Introduction
Neuromonitoring can help determine the extent of neurologic injury, can detect secondary injury at a potentially reversible stage, can help assess the effect of clinical care on an individual patient, and may aid in predicting outcome. This can be particularly important in the neurocritical care unit (NCCU), where the neurologic examination may be difficult because of depressed consciousness, hypothermia, or use of sedatives. Somatosensory evoked potentials (SSEPs) have been used in clinical practice since they were first described in the late 1940s in patients with myoclonus, and after electroencephalography (EEG) are the most frequently used neurophysiologic tool in the NCCU. In general, SSEPs are less affected by sedation or hypothermia than EEG. Brainstem auditory evoked potentials (BAEPs) have been used since the 1960s to study noninvasively and objectively the function of the brainstem auditory pathways. Other tools such as motor evoked potentials and visual evoked potentials are less frequently used in the NCCU. Use of evoked potential (EP) monitoring in neurocritical care practice varies regionally and even between intensive care units (ICUs), and the ready availability of neuroimaging has limited the application of EPs in many ICUs. However, the diagnostic and prognostic value of EPs is embraced by national and international organizations. Advantages of EPs include: (1) they are noninvasive, (2) they may be used serially, (3) they give objective quantitative values that can be followed over time and compared between patients, (4) they are relatively stable in the presence of mild hypothermia and relatively resistant to many commonly used sedatives, and (5) they are inexpensive. In addition, modern invasive neuromonitoring and treatment devices such as intravascular cooling catheters can limit patient transport or use of magnetic resonance imaging (MRI). In the absence of portable imaging devices, diagnostic tools such as EPs that can be brought to the patient’s bedside provide an alternative diagnostic tool. The technical feasibility of continuous EP monitoring along with continuous EEG monitoring has been demonstrated.
Engineering and Technology
Somtatosensory Evoked Potentials
SSEP testing assesses the integrity of the dorsal column–lemniscal system. This pathway projects via the dorsal column of the spinal cord to the cuneate nucleus in the lower brainstem, to the ventroposterior lateral thalamus, to the primary somatosensory receiving cortex, and then to a wide network of cortical areas involved in somatosensory processing (secondary somatosensory cortex, posterior parietal cortex, posterior and mid-insula and mid-cingulate cortex). Median and tibial nerves are most often stimulated in SSEP testing, although others (e.g., ulnar, peroneal) may be used when appropriate.
Stimulation
The stimulus for SSEPs is a brief electrical pulse delivered by a pair of electrodes placed on the skin above the nerve. The median nerve is stimulated at the lateral-anterior wrist; the tibial nerve is stimulated at the ankle, with the cathode between the Achilles tendon and the medial malleolus. To reduce artifact produced by electrical stimulation, a ground electrode is placed between the stimulation site and the recording site. The stimulus is delivered as a constant monophasic square-wave pulse that lasts approximately 100 to 200 msec, at a rate of typically 4 per second. The intensity of the stimulation is two to four times the sensory threshold as evidenced by the contraction of the muscle innervated by the stimulated nerve.
Recording
Both standard surface disk electrodes and needle electrodes can be used. Recording electrodes for upper limb SSEPs are placed at the clavicle between the heads of the sternocleidomastoid muscles (Erb’s point) and on the skin overlying cervical bodies 6 to 7. On the scalp, electrodes are placed at CP3 and CP4 (respectively midway between C3 and C4) of the international 10-20 system. Bipolar recording between CP3 and CP4 detects the cortical component of the SSEP; referential recording, to earlobe or noncephalic locations (e.g., the shoulder), is used to detect subcortial (brainstem) components. For tibial SSEPs, electrodes are placed in the popliteal fossa (peripheral component) and over the lumbar vertebra (lumbar component). At least two bipolar channels (e.g., CPz-Fpz and CP3-CP4) (see Chapter 25 for a review of EEG montage nomenclature) should be used to record the cortical component; as with the median SSEPs, referential scalp recording is used to detect subcortical components. The SSEP waveform is obtained by averaging typically from 500 to 2000 stimuli. It is is necessary to repeat at least two independent averages to demonstrate reproducibility. For clinical purposes, analysis times of 50 msec (median nerve) or 100 msec (tibial nerve) are used.
Filters
SSEPs are recorded using a broad pass-band with high-pass and low-pass filters set typically to 30 Hz and 2000 Hz, respectively. Notch filters to eliminate power line noise (50 or 60 Hz) should be used with caution because of their tendency to create “ringing” oscillatory artifact. Digital postprocessing of the recorded signal may be appropriate in specific situations.
Brainstem Auditory Evoked Potentials
BAEPs are generated using brief auditory stimuli that activate the cochlea, the auditory nerve, and then the brainstem auditory pathways.
Stimulation
The stimulus used to generate a BAEP consists of a brief (less than 200 μsec) “click,” typically delived at a rate of approximately 10 Hz. The stimuli are presented to one ear at a time using headphones or ear inserts while the nonstimualted ear is “masked” with white hissing noise to prevent stimulation by sound conducted through the cranium.
Recording
Either standard surface electrodes or needle electrodes may be used. BAEPs are typically recorded using a two-channel montage: ipsilateral ear to vertex (channel 1) and contralateral ear to vertex (channel 2). Averaging of 2000 to 4000 stimuli is typically required; at least two independent averages must be recorded to prove reproducibility of waveforms.
Filters
BAEPs are recorded using a broad pass-band, with the high-pass filter typically set to 100 Hz or 150 Hz and the low-pass filter set to 3000 Hz.
Information Provided and What the Information Means
Somtatosensory Evoked Potentials
SSEP recordings consist of signals recorded from different sites along the afferent sensory pathways. By convention, negative singals are displayed as upward deflections, and positive signals downward. Physiologic signals are recorded with differential amplifiers, devices that amplify the difference between two inputs. A negative signal in the first, or “noninverting” input produces an upward inflection; a negative signal in the second, or “inverting” input produces a downward inflection. The time between stimulation and the recorded potential is called the latency , and is usually expressed in milliseconds. A normal median SSEP waveform is shown in Figure 24.1 . Generator sources for specific components of the SSEP were indentified initially based on the effects of clinical lesions and direct recording from intracranial structures; more recently, source modeling based on three-dimensional MRI has been used to revisit these generators.

Clinical interpretation is based primarily on the analysis of “short latency” SSEP signals, up to and including those corresponding to the activation of the somatosensory receiving cortex ( Table 24.1 ). The N9 is a compound action potential reflecting the peripheral afferent volley and is used as a reference point from which to calculate conduction time for the centrally recorded potentials. Peripheral neuropathy may increase the latency and decrease the amplitude of this waveform. N13 is generated by dorsal horn cells of the cervical spinal cord. P14 is generated in the caudal medial lemniscus within the lower medulla, with possible additional presynaptic contribution from the upper cervical cord. N18 is attributed to postsynaptic activity in multiple brainstem gray matter structures. N20 reflects activation of the primary somatosensory receiving area, located in the posterior bank of the rolandic fissure in Brodmann’s area 3b. Although actually measuring a composite of white and gray matter events in the large fiber sensory system between the cervicomedullary junction and somatosensory cortex, the P14 to N20 interpeak latency (calculated by subtracting the P14 peak latency from the N20 peak latency) is commonly referred to as the central conduction time (CCT). Absent or delayed central conduction with present peripheral conduction may permit lesions to be localized by SSEPs.
Recording Site | Latency, Mean (msec) | Latency, Upper Limit (msec) | |
---|---|---|---|
MEDIAN SSEP | |||
N9 | Erb’s point | 9.8 | 11.5 |
N13 | Cervical spine (C7) | 13.3 | 14.5 |
N20 | Contralateral cortex (CP3 or CP4) | 19.8 | 23.0 |
INTERVALS MEDIAN | |||
CCT (P14-N20) | — | 5.6 | 6.6 |
Tibial SSEP | |||
N8 | Popliteal fossa | 8.5 | 10.5 |
N22 | Lumbar spine (L1) | 21.8 | 25.2 |
P30 | Fz-Cv7 | 29.2 | 34.7 |
P39 | Cz-Fz | 38.0 | 43.9 |
INTERVALS TIBIAL | |||
N22-P30 | — | 7.4 | 10.2 |
P30-P39 | — | 8.7 | 13.4 |
Principal components of the tibial SSEP include the N8 recorded from the popliteal fossa, the N22 generated by the dorsal gray matter of the lumbosacral cord, the P30 generated at the cervicomedullary junction, and the P39 generated in the somatosensory cortex. In contrast to the median nerve SSEP, the P39 cortical signal is positive in polarity and variably maximal in amplitude either near the vertex or over the centroparietal scalp ipsilateral to the stimulated leg. This interindividual variability in the scalp topography of P39, due to the variations in the normal location of the leg and foot area within the interhemispheric fissure, necessitates use of at least two bipolar scalp-scalp channels, one in the midline and another using laterally placed electrodes, to ensure reliable detection.
Middle- and long-latency SSEP components are not commonly used clinically in the ICU because they are less reliable and more susceptible than short-latency SSEPs to the effects of medication and to depressed levels of consciousness.
Brainstem Auditory Evoked Potentials
Clinical interpretation of the BAEP is based on signals generated within approximately 7 milliseconds following the stimulus ( Fig. 24.2 ). Studies suggest that BAEPs have multiple generators, with nonsequential interaction between second- and third-order ipsilateral and contralateral neuronal cells and axons. Although the exact identity of short-latency BAEP waveforms remains somewhat uncertain, commonly recognized generators include the distal auditory nerve (wave I), the auditory nerve as it exits the porus acousticus or the cochlear nucleus (wave II), the cochlear nucleus or ipsilateral superior olivary nucleus (wave III), the superior olivary nucleus or axons of the lateral lemniscus (wave IV), and the inferior colliculus and ventral lateral lemniscus (wave V). Because waves II and IV are less reliably recorded across individuals, clinical interpretation is based primarily on assessment of waves I, III, and V (see Fig. 24.2 ).

BAEPs are resistant to the effects of sedative medication and to a patient’s level of consciousness depression, adding to their utility in the ICU setting. Although the amplitude and latency of these waves are affected substantially by click stimulus intensity, the I to V interpeak latency (as well as I to III and III to V interpeak latencies) is relatively unaffected by intensity changes and interpeak latency and is therefore relied on for clinical interpretion.
Delays in wave V with a normal wave I latency suggest a conduction abnormality somewhere central to the distal portion of cranial nerve VIII. Absence of wave I with preserved wave V could reflect a problem with the peripheral hearing apparatus or with the auditory nerve, but commonly reflects technical difficulty recording wave I. Functional disruption of the brainstem may cause loss of waves II to V with preservation of wave I. Unilaterally abnormal BAEPs most often reflect ipsilateral brainstem damage.
More rostral structures, including generators within the primary auditory cortex and surrounding areas, are responsible for longer latency components. However, as with SSEPs, the later components of the BAEP are affected by consciousness state and attention, and are less commonly used clinically in the ICU setting.
Indications for Use
In the ICU, EPs are primarily used for prognostication, particularly in patients after cardiac arrest and those with traumatic brain injury (TBI). Diagnostically, EPs have largely been replaced by imaging studies (see Chapter 26 , Chapter 28 ), invasive neuromonitoring (e.g., brain oxygen monitoring), and continuous EEG (see Chapter 25 ). However, serial and even continuous EP monitoring is feasible and may be considered when continuous noninvasive neuromonitoring is warranted and EEG may be of limited value, such as in evolving spinal cord or brainstem injury. In addition EPs may be used to complement or supplement the information provided by imaging or other monitors. Consensus recommendations to guide optimal use of electrophysiologic tests including SSEP for diagnosis, follow-up, and prognosis in the ICU have been published.
Postanoxic Coma (Hypoxic-Ischemic Encephalopathy)
After cardiopulmonary resuscitation, the neurologic examination does not reliably predict the presence or absence of specific SSEP patterns, particularly absent or present N20 responses. Nevertheless, EPs can help predict outcome, particularly poor outcome rather than good outcome, after cardiac arrest. Normal SSEP responses have less predictive power than abnormal or absent responses. It does not appear that induced hypothermia after cardiac arrest (e.g., 33° C for 24 hours) affects the predictive power of SSEPs, although withdrawal of care may bias these observations. In particular, bilaterally absent cortical SSEP responses 3 days after resuscitation are associated with a poor prognosis. Short-latency SSEPs (N20) may be the most reliable method to predict poor outcome in anoxic-ischemic encephalopathy. This is included in practice parameters on outcome prediction in comatose patients after cardiopulmonary resuscitation from the Quality Standards Subcommittee of the American Academy of Neurology and regarded as Level II evidence (i.e., probably disease specific). For example, in a prospective study of 407 cardiac arrest patients, Zandbergen et al. observed bilaterally absent N20s in 45% of patients that were comatose at 72 hours; all these had poor outcome ( Fig. 24.3 ). In a subsequent systematic literature review that included 4500 anoxic patients, Zandbergen et al. observed that bilaterally absent N20s within the first week were 100% specific for poor outcome. However, among those patients with poor outcome, 46% may have preserved N20 responses within the first week of cardiac arrest ( Fig. 24.4 ). SSEPs perform favorably when compared with other outcome predictors such as the pupillary light response, the Glasgow Coma Scale (GCS), or EEG. Combining SSEPs with other outcome predictors such as EEG or serum markers of neuronal injury provides high prognostic accuracy. Although SSEPs may allow the clinician to predict poor prognosis with some degree of accuracy, good outcome is much more difficult to predict. Discrepancies between SSEP findings and outcome (i.e., recovery of SSEPs but absence of clinical improvement) may be explained by hypoxic injury sparing the somatosensory tracts, or secondary brain injury acquired after the SSEPs were obtained (i.e., recurring cardiac arrests or cardiac output failure).


Several other SSEP parameters including the CCT, the N20-to-P25 amplitude ratio, and the N70 latency are associated with outcome but are less robust than absent cortical SSEP responses. When the N20s are present or questionable, late SSEP components such as the N70 (absent or delayed >130 msec) can help identify patients likely to have a poor outcome. However, false positives are common (between 4% and 15%) and make it impractical to base treatment decisions on this finding. Late components may be better associated with long-term cognitive outcome than short-latency components but are not routinely used for patients with cardiac arrest. The role of BAEPs after cardiac arrest is less well studied. However, middle-latency auditory evoked responses are absent in patients who die or remain in a persistent vegetative state. Finally, EPs can be used to guide care in neonatal encephalopathy or childhood asphyxia.
Traumatic Brain Injury
Following TBI, SSEPs can help predict short-term mortality and long-term outcome. Bilaterally absent cortical SSEP responses with preserved peripheral and spinal potentials are associated with poor outcome. In general, SSEPs more accurately predict poor outcome than good outcome. For example, in a review of 44 studies, Carter and Butt observed that 71% of patients with normal SSEPs ( N = 553) have a favorable outcome (normal or moderate disability; Fig. 24.5 ). By contrast when SSEPs are absent bilaterally ( n = 777), 99% have an unfavorable outcome (severe disability, vegetative state, or death). The positive predictive value and sensitivity are 71% and 59%, respectively, for normal SSEP and a favorable outcome; and 99% and 46.2%, respectively, for bilaterally absent SSEP and an unfavorable outcome. Patients who have a favorable outcome despite bilaterally absent SSEPs ( N = 12) are more commonly children, have focal lesions, have subdural or extradural fluid collections, or have undergone decompressive craniotomy in the 48 hours before the recording. Overall, SSEPs perform favorably when compared with other TBI outcome predictors such as the GCS, pupillary or motor responses, computed tomography (CT), and EEG findings, and the the false-positive rate of bilaterally absent SSEPs after TBI is less than 0.5%.

Repeated SSEPs can help detect secondary injury (e.g., hematoma enlargement or increased intracranial presure [ICP]), brainstem herniation, or cerebral ischemia. In some patients a change in SSEPs may precede an ICP increase. Serial SSEPs also may suggest recovery ( Fig. 24.6 ). For example, reduction of latency and normalization of amplitude may occur earlier than recovery in the clinical examination. Poor long-term functional outcome (death or vegetative state at 2 years) is seen in comatose patients with traumatic brainstem lesions and absent N20s that do not recover within 48 hours of the injury. However, recovery of the N20 does not necessarily mean recovery of brain function. Prolongation or absent CCT is associated with poor 1-year cognitive and behavioral function.

Although rare, reappearance of previously absent bilateral SSEPs may occur after TBI. This is described in some patients with good outcome after infratentorial hemorrhage or following hemicraniectomy. In addition, circumscribed bilateral sensory tract contusions in the brainstem may mimic bilateral SSEP loss from supratentorial injury. This may not necessarily mean a fatal prognosis. Finally, marked attenuation of the cortical N20-P25 must be distinguished from persistent loss of these signals. BAEPs are used less frequently than SSEPs for TBI outcome prediction, but when performed, BAEP abnormalities are more frequently seen in TBI patients with poor outcome.
Acute Ischemic Stroke
Diagnosis of acute ischemic stroke (AIS), is done by neurologic examination and neuroimaging. EPs, however, may be used in a patient with infratentorial ischemia if MRI is not available or in a patient with a pacemaker who cannot have an MRI, and can be used for continuous monitoring in select patients at risk for herniation (e.g., a large cerebellar stroke). Ischemia of the pontine tegmentum results in both BAEP (loss of wave V) and SSEP abnormalities (absent N20). Ischemia restricted to the cerebellar peduncles only affects the BAEPs. False-negative EP results may occur—brainstem strokes may have normal SSEPs with medial or lateral strokes in up to 60% and 75% patients, respectively. EPs may be useful in patients with locked-in syndrome to provide objective evidence of brainstem involvement although there is no specific pattern to the abnormalities, and depending on the affected structures, BAEP and SSEP findings may vary from unilaterally normal to bilaterally absent. Finally, BAEP obtained within 24 hours of large middle cerebral artery infarction may help identify patients at risk for malignant edema and thus the need for a hemicraniectomy.
The role of EPs in outcome prediction after AIS is less well studied than TBI or anoxia and is mainly limited to case series of patients with posterior fossa or massive hemispheric infarction. Bilateral BAEP and SSEP abnormalities are seen in patients with poor outcome after pontine and mesencephalic infarction, and those with basilar thrombosis and can be particularly helpful when making decisions about prognosis when consciousness is impaired. Good outcome (moderate disability or better) is more likely when BAEPs and SSEPs are normal. EP abnormalities are of indeterminate predictive value in patients with locked-in syndrome. Cerebellar infarcts associated with coma may be accompanied by prolongation of the I to V interpeak latencies, although this abnormality may persist despite clinical improvement.
There are conflicting data on the association between EP findings and outcome after supratentorial AIS. On the one hand, normal BAEPs but not SSEPs obtained before a hemicraniectomy are associated with survival and good functional outcome (Barthel Index ≥60) after large hemispheric strokes. On the other hand, SSEP findings after small internal capsule or corona radiate strokes do not correlate with 3-month outcome (Barthel Index). Others have found that serial SSEPs and BAEPs are associated with 30-day Glasgow Outcome Scale independent of stroke type and location, with the exception that BAEPs are not associated with outcome in those with infratentorial lesions. In part these differences in results may stem from differences in study design and small sample size.
Several EP observations are associated with stroke location. AIS of the ventroposterior thalamus leads to an absent response, decrease in amplitude, delay in peak latency, or attenuation of median N20-P25 and tibial P40 components. Lateral ventroposterior lesions preferentially affect tibial SSEPs, whereas medial lesions affect median SSEPs. Strokes that affect the thalamocortical pathways lead to less consistent SSEP abnormalities. Although large strokes of the corona radiate may lead to similar SSEP abnormalities as thalamic strokes, smaller lesions of the corona radiate or internal capsule often have an inconsistent relationship between EP findings and sensory symptoms. This inconsistency is likely from incomplete involvement of the thalamocortical pathways in small strokes.
Intracerebral Hemorrhage
Evoked potentials (EPs) have little if any role in the diagnosis of an intracerebral hemorrhage (ICH). In addition EP localization value is limited after thalamic or putaminal ICH. However, BAEP abnormalities may provide information about small posterior circulation bleeds, particularly when tegmental structures are affected. Serial SSEPs may provide an alternative to frequent brain imaging to examine potential for neurologic recovery, particularly for ICHs in the posterior circulation. Recovery of motor function after ICH in the putamen or thalamus generally parallels the normalization of SSEP components.
SSEPs and BAEPs can provide prognostic information in patients with an ICH located in the brainstem, particularly in the pons or in deep supratentorial structures including the putamen and thalamus. In particular, prolongation of I to V interpeak latencies in the BAEP and bilaterally absent N20s in the SSEP are are associated with poor prognosis. SSEPs may have a lower false-negative rate for poor outcome than BAEPs after putaminal ICHs. Good functional outcome is likely when BAEPs are normal and SSEPs are normal after putaminal ICH. Following a pontine ICH, good outcome may be seen if normal amplitudes and latencies of waves I to V in BAEPs are at least unilaterally present.
Subarachnoid Hemorrhage
There is an unclear relationship between the severity of subarachnoid hemorrhage (SAH) and EP findings; some studies suggest an association between CCT prolongation or absence of SSEP and BAEP responses and the Hunt Hess grade, whereas others found no association between CCT abnormalities and clinical severity. Xenon single photon emission computed tomography (SPECT) studies suggest CCT prolongation is associated with impaired cerebral blood flow (CBF) after SAH, particularly when CBF is less than 30 mL/100 g/min. However, EP studies do not have a role in vasospasm diagnosis in clinical practice.
Studies in the 1970s did not find an association between EP findings and outcome. Subsequent studies describe an association between BAEP and SSEP abnormalities including bilateral CCT prolongation (>6 msec) or bilaterally absent cortical responses and poor outcome or death after SAH. Tibial rather than median nerve SSEP may be more robust in outcome prediction. Patients with normal SSEPs have the potential for a good outcome. These differences may be associated with advances in SAH management particularly for poor-grade SAH patients.
Spinal Cord Injury
EPs have an established role during spinal surgery but are not frequently used to monitor patients with spinal cord injury (SCI) in the ICU. Although EP abnormalities after SCI, including spinal cord ischemia, are associated with long-term outcome, they do not appear to add any prognostic accuracy to the neurologic examination but can be used to supplement it. SSEPs may have a role in injury assessment for comatose patients with suspected SCI. In these patients the extent of neurologic deficits is associated with the degree of SSEP abnormalities: N9 and N20 latencies for the upper limbs and P40 and P60 for the lower limbs.
Brain Death
There is no single method to confirm brain death, the diagnosis of which depends in large part on the clinical examination (see Chapter 13 ) and strict protocols. However, Wijdicks in a review conducted a decade ago, found there was a lack of consensus on the exact criteria in many published practice parameters and guidelines and that 28 of 70 (40%) of the practice guidelines he studied mandated the use of confirmatory testing. Many of these recommended use of EPs. When repeat studies are performed, patients who progress to brain death lose subcortical SSEP and all BAEP responses. Loss of the median nerve SSEP noncephalic P14 and of its cephalic referenced reflection N14 and the N18 is seen in brain death. No specific BAEP pattern is seen in patients who progress to brain death. Diagnostic accuracy is improved by combining BAEPs and SSEPs. EPs can also be helpful in patients when there is doubt about the clinical findings or cardiopulmonary instability limits the ability to perform an apnea test.
Coma Associated with Metabolic or Toxic Encephalopathy
The International Society for Hepatic Encephalopathy and Nitrogen Metabolism (ISHEN) published guidelines for the use of neurophysiologic investigations in hepatic encephalopathy. There are three main recommendations. First, the choice of neurophysiologic test depends in part on the severity of encephalopathy. Quantitative EEG may be used for mild forms, but SSEPs should be considered for more severe encephalopathy. Second, when N20 responses are bilaterally absent, further evaluation is recommended to determine the extent of irreversible brain injury before liver transplantation. Third, SSEPs and BAEPs may be used to help make decisions about ICP and its management, for example, when an ICP monitor is not feasible or to complement the information of an ICP monitor (i.e., determining the consequence of an ICP increase).
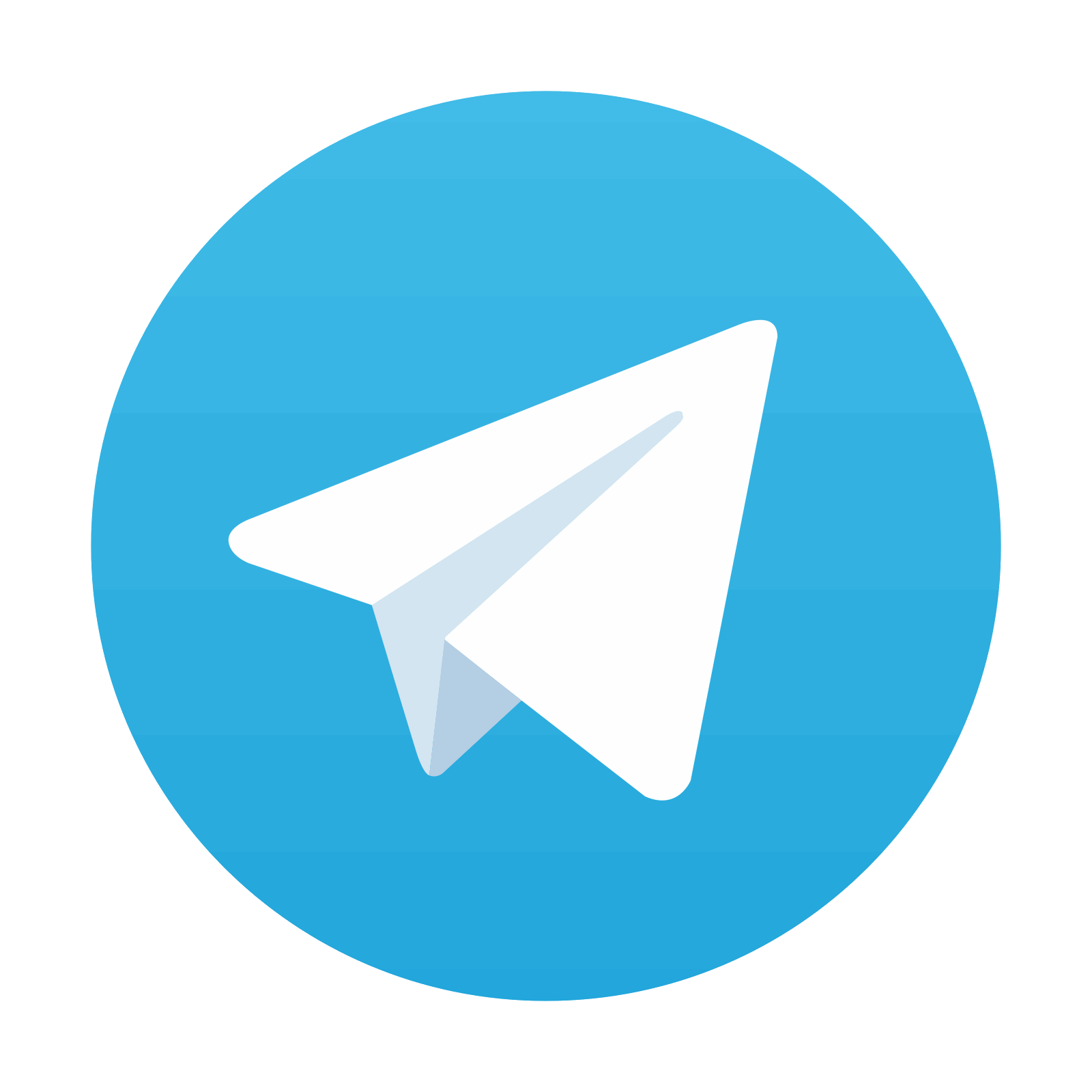
Stay updated, free articles. Join our Telegram channel

Full access? Get Clinical Tree
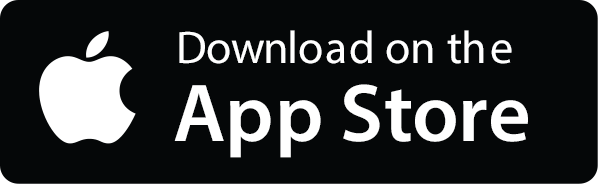
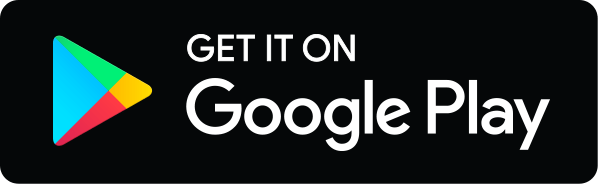