Epilepsy Surgery in Focal Malformations of Cortical Development
Jorge A. González-Martánez
Imad M. Najm
William E. Bingaman
Paul Ruggieri
Focal malformations caused by abnormal cortical development (MCD) are the pathologic substrates in an increasing number of patients with chronic epilepsy (1,2) and are even more common in children referred for surgical treatment (3,4). Among the histopathologic abnormalities included under this rubric are abnormalities of the architectural organization (laminar and columnar disorganization) of the cortex and the presence of dysmorphic (dysplastic) neurons, large neurons (meganeurons), and balloon cells (Fig. 80.1). Focal MCD may be restricted to the cortical mantle or may encompass the subcortical white matter and periventricular regions. As the sensitivity of neuroimaging techniques increases, even more patients with “cryptogenic” epilepsy will be found to have subtle alterations in cortical architecture (1,5,6) that may extend well beyond the abnormalities now visible on magnetic resonance imaging (MRI) scans (7). In surgical series, focal MCD tend to carry an unfavorable prognosis for seizure-free outcome (4,8).
As resective surgery depends on accurate preoperative localization of the epileptogenic zone, various clinical, electroencephalographic (EEG), anatomic, functional, metabolic, and neuropsychological techniques are enlisted to elucidate the pathologic cause and the relationships between the ictal onset zone and eloquent (functional) areas of the brain. Epilepsy surgery aims for the complete resection (or disconnection) of the cortical and subcortical areas responsible for the generation of seizures (epileptogenic zone) (9,10). Some epileptogenic areas may overlap with functional (eloquent) cortex, which must be preserved. An array of noninvasive and invasive diagnostic tools are available: analysis of seizure semiology, video-scalp electroencephalographic recordings, invasive subdural electrocorticographic (ECoG) recordings (intraoperative or extraoperative), extraoperative depth electrode recordings (stereo-encephalography [SEEG]), magnetoencephalography (MEG), and MRI. Neuroimaging techniques may provide functional (ictal single-photon-emission computed tomography [fSPECT] and fMRI), as well as metabolic (magnetic resonance spectroscopy [MRS] and positron emission tomography [PET]) information. These methods are usually complementary and will define cortical zones of interest: symptomatogenic, irritative, ictal onset, and functional deficit in addition to eloquent cortex and the epileptogenic lesion (for a detailed review, see refs. 9 and 10).
This chapter discusses methods to confirm the diagnosis of epilepsy in focal MCD; techniques to identify of the epileptogenic lesion; the role of subdural electrodes in localizing the epileptogenic zone and mapping the functional cortex and its relationships with the anatomic lesion; and surgical strategies and postresective seizure outcome.
DIAGNOSIS OF FOCAL EPILEPSY
Prolonged video-scalp EEG monitoring, analysis of clinical semiology, remains the gold standard for diagnosis of focal epilepsy (10). This noninvasive sampling technique gives an excellent overview of the location and extent of the epileptogenic areas but only approximates the boundaries of both the irritative and epileptogenic zones. Scalp electroencephalography detects only epileptiform activity
that results from EEG synchronization of large areas of cortex, estimated in some studies to be between 6 and 8 cm2 (11), and recordings are disturbed by the smearing effect of bone and other high-resistance structures (e.g., meninges and scalp) between the cortical generators and the recording electrodes (12, 13, 14). MEG may overcome some of these problems (11). Between 85% and 100% of patients with MCD exhibit epileptiform discharges on interictal scalp EEG recordings (15,16), ranging from lobar to lateralized, nonlocalizing to diffuse (including generalized spike-wave patterns in some cases of subependymal heterotopia [16]). The spatial distribution of interictal spikes is usually more extensive than the structural abnormality as assessed by intraoperative inspection or visual analysis of MRI findings (16, 17, 18, 19).
that results from EEG synchronization of large areas of cortex, estimated in some studies to be between 6 and 8 cm2 (11), and recordings are disturbed by the smearing effect of bone and other high-resistance structures (e.g., meninges and scalp) between the cortical generators and the recording electrodes (12, 13, 14). MEG may overcome some of these problems (11). Between 85% and 100% of patients with MCD exhibit epileptiform discharges on interictal scalp EEG recordings (15,16), ranging from lobar to lateralized, nonlocalizing to diffuse (including generalized spike-wave patterns in some cases of subependymal heterotopia [16]). The spatial distribution of interictal spikes is usually more extensive than the structural abnormality as assessed by intraoperative inspection or visual analysis of MRI findings (16, 17, 18, 19).
IDENTIFICATION OF THE ANATOMIC (POTENTIALLY EPILEPTOGENIC) LESION
MRI anatomic and signal abnormalities have been described in MCD (Fig. 80.2). An abnormal gyral anatomy or increased cortical thickness, or both, is common in some types of MCD (17,18). The underlying white matter is thin, with an increased signal on T2-weighted images. Other cases show variable changes that include short and indistinct white-matter digitations with asymmetric cortical thickening. Variable degrees of white-matter signal abnormalities may be evident on T2-weighted (or fluid attenuated inversion recovery [FLAIR]) images (20). The combination of overt gyral abnormalities and signal changes make the diagnosis of some dysplastic lesions obvious; however, subtle unilateral focal cortical abnormalities that are difficult to assess on conventional T1-weighted two-dimensional images may be the only finding. Despite significant MRI abnormalities in most cases of MCD, up to 25% of patients with “normal” MRIs had histopathologic changes in focal lesions (21). Milder forms of MCD characterized by cortical laminar and columnar disorganization in the absence of balloon cells may not be seen on high-resolution MRI (22). A recent review of patients treated surgically at the Cleveland Clinic between 1991 and 2001 noted normal results on visual analysis of high-resolution MRI studies in approximately 20% of patients with pathologically confirmed MCD (P. Widdess-Walsh and I. Najm, unpublished data, 2002).
MRI-based three-dimensional volume reconstructions have been used in an attempt to uncover sulcal or gyral abnormalities that may have gone undetected on visual analyses of the two-dimensional images (23, 24, 25, 26, 27). The utility of these postprocessing techniques has yet to be validated
through careful outcome analyses and direct ECoG and histopathologic correlations. Novel magnetic resonance sequences such as diffusion tensor imaging (28) and magnetization transfer neuroimaging (29) may demonstrate abnormalities not visualized on conventional MRI, but as yet clinical experience with these methods is limited.
through careful outcome analyses and direct ECoG and histopathologic correlations. Novel magnetic resonance sequences such as diffusion tensor imaging (28) and magnetization transfer neuroimaging (29) may demonstrate abnormalities not visualized on conventional MRI, but as yet clinical experience with these methods is limited.
![]() Figure 80.3 A: Intraoperative coregistration of subdural grids and depth electrodes on magnetic resonance imaging-based three-dimensional reconstruction of the brain. B: Corresponding intraoperative view of subdural grids and depths electrodes in their final position in the same patient. Frontal and temporal lobes and sylvian fissures are indicated. Please see color insert. |
A sizable number of patients with MCD do not achieve a good outcome even after “complete” resection of the lesion. Of the adult patients operated on at the Cleveland Clinic between 1991 and 2001, 57% were seizure free at least 6 months after surgical resection (compared with 78% who underwent surgery for drug-resistant temporal lobe epilepsy caused by hippocampal sclerosis) (P. Widdess-Walsh and I. Najm, unpublished data, 2002). These results suggest that current imaging (and evaluation) techniques do not accurately map the extent of dysplastic and epileptogenic regions in MCD. In other words, the topographic and functional relationships between MRI-revealed focal MCD and the epileptogenic zone are far from clear and unequivocal: neither all focal MCD nor all components of a focal MCD are necessarily epileptogenic. For these reasons, additional techniques that directly record in situ epileptogenicity from the cortex and map the exact extent of the abnormalities, such as the placement of subdural grids or depth electrodes and prolonged extraoperative recordings, may be needed.
Subdural Electrodes (Strips and Grids)
Subdural electrodes (stainless steel or magnetic resonancecompatible platinum) electrodes, embedded in strips or sheets of polyurethane or other synthetic material, are implanted over the suspected epileptogenic or functional dysplastic regions (30, 31, 32, 33, 34, 35). Subdural grids are inserted through either open craniotomy incisions or burrholes and registered stereotactically for extraoperative mapping (Fig. 80.3). The cortical covering may extend beyond the visualized cortical area, as grids may be slid over the edges of the craniotomy to cover adjacent areas for better ECoG or functional sampling. Besides the ECoG recordings and direct electrical stimulation studies, grids can be used to record somatosensory evoked potentials after stimulation of the trigeminal (lip) or median nerves for central sulcus localization.
Chronic extraoperative subdural techniques can record cortical ictal events and determine the functionality of the underlying cortex. Additionally, these recordings are performed on a relaxed, cooperative patient in the controlled environment of the epilepsy monitoring unit without the time constraints of the operating room.
Because foreign bodies are inserted into the cranial vault, the risks of the procedure include wound infection, flap osteomyelitis, acute meningitis, cerebral edema, and hemorrhage (36,37). Concerns about increased intracranial pressure may reduce the maximal number of electrodes that can be inserted and therefore produce incomplete epileptic mapping from large cortical areas. Other limitations may include the anatomic location of the proposed area of sampling (e.g., mesial orbitofrontal) and “re-do” surgeries with cortical adhesions. Where surgical access is difficult, as in the mesial surface of the hemispheres or in deep regions of the brain, SEEG, alone or combined with subdural grids, allows adequate coverage and precise targeting of the desired areas.
Implantation of Invasive Electrodes
When noninvasive studies produce nonconcordant or inconclusive information on the localization and extent of the seizure onset zone or the eloquent cortex, invasive studies involving subdural grids or depth electrodes may be needed (9,38,39). Jayakar (38) proposed the following relative indications for the use of subdural grids: normal
results on structural imaging, extratemporal location, divergent noninvasive data, encroachment on eloquent cortex, tuberous sclerosis, and cortical dysplasia. Rosenow and Lüders (10) recommended subdural electrodes for patients with focal epilepsy (single focus) only when there is a reasonable hypothesis on the location of the epileptogenic zone (derived from noninvasive studies).
results on structural imaging, extratemporal location, divergent noninvasive data, encroachment on eloquent cortex, tuberous sclerosis, and cortical dysplasia. Rosenow and Lüders (10) recommended subdural electrodes for patients with focal epilepsy (single focus) only when there is a reasonable hypothesis on the location of the epileptogenic zone (derived from noninvasive studies).
In patients with focal MCD, invasive electrode recordings are used to define the epileptogenic region and map eloquent areas.
Definition of the Epileptogenic Zone
In most cases of focal MCD, data generated from noninvasive EEG recordings and other electrophysiologic/neuroimaging techniques are sufficient to approximate the location of the epileptogenic zone.
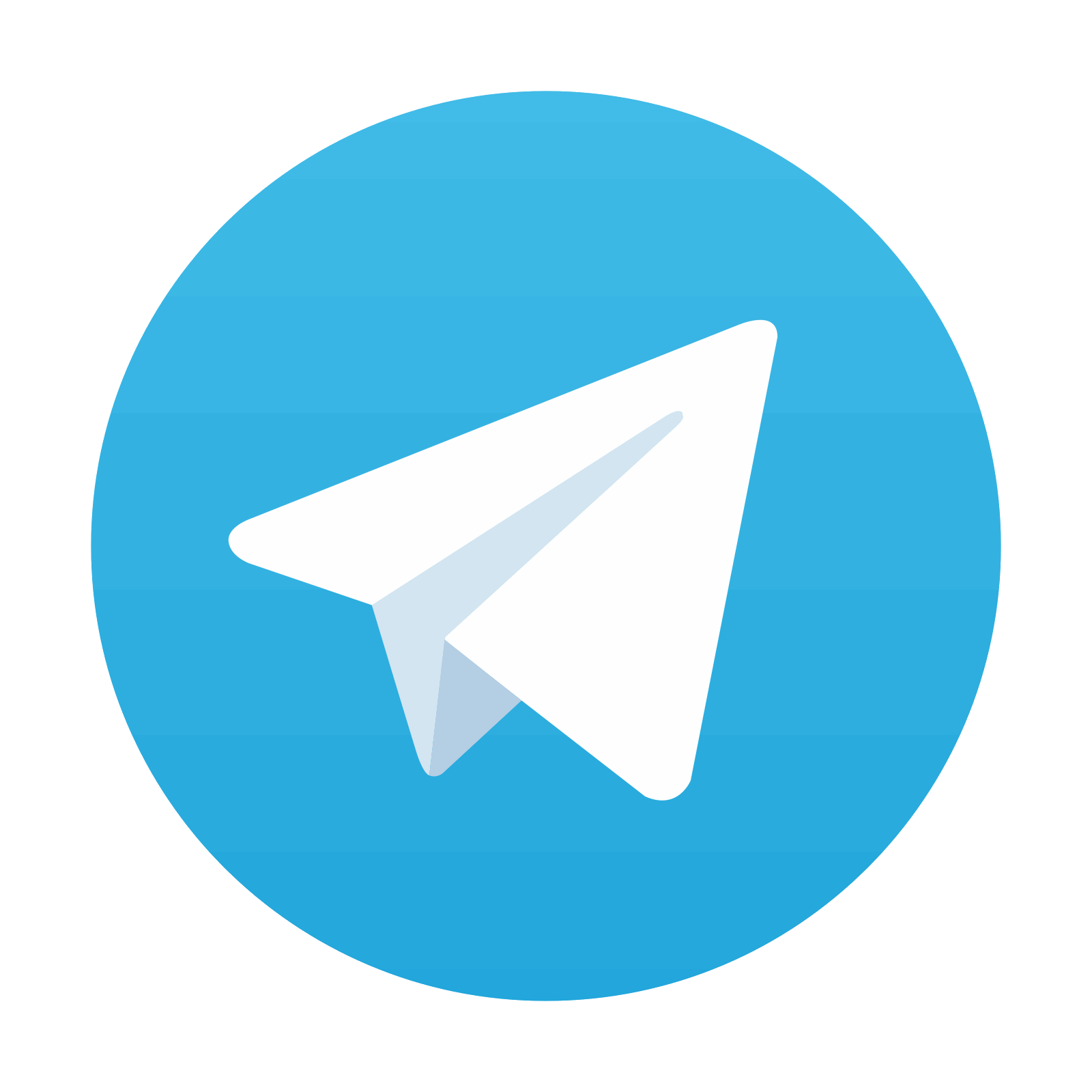
Stay updated, free articles. Join our Telegram channel

Full access? Get Clinical Tree
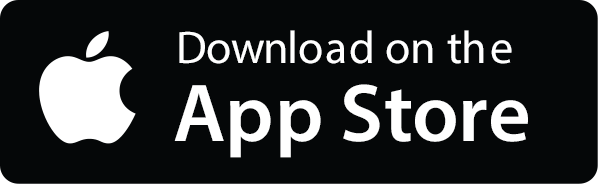
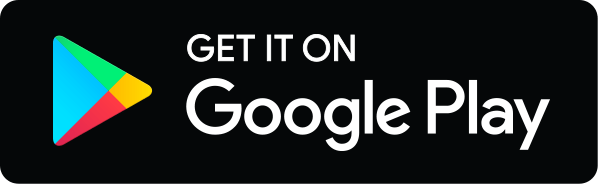