CoA oxidase deficiency, and neonatal adrenoleukodystrophy.
Zellweger Syndrome
ZS is the most common peroxisomal disorder in early infancy, with an estimated incidence of 1:50,000 to 1:100,000. The ZS phenotype is caused by mutations in any of several different genes involved in peroxisome biogenesis, including peroxin-1 (7q21-q22), peroxin-2 (8q21.1), peroxin-3 (6q23-q24), peroxin-5 (12p13.3), peroxin-6 (6p21.1), and peroxin-12 (chromosome 17). In addition to these, a ZS locus on 7q11 is suspected on the basis of chromosomal aberrations.
Dysmorphic features may be noted shortly after birth. Within the first week of life, the affected child develops a severe encephalopathy, hypotonia, and hyporeflexia. Seizures occur in 80% of patients, including partial, generalized tonic-clonic (rare), and myoclonic seizures, and atypical flexor spasms. Multisystem abnormalities of the brain, kidneys, liver, skeletal system, and eyes may occur. Eye abnormalities include cataracts, glaucoma, corneal clouding, optic nerve hypoplasia, pigmentary retinal degeneration, and Brushfield spots. The presence of the latter, along with hypotonia and a mongoloid appearance, may cause confusion in the diagnosis of Down syndrome versus ZS. Findings on neuroimaging and pathologic examination are distinctive, with pachygyria or polymicrogyria localized to the opercular region and cerebellar heterotopias.
Patients with ZS have partial motor seizures originating in the arms, legs, or face. The seizures do not culminate in generalized seizures and are easily controlled with antiepileptic drugs (AEDs). The interictal electroencephalogram of patients with ZS shows infrequent bilateral independent multifocal spikes, predominantly in the frontal motor cortex and surrounding regions (20). Less frequently, hypsarrhythmia is observed.
ZS is the most severe of the peroxisomal disorders, with an apparently reduced number or absence of peroxisomes. Santos and associates (21) discovered the presence of peroxisomal ghosts that contained peroxisomal membrane proteins in patients with ZS. These ghosts lack catalase function and many or all proteins of the matrix. An accumulation of very-long-chain fatty acids occurs from a reduction in peroxisomal β-oxidation enzymes. The elevation of very-long-chain fatty acids in the serum helps determine the diagnosis. Treatment is primarily supportive, with dietary therapy being tested in milder forms of the disease.
Neonatal Adrenoleukodystrophy
Neonatal adrenoleukodystrophy, an autosomal recessive disorder with pathologic and biochemical findings resembling X-linked adrenoleukodystrophy, bears some similarity to ZS. Distinguishing features include absent or minimal facial dysmorphisms, later onset of seizures, absent or minimal cerebral malformations, and longer life span. Frequent, often intractable seizures occur that may be tonic, clonic, myoclonic, or epileptic spasms (22). No characteristic EEG pattern has been defined, but descriptions have included high-voltage slowing, polymorphic delta activity, multifocal paroxysmal discharges, burst suppression, and hypsarrhythmia. There is evidence that this disorder may be caused by a number of different gene defects, including a mutation in the peroxisomal targeting signal-1 receptor gene PXR1, and mutations in the peroxin-1 gene, peroxin-10 gene (chromosome 1), and peroxin-13 gene (2p15) (23).
Acyl-Coenzyme A Oxidase Deficiency
Acyl-CoA oxidase deficiency was initially described in two siblings by Poll-The and colleagues (24). Clinical features included hypotonia, pigmentary retinopathy, hearing loss, developmental delay, adrenocortical insufficiency, absence of dysmorphic features, and onset of seizures shortly after birth. A deficiency in acyl-CoA oxidase was identified, resulting from a deletion in its coding gene (17q25). In
children with acyl-CoA oxidase deficiency, serum very-long-chain fatty acid levels are elevated, whereas pipecolic acid levels are normal. Cortical malformations are generally absent, and the interictal EEG may show continuous diffuse high-voltage theta activity.
children with acyl-CoA oxidase deficiency, serum very-long-chain fatty acid levels are elevated, whereas pipecolic acid levels are normal. Cortical malformations are generally absent, and the interictal EEG may show continuous diffuse high-voltage theta activity.
Urea Cycle Disorders
Of the six enzymes in the urea cycle that are involved in the conversion of ammonia to urea, five may be defective and cause neonatal seizures. These deficiencies, with their estimated frequency in the general population are as follows: carbamoyl phosphate synthetase, 1:800,000; ornithine transcarbamoylase, 1:80,000; argininosuccinate synthetase, 1:250,000; argininosuccinate lyase, 1:70,000; and N-acetylglutamate synthetase, only a few cases reported (2). The clinical disorder resulting from a deficiency in the sixth enzyme, arginase, presents later in infancy. All these disorders are autosomal-recessive except for ornithine transcarbamoylase deficiency, which is X-linked dominant.
The clinical manifestations of the disorders are similar and result, at least in part, from ammonia elevations. Typically, affected newborns present with poor feeding, emesis, hyperventilation, lethargy, or convulsions 1 to 5 days after birth. These signs lead to deepening coma, with decorticate and decerebrate posturing and progressive loss of brainstem function. Brain imaging and pathology reveal cerebral edema with pronounced astrocytic swelling. Ammonium is taken up by the astrocytes and rapidly converted to glutamine in an energy-dependent process. The edema is associated with glutamine accumulation and energy depletion. In experimental models, blocking glutamine synthesis has prevented the occurrence of hyperammonemia-associated cerebral edema (25).
The clinical diagnosis is confirmed by elevations in serum ammonia, absence of urine ketones, and respiratory alkalosis. (In contrast, metabolic acidosis and ketosis frequently occur with disorders of organic acid or pyruvate metabolism.) Measurement of plasma citrulline and argininosuccinic acid, as well as urine orotic acid, can help differentiate the enzymatic defects. Definitive diagnosis is established via enzyme assays or deoxyribonucleic acid (DNA) analysis.
The EEG shows a low-voltage pattern, with diffuse slowing and multifocal epileptiform discharges (26). Two patients studied by Verma and coworkers (27) in 1984 demonstrated episodes of sustained monorhythmic theta activity. In patients with acute neonatal citrullinemia, a burst-suppression pattern has been described (28).
In the acute setting, hemodialysis has been used to reduce serum ammonia and can be lifesaving. Protein restriction and medical therapy aimed at lowering serum ammonia are recommended in the long-term management of these children. Liver transplantation has been successful in reducing ammonia levels in patients and in reversing neurologic deficits in adults with milder disease (29,30).
Maple Syrup Urine Disease
Maple syrup urine disease, resulting from a defect in the branched-chain α-keto acid dehydrogenase complex, was first reported by Menkes and colleagues (31) in 1954. The enzyme defect leads to accumulation of the branched-chain amino acids—valine, leucine, isoleucine—and their keto acids in body tissues and fluids. The gene locus is 19q13.1-q13.2 (32). Pathologic studies reveal diffuse myelin loss and increased total brain lipid content. Cystic degeneration of the white matter associated with gliosis is observed. Disordered neuronal migration may occur with heterotopias and disrupted cortical lamination.
Feeding difficulties and lethargy are observed during the first to second weeks of life. If left untreated, these signs may progress to stupor, apnea, opisthotonos, myoclonic jerks, and partial and generalized seizures.
A characteristic odor can be detected in the urine and cerumen, but this may not be detectable until several weeks after birth. Laboratory testing reveals a metabolic acidosis and elevated blood and urine ketones. Hypoglycemia may be present, exacerbating seizures. Ferric chloride testing of the urine causes a gray-green reaction, and the 2,4-dinitrophenylhydrazine test is positive. A marked elevation in branched-chain amino acids/branched-chain keto acids in the plasma, urine, and CSF is observed, and the presence of the abnormal metabolite L-alloisoleucine is pathognomonic for this condition. Definitive testing can be performed by enzyme assay.
The electroencephalogram shows diffuse slowing and a loss of reactivity to auditory stimuli. The “comb-like rhythm” characteristic of maple syrup urine disease was initially reported by Trottier and associates (33) in 1975, when bursts of a central mu-like rhythm were observed in four affected patients. Tharp (34) described resolution of this pattern in an affected infant when dietary therapy was initiated. Korein and coworkers (35) observed a paroxysmal spike and spike-wave response to photic stimulation in 7 of 15 affected patients. Acute treatment is aimed at counteracting the effects of hypoglycemia, acidosis, and hypotension. Dialysis or exchange transfusion rarely is necessary. Dietary therapy with protein restriction, thiamine supplementation, and elimination of branched-chain amino acids from the diet is the mainstay of treatment.
Organic Acidurias
The disorders of organic acid metabolism comprise a large number of inborn errors, including isovaleric aciduria and several ketotic hyperglycinemic syndromes (propionic acidemia, methylmalonic acidemia, and β-ketothiolase deficiency).
Symptoms develop during the neonatal period in half of the children with isovaleric aciduria (gene locus, 15q14-q15) (36), with poor feeding, vomiting, dehydration, and a progressive encephalopathy manifested by
lethargy, tremors, seizures, and coma. Depressed platelets and leukocytes may be seen, and the urine odor has been described as similar to that of “sweaty feet” (37). Cerebral edema is present, and seizures are most often partial motor or generalized tonic. The electroencephalogram shows dysmature features during sleep. Distinctive biochemical findings include metabolic acidosis, ketosis, lactic acidosis, hyperammonemia, and transient bone marrow suppression. High urine concentrations of isovalerylglycine, a by-product of an alternative pathway of detoxification by glycine-N-acylase, is diagnostic.
lethargy, tremors, seizures, and coma. Depressed platelets and leukocytes may be seen, and the urine odor has been described as similar to that of “sweaty feet” (37). Cerebral edema is present, and seizures are most often partial motor or generalized tonic. The electroencephalogram shows dysmature features during sleep. Distinctive biochemical findings include metabolic acidosis, ketosis, lactic acidosis, hyperammonemia, and transient bone marrow suppression. High urine concentrations of isovalerylglycine, a by-product of an alternative pathway of detoxification by glycine-N-acylase, is diagnostic.
The symptoms of propionic acidemia also appear during the neonatal period, with 20% of affected newborns having seizures as the first symptom. Characteristic features include vomiting, lethargy, ketosis, neutropenia, periodic thrombocytopenia, hypogammaglobulinemia, developmental retardation, and intolerance to protein. Patients may have very puffy cheeks and an exaggerated cupid’sbow upper lip. Mutations have been identified in both the α subunit (13q32) and β subunit (3q21-q22) of propionyl coenzyme A (CoA) carboxylase (38). Convulsions are typical, although partial seizures have also been reported. The electroencephalogram shows background disorganization, with marked frontotemporal and occipital slow-wave activity (39). In 40% of children, myoclonic seizures develop in later infancy, and older children may have atypical absence seizures. Biochemical findings include metabolic acidosis, ketosis, and elevation of branched-chain amino acids and propionic acid.
Methylmalonic acidemia may be caused by deficiencies of the enzyme methylmalonyl-CoA mutase or of adenosylcobalamin synthetic enzymes. Methylmalonic acidemia occurs in association with homocystinuria in a combined deficiency of methylmalonic-CoA mutase and methyl-tetrahydrofolate:homocysteine methyltransferase (40,41). Forms responsive to vitamin B12 have been reported (42). Stomatitis, glossitis, developmental delay, failure to thrive, and seizures are the major features. Diffuse tonic seizures and partial seizures with secondary generalization are the most frequent seizure types. Seizures may be characterized by eyelid clonus with simultaneous upward deviation of the eyes. In a review of 22 patients, Stigsby and collaborators (39) described abnormalities on the electroencephalogram in seven patients, consisting of multifocal spike discharges and depressed background activity in two, excessive generalized slowing in two, and mild background slowing with lack of sleep spindles in three. Two children were reported to have myoclonus and a hypsarrhythmic EEG pattern (43). Lesions of the globus pallidus on computed tomography or magnetic resonance imaging (MRI) are characteristic.
Fatty Acid Oxidation Defects
Deficiencies of carnitine palmitoyltransferase types I and II are common disorders of mitochondrial fatty acid oxidation and may present in the newborn period (44). The infantile type of carnitine palmitoyltransferase II deficiency presents as severe attacks of hypoketotic hypoglycemia, sometimes associated with cardiac damage, culminating in sudden death. In addition, cystic dysplasia of the brain has been noted. A deficiency in carnitine acylcarnitine translocase also may produce seizures, apnea, and bradycardia in the neonatal period. Seizures may occur in other defects of fatty acid oxidation, most notably in short-chain acyl-CoA dehydrogenase deficiency (45).
Pyruvate Dehydrogenase Deficiency, Pyruvate Carboxylase Deficiency, and Leigh Syndrome
The pyruvate dehydrogenase complex is composed of multiple copies of three enzymes: pyruvate decarboxylase (E1), dihydrolipoyl transacetylase (E2), and dihydrolipoyl dehydrogenase (E3). The core of the complex is formed by 60 E2 subunits, and the other enzymes are attached to the surface. The E1 enzyme is itself a complex structure, a heterotetramer of two α and two β subunits. The E1 α subunit is particularly important, as it contains the E1 active site; its gene locus is Xp22.2-p22.1. Pyruvate dehydrogenase deficiency has a wide variety of clinical presentations, ranging from acute lactic acidosis in infancy with severe neurologic impairment to a slowly progressive neurodegenerative disorder (46). It is one of the most common of the defined genetic defects of mitochondrial energy metabolism. Seizures frequently occur and may be manifested by infantile spasms and myoclonic seizures (47). EEG findings include multifocal slow spike-and-wave discharges. Structural abnormalities, such as agenesis of the corpus callosum, are often present on neuroimaging. The most common mutations associated with this disorder are in the E1 subunit gene located on the short arm of the X chromosome.
Two predominant clinical presentations occur with pyruvate carboxylase deficiency. The neonatal type manifests with severe lactic acidemia and death in the first few months of life. The juvenile presentation begins in the first 6 months of life with episodes of lactic acidemia precipitated by an infection. Developmental delay, failure to thrive, hypotonia, and seizures, including infantile spasms with hypsarrhythmia, may be seen. In milder forms, diffuse 1.5- to 3-Hz slowing has been noted (39). Seizures are related to the hypoglycemia that occurs secondary to Krebs cycle dysfunction. Treatment with the ketogenic diet or corticotropins may markedly exacerbate the disorder and should be avoided (48,49).
Leigh syndrome (subacute necrotizing encephalomyelopathy) may be related to various metabolic defects, including cytochrome c oxidase deficiency and defects in other enzymes involved in energy metabolism. Mutations in the mitochondrial DNA-encoded adenosine triphosphate (ATP)-6 subunit of ATP synthase (complex V) and any of the three catalytic subunits (E1, E2, or E3) of the
pyruvate dehydrogenase complex may result in the same phenotype described by Leigh in 1951 (50,51). The syndrome may also be caused by isolated deficiency in mitochondrial complex I (nicotinamide adenine dinucleotide: ubiquinone oxidoreductase) and complex II. In addition, rare causes of Leigh syndrome include myoclonic epilepsy with ragged-red fibers (MERRF), mitochondrial encephalomyopathy with lactic acidosis and stroke-like episodes (MELAS), and mitochondrial DNA depletion (52,53). Within the first year of life, classic features include failure to thrive, lactic acidosis, and developmental delay. The disease progresses with spasticity, abnormal eye movements, and central respiratory failure. A variety of different seizures, including focal and generalized seizures, have been described (54). Cases of infantile spasms and hypsarrhythmia have been reported with Leigh syndrome (55,56). In addition, there have been several cases of epilepsia partialis continua (57). EEG features do not appear to be distinctive enough to contribute to the clinical diagnosis of Leigh syndrome (58).
pyruvate dehydrogenase complex may result in the same phenotype described by Leigh in 1951 (50,51). The syndrome may also be caused by isolated deficiency in mitochondrial complex I (nicotinamide adenine dinucleotide: ubiquinone oxidoreductase) and complex II. In addition, rare causes of Leigh syndrome include myoclonic epilepsy with ragged-red fibers (MERRF), mitochondrial encephalomyopathy with lactic acidosis and stroke-like episodes (MELAS), and mitochondrial DNA depletion (52,53). Within the first year of life, classic features include failure to thrive, lactic acidosis, and developmental delay. The disease progresses with spasticity, abnormal eye movements, and central respiratory failure. A variety of different seizures, including focal and generalized seizures, have been described (54). Cases of infantile spasms and hypsarrhythmia have been reported with Leigh syndrome (55,56). In addition, there have been several cases of epilepsia partialis continua (57). EEG features do not appear to be distinctive enough to contribute to the clinical diagnosis of Leigh syndrome (58).
Disorders of Carbohydrate Metabolism
Fructose-1,6-bisphosphatase deficiency, a rare, potentially life-threatening disorder of gluconeogenesis, presents within the first few days of life with respiratory abnormalities, hypotonia, lethargy, hepatomegaly, irritability, and convulsions. Laboratory findings reveal lactic acidosis, ketosis, hypoglycemia, elevated plasma concentrations of alanine, and the presence of abnormal urinary organic acids with glycerol and glycerol-3-phosphate. The gene location is 9q22.2-q22.3 (59). Neurologic sequelae can be prevented by avoidance of hypoglycemia.
Hereditary fructose intolerance (fructose-1,6-biphosphate aldolase deficiency) may be seen in the neonatal period in infants who are formula fed and given fructose or sucrose early in life. Symptoms include profound hypoglycemia, emesis, and convulsions. If the disease is readily diagnosed, fructose and sucrose can be eliminated from the diet before significant systemic injury occurs.
Glut-1 deficiency syndrome, a disorder of glucose transport, may present with seizures in the neonatal period. Because the disorder typically presents later in infancy, it is discussed in the section on metabolic disorders of infancy.
Early Onset Multiple Carboxylase Deficiency (Holocarboxylase Synthetase Deficiency)
The rare disorder of early onset multiple carboxylase deficiency presents in the first week of life with lethargy, respiratory abnormalities, irritability, poor feeding, and emesis. A skin rash is present in more than 50% of patients. Generalized tonic convulsions, partial motor seizures, and multifocal myoclonic jerks develop in 25% to 50% of cases.
A deficiency in the enzyme holocarboxylase synthetase leads to a decrease in holocarboxylase (60). As this enzyme links biotin to four carboxylases in the mitochondria and one in the cytosol, an inactivity of all carboxylases results. The gene location is 21q22.1. Although rare, this condition is very important to recognize because prompt treatment with biotin may result in dramatic improvement.
Laboratory findings demonstrate ketoacidosis and organic aciduria. Hyperammonemia may be seen with acute episodes. Electrographically, a burst-suppression pattern or multifocal spikes are observed. Definitive diagnosis can be made by enzyme assays, and prenatal testing is available. The gene defect has been localized to chromosome 21q22.1. Recently, Aoki and associates (61) reported seven mutations (three missense, two single-base-pair deletions, a three-base in-frame deletion, and a 68-basepair deletion) identified in the complementary DNA (cDNA) of seven patients from Europe and the Middle East with holocarboxylase synthetase deficiency.
Treatment with biotin (10 mg/day) produces clinical improvement, and there is evidence that prenatal maternal treatment may be helpful.
METABOLIC DISORDERS OF EARLY INFANCY
Lysosomal Disorders
Tay-Sachs Disease and Sandhoff Disease
GM2 gangliosidosis is a lysosomal disorder that invariably includes seizures as a prominent feature. The infantile forms of GM2 gangliosidosis include Tay-Sachs disease, caused by a deficiency in hexosaminidase A, and Sandhoff disease, caused by a deficiency in hexosaminidase A and B. The clinical presentation is that of a progressive encephalopathy.
Tay-Sachs disease, an autosomal recessive disorder localized to chromosome 15 (15q23-q24) (62), is found in the Ashkenazi Jewish population of Eastern or Central European descent. The overall incidence in the general population (1in 112,000 livebirths) increases to 1 in 3900 in this defined group. The enzymatic defect leads to intraneuronal accumulation of GM2 ganglioside. Normal development is seen until 4 to 6 months of age, when hypotonia and loss of motor skills occur. Within the next 1 to 2 years, spasticity, blindness, and macrocephaly develop. At this stage, seizures become prominent, with frequent partial motor, complex partial, and atypical absence seizures that respond poorly to medication. Myoclonic jerks are frequent and are often triggered by an exaggerated startle response to noise. The electroencephalogram is normal early in the course of disease. Gradually, background activity slows, with bursts of high-voltage delta activity and very fast central spikes (63). Diffuse spike and sharp-wave activity may be noted with acoustically induced myoclonic seizures. As the disease progresses, EEG amplitude declines. The classic cherry-red spot is present in the ocular fundi of more than 90% of patients. Enzymatic studies reveal an isolated absence or deficiency in hexosaminidase
A activity. Prenatal diagnosis and carrier detection for high-risk populations are available.
A activity. Prenatal diagnosis and carrier detection for high-risk populations are available.
Sandhoff disease is associated with a mutation of the β-subunit of hexosaminidase, located on chromosome 5 (5q13) (64). Unlike Tay-Sachs disease, there is no association with a particular ethnic group. Clinical presentation is similar to that of Tay-Sachs; however, distinguishing features in some patients include hepatosplenomegaly and skeletal involvement. Enzymatic testing demonstrates the diminished activity of hexosaminidase A and B. Detection of N-acetylglucosamine-containing oligosaccharides in the urine and foam cells in the bone marrow is also diagnostic. As with Tay-Sachs disease, no treatment is immediately available, although one possible strategy is to deplete substrate using an inhibitor of glycosphingolipid biosynthesis, such as N-butyldeoxynojirimycin.
Krabbe Disease (Globoid Cell Leukodystrophy)
Another lysosomal disorder occurring in this age-group is globoid cell leukodystrophy (Krabbe disease). This disorder, linked to chromosome 14q31, is caused by deficient activity of galactosylceramidase. There are four forms of the disorder: (a) infantile, with onset before age 6 months; (b) late infantile, with onset between ages 6 months and 3 years; (c) juvenile, with onset between ages 3 and 7 years; and (d) adult, with onset after 7 years of age (65). The majority of cases begin within 3 to 6 months of life with irritability, poor feeding, emesis, and rigidity. Muscular spasms induced by stimulation are prominent. Blindness and optic atrophy ensue. Initially, increased tendon reflexes are present and then gradually diminish as breakdown of peripheral myelin occurs. Partial or generalized clonic or tonic seizures, as well as infantile spasms, are seen, which may be difficult to distinguish from muscular spasms (66,67). In contrast to what is observed in many classic white matter diseases, seizures occur early in the course of Krabbe disease in 50% to 75% of infants with the disorder. EEG characteristics include a hypsarrhythmia-like pattern with irregular slow activity and multifocal discharges of lower amplitude than that typically seen with West syndrome (68). In a 1969 study of seven infants by Kliemann and coworkers (68), six children had prominent β activity occurring independently in the posterior temporal regions and vertex that was superimposed over slower, high-amplitude waves. This activity was observed to be state-dependent and to occur in long runs without any apparent clinical manifestations. In the terminal stages of the disease, little electrical activity is detected. Extensive demyelination of the central and peripheral nervous systems occurs, leading to the classic laboratory features of delayed nerve conduction velocities and elevated CSF protein. The presence of multinucleated macrophages containing galactocerebroside (“globoid cells”) is the pathologic hallmark of the disease. The disease is relentlessly progressive, with death by 1 to 2 years of age. Recently, bone marrow transplantation has been a promising treatment option for these patients (69).
GM1 Gangliosidosis Types I and II
The infantile form of GM1 gangliosidosis, type I, has been localized to chromosome 3 (3p21.33). A deficiency in β-galactosidase leads to the accumulation of GM1 ganglioside and degradation products in nerve cells and other tissues. The affected child is initially normal and then has regression of development at 3 to 6 months of age, with rapid neurologic deterioration. Seizures develop by 2 years of age. Clinical features may include coarse facial features, hepatomegaly, bone deformities (dysostosis multiplex), visual abnormalities, hypotonia, progressive microcephaly, and hematologic abnormalities. A macular cherry-red spot can be seen. Diagnosis is determined by urine findings of galactose-containing oligosaccharides in association with elevated keratan sulfate, by characteristic vacuolization in blood lymphocytes or bone marrow, and by distinctive findings on long bone and spine radiographs. Definitive testing by enzymatic assay is available.
Neurologic deterioration in the juvenile form of GM1 gangliosidosis type II is generally slower than in type I. Cerebral manifestations with regression of developmental milestones and visual symptoms are typically present by 2 to 4 years of age. EEG features of both forms include background slowing, with increasing, irregular slow activity as the disease progresses (70). In type II, a fluctuating 4- to 5-cycle temporal rhythmic discharge has been observed.
Disorders of Vitamin Metabolism
Disorders of vitamin metabolism with symptoms that appear in early infancy include biotinidase deficiency and folic acid disorders.
Late-Onset Multiple Carboxylase Deficiency (Biotinidase Deficiency)
Seizures are a prominent feature of late-onset multiple carboxylase deficiency, occurring in 50% to 75% of affected children. Onset of symptoms begins at 3 to 6 months of age, with hypotonia and developmental delay. Seborrheic or atopic dermatitis and alopecia are common. As the disease progresses, ataxia, optic atrophy, and sensorineural hearing loss develop. Seizures, which may be generalized tonic-clonic, partial, myoclonic, or infantile spasms, are the presenting feature in 38% of patients. EEG findings may include a suppression-burst pattern, absence of physiologic sleep patterns, poorly organized and slow waking background activity, and frequent spike and spike-and-slow-wave discharges (71). A deficiency in biotinidase, the enzyme that cleaves biotin from its precursor, can be demonstrated in the serum, leukocytes, or culture fibroblasts. Incidence is estimated at 1 in 40,000 livebirths, and the gene locus is 3p25 (72). As this is a treatable condition, therapeutic trials with high-dose oral biotin should be considered in infants with developmental delay and persistent seizures of unknown etiology.
Methylenetetrahydrofolate Reductase Deficiency
Methylenetetrahydrofolate reductase deficiency (1p36.3) (73) is the most common inborn error of folate metabolism. The metabolic defect results from insufficient production of 5-methyltetrahydrofolate, which is needed for the remethylation of homocysteine to methionine, because of a deficiency in methylenetetrahydrofolate reductase. In affected individuals, a progressive neurologic syndrome develops in infancy. Children with this disorder have acquired microcephaly and seizures characterized by intractable infantile spasms, generalized atonic and myoclonic seizures, and partial motor seizures. EEG findings vary from diffuse slowing of background activity to continuous spike-wave complexes or multifocal spikes. The early onset form differs from the late-onset form. The latter presents with progressive motor deterioration, schizophrenia-like psychiatric symptoms, and recurrent strokes; seizures are uncommon (74). Homocystinuria and elevated serum concentrations of homocystine with reduced or normal serum methionine are the main biochemical features. Dietary supplementation with folic acid, betaine, and methionine has proven beneficial (75). In the acute setting, high-dose methionine has been effective in stopping seizures.
Defects in methionine biosynthesis are also associated with seizures. Convulsions are frequent and are predominantly generalized, although myoclonic seizures with hypsarrhythmia have been reported. Diagnostic laboratory findings are megaloblastic anemia, homocystinuria, decreased methionine, and normal folate and cobalamin concentrations in the absence of methylmalonic aciduria.
Congenital Folate Malabsorption
Seizures are common in patients with congenital folate malabsorption, a rare condition believed to be caused by a defect in the folate transporter system. Folate is concentrated in the nervous system, and the CSF concentrations of folate are higher than the serum concentrations. If left untreated, a slowly progressive encephalopathy results, with intractable seizures. Other clinical and laboratory features include a female predominance, megaloblastic anemia, mouth ulceration, diarrhea, and failure to thrive. Neuroimaging studies reveal calcifications in the occipital lobes and basal ganglia (76). Treatment with high-dose oral or intravenous folate, in conjunction with methionine and vitamin B12, has been successful for seizure control (77).
Inherited defects of vitamin B12 metabolism also may produce severe megaloblastic anemia in early infancy. Generalized tonic convulsions almost invariably occur and are refractory to conventional treatment. Methylmalonic aciduria without homocystinuria suggests this diagnosis.
Inborn Errors of Creatine Metabolism
Disorders of creatine metabolism have been identified at two points in the metabolic pathway. The first defect involves the biosynthesis of creatine and is associated with either a deficiency in guanidinoacetate N-methyltransferase deficiency (19p13.3) or in arginine:glycine amidinotransferase. The second defect occurs at the level of the creatine transporter 1. These disorders result in a depletion of brain creatine. Guanidinoacetate N-methyltransferase deficiency has a more severe phenotype and has been associated with medically refractory epilepsy (78). Normal development is followed by a developmental regression between 3 months and 2 years of age. Seizures present in the first months of life with generalized tonic-clonic, astatic, absence, myoclonic, or partial seizures. Multifocal epileptiform discharges have been reported on the electroencephalograms of affected individuals (79). Other clinical features may include dystonia, dyskinesias, microcephaly, and autistic behaviors. Supplementation with creatine monohydrate (350 mg/kg per day to 2 g/kg per day) has led to improvement in affected individuals (79). Restriction of arginine and supplementation of ornithine has been used to reduce guanidinoacetic acid accumulation (80). Diagnosis can be made by magnetic resonance spectroscopy (MRS), which demonstrates reduced brain creatine.
Glut-1 Deficiency Syndrome
The Glut-1 deficiency syndrome (Glut-1 DS), previously referred to as glucose transporter protein deficiency syndrome, was first described in 1991 (81,82). The condition results from a loss of functional glucose transporters, encoded by the GLUT-1 gene, that mediate glucose transport across the blood-brain barrier. Clinical features include developmental delay, ataxia, hypotonia, infantile seizures, and acquired microcephaly. A reduction in the CSF-to-blood glucose ratio to half of normal (typically, CSF glucose <40 mg/dL) and a low lactate concentration are diagnostic. Additional confirmation of impaired glucose transport can be performed through assays in erythrocytes (83). The GLUT-1 gene was initially mapped to the short arm of chromosome 1 (1p31.3-p35) and more recently to 1p34.2 (84). A variety of mutations in the GLUT-1 gene have been described (85,86). Families with an autosomal dominant inheritance pattern have been identified (87,88). Seizures are often the first identified feature of this syndrome. Typical seizure types include absence, myoclonic, astatic, generalized tonic-clonic, and partial seizures. A normal electroencephalogram is commonly seen between seizures, although generalized 2.5- to 4-Hz spike-wave discharges are observed in more than one-third of children older than 2 years of age (89). Affected individuals without the classic clinical features have been identified and a screening lumbar puncture should be considered in those with refractory epilepsy (87). Seizures tend to be refractory to AEDs. Initiation of the ketogenic diet is effective in the treatment of seizures as well as of the overall disease process, as it provides an alternative cerebral energy source.
Organic Acidurias
Seizures in early infancy may be the presenting symptom of branched-chain organic acidurias. These include isovaleric aciduria, 3-methylcrotonyl-CoA carboxylase deficiency, 3-methylglutaconic aciduria with normal 3-methylglutaconyl-CoA hydratase, and 3-hydroxy-3-methylglutaric aciduria. Seizures including convulsions and infantile spasms tend to be prominent in 3-methylcrotonyl-CoA carboxylase deficiency. The gene locus is at 3q25-q27. The typical abnormal organic acids include 3-hydroxyisovaleric acid and 3-methylcrotonylglycine. Serum concentrations of free carnitine are low. A biotin-responsive form has been identified (90).
Severe developmental delay, progressive encephalopathy, and seizures are features of 3-methylglutaconic aciduria with normal 3-methylglutaconyl-CoA hydratase (91). This disorder results from a mutation on chromosome 9 in the gene encoding the enzyme 3-methylglutaconyl-CoA hydratase. Seizures occur in one-third of cases, and infantile spasms have been reported early in the course of the disorder. The typical organic acid abnormality includes marked elevations in 3-methylglutaconic acid and 3-methylglutaric acid in the urine.
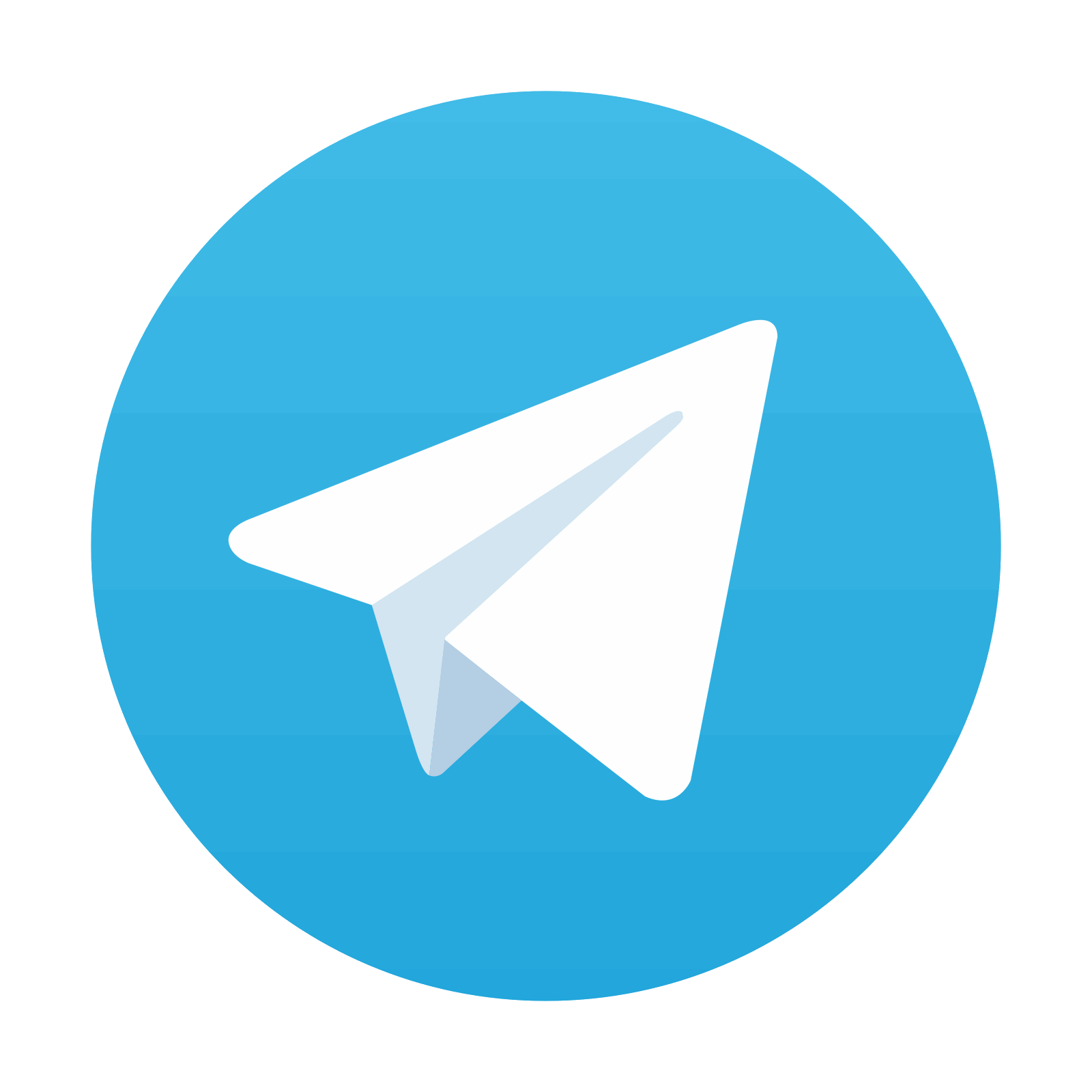
Stay updated, free articles. Join our Telegram channel

Full access? Get Clinical Tree
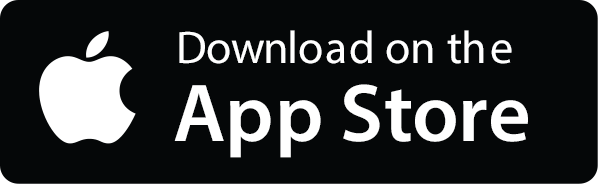
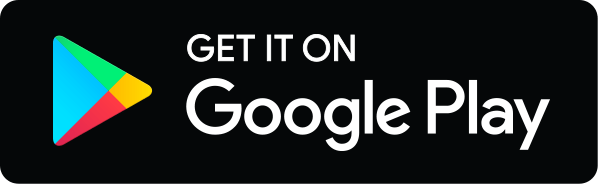