Epilepsy Surgery in the Absence of a Lesion on Magnetic Resonance Imaging
Elson L. So
Magnetic resonance imaging (MRI) is a prerequisite in the evaluation of patients for epilepsy surgery. It is well known that epilepsy surgery involving a focal MRI lesion generally confers the best prognosis for postsurgical seizure control (1,2). Complete resection of the lesion is associated with the probability of excellent postsurgical outcome that ranges from 70% to 90%, depending on the location and nature of the MRI lesion. In fact, patients with an MRI lesion that has concordant interictal and ictal electroencephalographic (EEG) discharges are the most privileged epilepsy surgery candidates, as they have approximately a 95% probability of successful seizure control following surgery (3,4).
In the absence of an MRI lesion, evaluation for epilepsy surgery becomes more challenging and complex. The location of the surgical focus and the extent of its resection are not as apparent in patients with nonlesional epilepsy as they are in those with lesional epilepsy. Additionally, the prognosis for postsurgical seizure control is not as favorable in patients with nonlesional epilepsy as it is in those with lesional epilepsy. This chapter discusses the challenges involved in epilepsy surgery evaluation when no lesion is present on MRI, and will describe a diagnostic approach and surgical strategy for optimizing the outcome of resective surgery in patients with nonlesional intractable focal epilepsy.
DEFINING MAGNETIC RESONANCE IMAGING-NEGATIVE EPILEPSY (NONLESIONAL EPILEPSY)
In the absence of an MRI lesion, epilepsy is referred to as nonlesional epilepsy. The term nonlesional epilepsy is sometimes used only when histologic examination of resected brain tissue does not reveal a well-recognized pathologic abnormality, such as a tumor or vascular malformation. Because this chapter discusses presurgical diagnosis and surgical strategy with a negative MRI, nonlesional epilepsy refers to the absence of a potentially epileptogenic lesion on MRI, regardless of what the postsurgical histopathologic examination may eventually reveal. The diagnostic and therapeutic issues covered in this chapter relate to resective surgery in patients with intractable focal epilepsy.
Before MRI is said to be “negative” and epilepsy is considered “nonlesional,” it is necessary to verify that the MRI was performed with techniques that optimize the ability to detect potentially epileptogenic lesions (5,6). Standard MRI studies are insufficient for the evaluation of patients for epilepsy surgery. Sensitivity in detecting a lesion was only 50% with expert review of standard MRI studies, but it increased to 91% with expert review of epilepsy-protocol MRI studies of the same patients (7). Specific MRI imaging techniques and sequences must be used to minimize the
risk of missing an epileptogenic lesion that would have facilitated presurgical localization and subsequent resection of the seizure focus. The requisite imaging techniques and sequences are described in the literature (5) and are affirmed by the Commission on Neuroimaging of the International League Against Epilepsy (8). The basic requirements are that (a) field strength of the MRI magnet must be 1.5 tesla or greater; (b) image slice thickness should be 1.5 mm or thinner, with no interslice gap; (c) images should be displayed in coronal, axial, and sagittal views; (d) T1-weighted, T2-weighted, and fluid attenuated inversion recovery (FLAIR) images should be obtained; (e) temporal lobe images should be obtained at oblique coronal planes that are perpendicular to the long axis of the hippocampal formation; and (f) quantitative volumetric measurement of the amygdala-hippocampal complex should be available.
risk of missing an epileptogenic lesion that would have facilitated presurgical localization and subsequent resection of the seizure focus. The requisite imaging techniques and sequences are described in the literature (5) and are affirmed by the Commission on Neuroimaging of the International League Against Epilepsy (8). The basic requirements are that (a) field strength of the MRI magnet must be 1.5 tesla or greater; (b) image slice thickness should be 1.5 mm or thinner, with no interslice gap; (c) images should be displayed in coronal, axial, and sagittal views; (d) T1-weighted, T2-weighted, and fluid attenuated inversion recovery (FLAIR) images should be obtained; (e) temporal lobe images should be obtained at oblique coronal planes that are perpendicular to the long axis of the hippocampal formation; and (f) quantitative volumetric measurement of the amygdala-hippocampal complex should be available.
Ideally, three-dimensional rendition of the brain surface must be possible when needed, along with the capability for accurate coregistration of images from other diagnostic procedures. Finally, physicians who review the MRI images must be highly skilled in detecting and interpreting the structural alterations associated with epileptic seizure disorders.
Despite absence of an MRI lesion, pathologic examination of cerebral tissue removed during surgery for nonlesional intractable epilepsy invariably reveals abnormal findings (9). Nonspecific gliosis, the most common finding, consists of varying degrees of glial proliferation and reduced neuronal population, or altered neuronal morphology (10). Varying degrees of malformation of cortical development are also observed, ranging from typical cortical dysplasia (11) to microdysgenesis. Microdysgenesis consists of abnormal clustering of neurons in amount or location, or minute cortical/subcortical foci of disorganized mature but nonneoplastic elements of neuronal and glial origins (glioneuronal hamartia) (12). Occult tumors and vascular malformations are reported to be present in 15% of the nonlesional epilepsy surgical specimens (9). Furthermore, nonlesional temporal lobe epilepsy is often considered putatively as lateral neocortical in seizure origin, but such an assumption should be made with caution because pathologic evidence of mesial temporal sclerosis is encountered in some patients whose MRI shows no amygdala-hippocampal atrophic or signal changes (13,14).
Regardless of the histologic basis of nonlesional epilepsy, the ultimate relevance of focal nonlesional epilepsy surgery is seizure control leading to satisfactory quality of life. Such a definitive outcome should be the gold standard for determining the accuracy of preoperative diagnostic tests in delineating the epileptogenic zone. Unfortunately, most published reports do not use this standard, relying instead on extracranial or intracranial ictal EEG-onset zone or focus (ictal onset zone) as the standard for comparison.
IMPLICATIONS OF NONLESIONAL INTRACTABLE EPILEPSY
The absence of MRI lesion is not uncommon in patients who undergo evaluation for intractable epilepsy. The proportion of intractable focal epilepsy patients with nonlesional MRI varies between centers, but the rate has been estimated at 10% to 15% of surgical series (9). Much of the variation in the rates reported is determined by the type of patients evaluated and the MRI techniques used. In the absence of a potentially epileptogenic lesion on MRI, localization of the site for epilepsy surgery is more complex, surgical resection is more difficult, and postoperative outcome is generally less favorable than that in lesional epilepsy surgery.
Limitations of Noninvasive Presurgical Evaluation in Patients with Non-lesional Epilepsy
Although the importance of a complete and accurate medical history in the evaluation of epilepsy cannot be overemphasized, seizure description provided by witnesses can be inadequate or inaccurate for the purpose of localizing seizure onset. A prospective study involving patients’ close friends and relatives demonstrated that their median accuracy for recalling features of convulsive seizures that they had observed was only 45%, compared with 70% for nonconvulsive seizures (15). Therefore, seizure semiology in each patient must be confirmed by analysis of video recordings of habitual seizures. Although several clinical manifestations of seizures correctly lateralize seizure onset to a hemisphere in 80% to 90% of patients (16), semiologic features are not as helpful in localizing seizure onset to a specific area or region within the hemisphere (17).
MRI and extracranial electroencephalography are the two major laboratory tests used in the initial phase of presurgical evaluation of candidates for epilepsy surgery. When the MRI is negative, greater reliance is placed on extracranial electroencephalography to determine the location of the epileptogenic zone (i.e., the focus that, when resected, results in seizure control). Both interictal and ictal extracranial EEG discharges have been valuable in identifying candidates who may benefit from epilepsy surgery, especially from anterior temporal lobectomy (4,18, 19, 20). In general, the location of the interictal epileptiform discharges (IEDs) on the scalp suggests the location of the seizure focus on the brain (21), but the association between the two varies with different IED locations (22). The association is best with temporal lobe IED. Caution should still be exercised when imputing temporal lobe IED to temporal lobe epilepsy, because temporal lobe location of extracranial IEDs is encountered in patients with frontal lobe, parietal lobe, or occipital lobe epilepsy (23, 24, 25).
In temporal lobectomy candidates, the IED location on the scalp and the presence of an MRI lesion are independently
and equally predictive of postsurgical seizure control (4). The presence of temporal lobe IEDs that are all concordant with temporal lobe ictal discharges is associated with a three to four times greater probability of excellent postsurgical seizure control than when IEDs are absent or nonconcordant. This odds ratio is similar to that between the presence and the absence of a focal MRI lesion. Moreover, in patients with lesional epilepsy, exclusively concordant scalp IEDs improve the surgical prognosis beyond that conferred by the presence of an MRI lesion. The rate of excellent postsurgical outcome in these patients is 94% when exclusively concordant IEDs are present versus 60% when they are absent. Conversely, the presence of exclusively concordant IEDs is not associated with a better prognosis for seizure control in patients undergoing nonlesional temporal lobe surgery. The probability of excellent postsurgical outcome is approximately 60% to 65%, regardless of the presence or absence of concordant IEDs in patients with nonlesional epilepsy.
and equally predictive of postsurgical seizure control (4). The presence of temporal lobe IEDs that are all concordant with temporal lobe ictal discharges is associated with a three to four times greater probability of excellent postsurgical seizure control than when IEDs are absent or nonconcordant. This odds ratio is similar to that between the presence and the absence of a focal MRI lesion. Moreover, in patients with lesional epilepsy, exclusively concordant scalp IEDs improve the surgical prognosis beyond that conferred by the presence of an MRI lesion. The rate of excellent postsurgical outcome in these patients is 94% when exclusively concordant IEDs are present versus 60% when they are absent. Conversely, the presence of exclusively concordant IEDs is not associated with a better prognosis for seizure control in patients undergoing nonlesional temporal lobe surgery. The probability of excellent postsurgical outcome is approximately 60% to 65%, regardless of the presence or absence of concordant IEDs in patients with nonlesional epilepsy.
IEDs are often absent or widespread in patients with frontal lobe epilepsy (26). In one study, nearly 20% of patients with frontal lobe epilepsy do not have scalp-recorded IEDs and, when present, IEDs were discordant with the frontal epileptogenic zone in 45% of patients (25). In patients undergoing frontal lobe surgery, the value of extracranial IED in localizing the epileptogenic zone and in predicting the postsurgical outcome is limited. The presence and location of scalp-recorded IED are not independently associated with the outcome of frontal lobe epilepsy surgery, which is the most common type of extratemporal epilepsy surgery (18).
Extracranial ictal EEG has certain inherent limitations, even in those with intractable epilepsy whose MRI had revealed a potentially epileptogenic lesion. Between 25% and 30% of all seizures recorded in patients with unilateral mesial temporal lobe epilepsy (27) or MRI-detected hippocampal atrophy (19) could not be lateralized to the diseased temporal lobe. In addition, a similar proportion of seizures could not be lateralized in patients who underwent successful temporal lobectomy (28). As many as 60% of extracranially recorded seizures in temporal lobectomy candidates had no localizing features at seizure onset (29). Of temporal lobectomy candidates, 10% had extracranial seizures with conflicting features (30) and 18% had falsely localizing seizures (31). The situation is no better in patients with extratemporal epilepsy. Approximately 35% to 50% of seizures recorded extracranially in extratemporal epilepsy are nonlateralizing (28). Epileptogenic foci in the mesial frontal region are frequently devoid of detectable IEDs or ictal discharges on the scalp (32,33). In one study, false lateralization or localization occurred in 28% of occipital and 16% of parietal seizures (33). Moreover, scalp-recorded seizure onset may not be obvious at extratemporal epileptogenic sites, but ictal discharges may appear instead at a temporal region to which it has spread (34, 35, 36).
In many of the above situations, invasive ictal electroencephalogram recordings can still be obviated when MRI shows a relevant lesion and other concordant clinical or interictal EEG features are also present (37). However, when MRI shows no lesion, insufficiently localizing EEG seizures complicate the task of delineating the epileptogenic focus for surgical resection. Furthermore, the finding of temporal lobe seizure onset on scalp electroencephalogram recordings cannot be relied on solely for localization of the ictal focus in patients with nonlesional epilepsy. In one study, 11 of 33 intractable epilepsy patients with negative MRIs had proven extratemporal seizure onset despite apparent onset of scalp-recorded EEG seizures at the temporal lobe region (38).
Complexity of Invasive Electroencephalogram Recordings and Surgical Resection in Patients with Nonlesional Epilepsy
In the absence of an MRI lesion, the adequacy of the extent of intracranial electrode implantation or surgical resection is not as apparent as when a lesion is present. The situation often calls for extensive intracranial electrode implantation over large regions in one or both hemispheres. Unfortunately, serious neurologic complications have been reported in 4% to 5% of patients with depth electrode implantation (39) and in as many as 20% of those with subdural electrode implantation (40). The number of intracranial electrodes required for sufficient coverage is difficult to determine when there is no structural abnormality on MRI. It is not unusual that additional electrodes need to be implanted when initial implantation yields insufficient information. Unfortunately, the risk for complications rises with the extent of intracranial electrode implantation. It is estimated that the risk for major complications increases by 40% for every 20 additional subdural electrodes implanted, with the risk as high as 82% with bilateral hemisphere implantations (41).
In lesional epilepsy surgery, surgical resection is guided mainly by the location and extent of the cerebral structural abnormality and its associated EEG abnormalities. In many patients, surgical resection that is restricted to the MRI lesion and its immediate surrounding tissues (lesionectomy) is sufficient for achieving seizure control (42). One study showed that the probability of seizure control is high when the MRI lesion is resected, but seizure outcome is poor when the resection involves mainly the abnormal electroencephalogram focus, not the MRI lesion (43).
In nonlesional epilepsy surgery, clinicians and surgeons are deprived of neuroanatomic landmarks to guide the extent of resection. In such cases, resection is then based on the extent of electroencephalogram abnormalities, but extensive resection based on abnormal EEG discharges raises the risk of perioperative morbidity. Conversely, restricted resection that spares electrophysiologically abnormal tissues may reduce the probability of postsurgical seizure control, especially in patients with extratemporal neocortical epilepsy (44).
Less Favorable Prognosis in Patients with Nonlesional Epilepsy Surgery
It has been known for some time that a remarkable discrepancy exists between the outcomes of lesional and nonlesional surgeries in patients with intractable focal epilepsy. The probability of excellent postsurgical outcome following nonlesional surgery is uniformly lower across many studies in the literature (45). In one series of 157 consecutive patients who underwent anterior temporal lobotomy, 62% of those with no MRI lesion versus 85% of those with an MRI lesion had an excellent outcome (i.e., no seizures, auras only, or rare nocturnal seizures) (4). The outcome in patients undergoing nonlesional frontal lobe surgery is even less favorable. In a study of 68 patients who underwent frontal lobe epilepsy surgery, only 40% of those without an MRI lesion had an excellent postsurgical outcome, whereas 72% of those with an MRI lesion experienced an excellent outcome (18). Moreover, the value of the intracranial electroencephalogram recording is limited in patients whose MRI detects no lesions. Only 22% of such patients became seizure free following epilepsy surgery, despite the presurgical localization of seizure onset by the intracranial electroencephalogram recording (46).
DIAGNOSTIC APPROACH IN NONLESIONAL EPILEPSY SURGERY
When MRI detects no lesion in patients undergoing evaluation for epilepsy surgery, the surgical focus has to be determined by detecting the abnormal functions associated with epileptic seizure activities or with epileptogenic properties. Abnormal functions of the seizure focus can be detected clinically by observing the seizure semiology, electrographically by analyzing the electroencephalogram recording, and topographically by assessing functional imaging results. Intracranial electrode implantation is required in many patients with nonlesional intractable epilepsy. In the absence of a structural lesion on MRI, functional imaging becomes essential for guiding intracranial electrode implantation and for limiting the extent of the implantation. In some cases, functional imaging results can obviate the need for intracranial electrode implantation. Functional imaging tests that are commonly used in epilepsy surgery evaluation include positron emission tomography (PET), single-photon-emission computed tomography (SPECT), magnetic resonance spectroscopy (MRS), and magnetoencephalography (MEG). The following sections discuss the application of noninvasive tests that are useful in the evaluation of patients for nonlesional epilepsy surgery.
Video with Extracranial Electroencephalographic Recordings
Despite their limitations, seizure semiology and extracranial electroencephalography must still be fully explored for clues that help lateralize or localize seizure onset. Some seizure auras have localizing value (47). Auras involving the perirolandic or occipital regions are especially useful in localizing seizure onset (23,24). Seizure semiology adds lateralizing information to that obtained from extracranial ictal EEG recordings. Without semiology, seizure onset can be adequately lateralized by noninvasive EEG recordings in only 65% of temporal lobectomy candidates (48). When seizure semiology is added, seizure onset is adequately lateralized in almost 95% of the candidates (48).
Some types of extracranial IEDs and ictal discharges have value in determining the location of a seizure focus and in guiding intracranial electrode implantation. Exclusively unifocal IEDs are strong predictors of the site of the ictal onset zone in both lesional and nonlesional temporal and extratemporal epilepsy (4,49). Resection of an extratemporal focus that is the sole location of IEDs is associated with a 77% probability of seizure-free outcome, regardless of the presence or absence of an MRI lesion (49). In temporal lobe epilepsy, the preponderance of scalp or sphenoidal IEDs at one temporal lobe strongly suggests that the ictal onset zone resides within that lobe. Ninety-five percent of the patients with a greater than 95% preponderance of IEDs at one anterior temporal region had intracranially recorded seizures originating at the same region (50).
It has been reported that epilepsies involving certain parts of the cerebral hemispheres have distinct EEG discharge patterns at ictal onset (33). For instance, a repetitive epileptiform pattern is more frequently encountered in seizures arising from the lateral frontal convexity, whereas a rhythmic temporal theta discharge pattern is exclusively seen in temporal lobe seizures. However, the majority of patients in whom these observations were made had MRI lesions, and it is not clear whether the findings are consistently observed in patients with nonlesional epilepsy. More recently, it was found that the presence of a fast discharge in the beta-frequency range at the onset of a frontal seizure is highly indicative of the location of the epileptogenic zone (20) (Figs. 82.1 and 82.2). Approximately 90% of patients with this focal ictal beta-discharge pattern at seizure onset became seizure free following resection of the frontal lobe focus, even when the MRI was negative. In comparison, postsurgical seizure freedom occurred in only 16.7% of nonlesional frontal lobe epilepsy patients who did not have a focal ictal beta-discharge pattern.
Positron Emission Tomography in Patients with Nonlesional Epilepsy
The most commonly used radioactive ligand in PET studies of patients with epilepsy is 2-[18F]fluoro-2-deoxy-D-glucose (FDG). Because of the short half-life and long cerebral uptake of FDG, FDG-PET studies are performed during the interictal period. The following discussion of FDG-PET findings refers to the well-known feature of interictally reduced
glucose uptake (i.e., hypometabolism) that is suggestive of an underlying potentially epileptogenic focus or region.
glucose uptake (i.e., hypometabolism) that is suggestive of an underlying potentially epileptogenic focus or region.
A review of the literature in 1994 found that approximately 70% of temporal lobe epilepsy patients have temporal lobe hypometabolic abnormalities on their interictal FDG-PETs (51). However, most patients in the studies reviewed had MRI lesions, and most PET images were assessed by visual inspection. Quantitative measurements of FDG uptake increase the sensitivity of PET studies in patients with focal epilepsy (52,53). Using quantitative measurements, a study of mostly temporal lobe epilepsy and some extratemporal lobe epilepsy patients demonstrated that the interictal hypometabolic zone was concordant with the intracranial ictal EEG onset zone in all patients (54). In another study, 75% of patients who had reduced lateral temporal FDG uptake of 15% or more, as compared with the other side, became seizure free after temporal lobectomy (52). This finding is particularly important because 67% of the patients in the study did not have a relevant MRI lesion. The study also showed that the finding of a hypometabolic temporal lobe is particularly useful when the extracranial ictal electroencephalogram is not localizing.
In contrast to the usefulness of interictal FDG-PET in patients with nonlesional temporal lobe epilepsy, the technique is not as useful in those with extratemporal lobe epilepsy. Whereas the yield of FDG-PET in lesional extratemporal epilepsy is modest (51), the yield declines when MRI is normal or when ictal EEG is indeterminate for seizure onset (55,56). Studies of FDG-PET in patients with nonlesional extratemporal epilepsy have generally reported a low yield. Only 9% of nonlesional extratemporal epilepsy patients have a localized FDG-PET abnormality (51). An exception was a study that reported that 85% of nonlesional frontal lobe epilepsy patients had a unilateral frontal hypometabolic region (57). However, the location of the hypometabolic region observed in that study
did not correspond to the ictal EEG onset zone in 20% of the patients.
did not correspond to the ictal EEG onset zone in 20% of the patients.
Caution must be exercised when using FDG-PET for localizing the focus for either temporal or extratemporal epilepsy surgery. In temporal lobe epilepsy, the hypometabolic PET defect frequently involves the lateral or inferior neocortical region, even in patients with mesial temporal sclerosis or with mesial temporal ictal onset (58). Furthermore, the abnormal FDG-PET focus is not confined to the temporal lobe in 30% of patients with proven mesial temporal lobe epilepsy. In these patients, the abnormal PET focus extends into the ipsilateral frontal lobe region (59). Similarly, the region of FDG-PET abnormality in patients with suspected frontal lobe epilepsy is often diffuse and poorly localized (55,60). In one study, the size of the hypometabolic region exceeded the ictal EEG onset zone in nearly 40% of patients with nonlesional frontal lobe epilepsy (57).
Despite the low yield of FDG-PET in extratemporal epilepsy, its clinical application is not limited to patients with suspected temporal lobe epilepsy. The clinical and EEG profiles, of many patients with MRI-negative intractable epilepsy are insufficient for classifying with confidence the epilepsy as either temporal or extratemporal, or for lateralizing with a certainty the seizure onset to one hemisphere. In those situations, the use of such functional imaging tests as PET should be considered as a means of detecting additional evidence for distinguishing between the two main types of focal epilepsy or for lateralizing seizure onset to one hemisphere. The PET abnormality is then used to guide the location and to limit the extent of intracranial electrode implantation. A wide region of PET abnormality that affects both temporal and extratemporal areas can be still be implanted with intracranial electrodes to detect a more discrete focus of ictal EEG onset. PET abnormalities confined to one hemisphere can obviate the need for bilateral hemispheric implantation of intracranial electrodes.
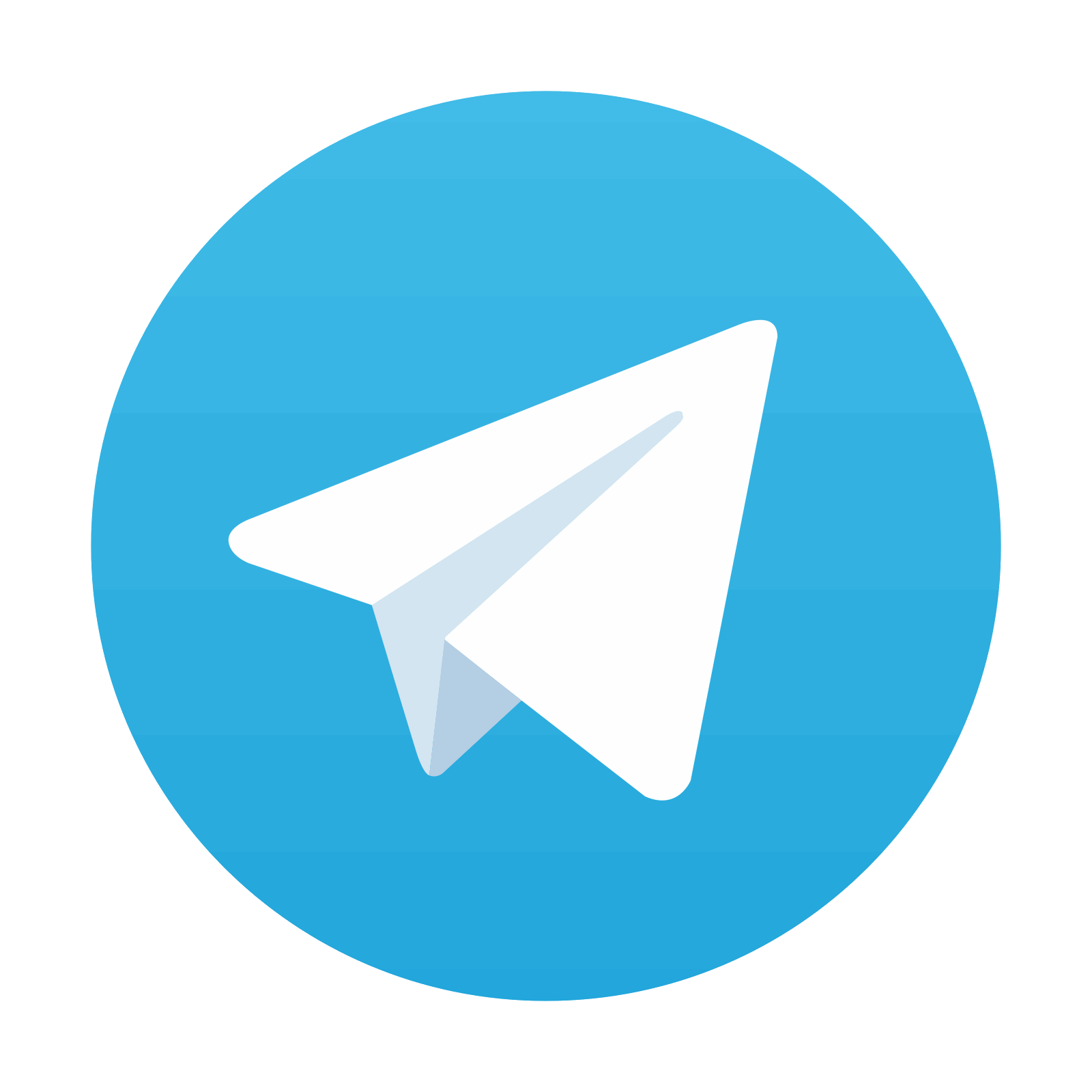
Stay updated, free articles. Join our Telegram channel

Full access? Get Clinical Tree
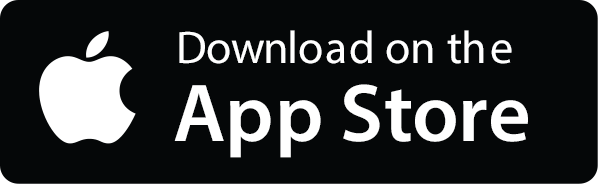
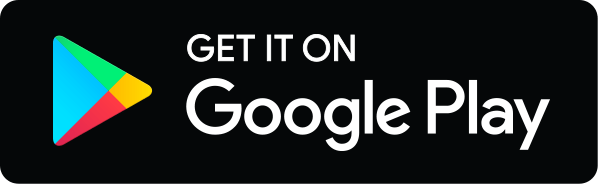