Figure 47.1. Internal carotid artery.
47.1.2 Pathophysiology of Carotid Artery Atherothrombosis
Stenosis severity seems to be the most important risk factor for ischemic stroke [10]. This notwithstanding, the relevance of the structural conformation of atheromatous plaque has recently been emphasized [11], which is why it seems more adequate to use the term “carotid artery disease” instead of “carotid stenosis”.
The vascular endothelium is responsible for maintaining the patency of the arterial wall through the balanced release of pro-atherogenic (thromboxane, angiotensin II, endothelin, thrombin, etc.) and anti-atherogenic substances (prostacyclins, nitric oxide, serotonin, histamine, etc.) Vascular risk factors, hemodynamic effects and some specific humoral products may cause endothelial dysfunction, a systemic and potentially reversible process. This dysfunction triggers the formation of carotid atheromatous plaque, which begins with cholesterol internalization and the incorporation of inflammatory cells into the vascular wall [12]. Local rheological factors and shear stress produced by the blood flow on certain regions of the arterial tree such as the carotid sinus favor these pathological phenomena.
Unless there is early intervention to reduce risk factors, the atheromatous plaque becomes increasingly vulnerable: reduced thickness of the fibrous capsule [13], ulceration, intraplaque hemorrhage due to vasa vasorum rupture, large necrotic lipidic core (>40%) [16,17] or in situ thrombosis [18].
Histologically, the vulnerable plaque shows an intense inflammatory-macrophagic infiltrate [19]. An increase in neovascularization of the plaque through the vasa vasorum has recently been described [20]. Each of these factors is associated with a higher risk of arterio-arterial embolic phenomena. Nevertheless, not all vulnerable plaques can generate embolisms. In fact, they can also be found in the carotid arteries of asymptomatic patients [14]. The “vulnerable patient” theory, which draws on the concept of thrombophilia or a transient pro-thrombotic state induced by various triggering mechanisms [21], has been advanced to explain this phenomenon. It has been observed that such patients exhibit elevated levels of C–reactive protein (CRP), leukocytosis, metalloproteinases, proteolytic enzymes and other biological markers [22-24]. These markers are intrinsically related to oxidative stress and inflammatory processes, and might be the mediators in the transformation of non-vulnerable plaques into vulnerable ones.
47.1.3 Ischemic Stroke Etiopathogeny in Patients with Carotid Artery Disease
Ischemic stroke in patients with carotid artery disease can be explained in terms of processes related to the arterial stenosis itself or to unrelated mechanisms such as cardioembolism, small vessel disease or, less frequently, hypercoagulability states. Among the 1021 strokes that occurred in 2885 NASCET patients, 11% were due to cardioembolism, 21% to small vessel disease, and 68% to carotid disease.
When analyzing exclusively the strokes that occurred in asymptomatic patients with stenosis >70% in the ICA territory (contralateral to the treated ICA), 45% were attributable to cardioembolism or small vessel disease [25], which is consistent with the multiple mechanism phenomenon proposed by Caplan [26].
With regard to the mechanisms directly related to carotid artery disease, we must consider hemodynamic phenomena causing severe hypoperfusion (<20% of brain blood flow compared to the contralateral hemisphere) [27], local thrombosis on a vulnerable plaque with subsequent distal embolism [18,28] or microthrombi formation on the surface of macro- and microscopically undamaged endothelial plaques (endothelial dysfunction without structural damage) [29,30]. Infrequently, emboli originating in the heart and impacting on a pre-existent carotid stenosis can be found.
47.1.4 Risk Factors
In general, risk factors for atheromatous plaque formation are the same as those that lead to atherosclerosis of other vessels, such as the intracranial, coronary, inferior limb arteries, etc.: arterial hypertension, dyslipidemia, smoking, diabetes, sedentary lifestyle, obesity, infectious disease and metabolic syndrome among others. Perhaps the most interesting fact is that the cardiovascular risk factor load is higher in patients with carotid artery disease (even asymptomatic) than in those who suffered an ischemic stroke of non-carotid origin. This finding is shown in Table 47.1 which compares the risk factor load of patients with asymptomatic carotid disease to those who suffered an ischemic stroke of non-carotid origin.
Dyslipidemia and smoking seem to be risk factors differentially associated with carotid disease. These data further support the hypothesis that an accumulation of risk factors might be necessary for the formation of atheromatous plaque which may later become vulnerable but not sufficiently so to cause an ischemic stroke.
Asymptomatic carotid artery disease [n=113] | Ischemic stroke or TIA [n=175] | p | |
Age, years (mean ±SD) | 67.3±8.2 | 67.6 ± 13.0 | 1.00 |
Male gender (%) | 74.3 | 64.6 | 0.08 |
Arterial hypertension (%) | 86.7 | 83.4 | 0.45 |
Diabetes mellitus (%) | 32.7 | 22.9 | 0.06 |
Dyslipidemia (%) | 75.2 | 54.3 | <0.01 |
Smoking (%) | 58.4 | 42.3 | 0.01 |
Myocardial infarction (%) | 35.4 | 17.1 | <0.01 |
Table 47.1. Risk factor load in patients with asymptomatic carotid artery disease and in patients with ischemic stroke of non-carotid origin [Data from Stroke Center. Neuroscience Institute, Favaloro Foundation, 01/01/06 to 12/31/08].
47.1.5 Clinical Manifestations of Ischemic Stroke due to Carotid Artery Disease
More severe neurological syndromes usually arise in ischemic strokes caused by carotid artery disease than in those doe to such other mechanisms as small vessel disease. Greater severity is related to the large size of infarcts. They have been classically defined as being >15 mm, although this threshold has recently been questioned [31]. Localization is usually cortico-subcortical, with a predominance of the MCA territory. This explains why, aside from the classical motor and/or sensory deficit signs, a patient with an ischemic stroke of carotid origin may present cortical signs such as aphasia, neglect, anosognosia, sensory extinction or visuospatial disturbances.
A distinctive clinical finding is retinal compromise presenting as transient monocular blindness (amaurosis fugax), ipsilateral to carotid disease. Less frequently, irreversible monocular blindness may arise. It usually manifests within a few seconds (described like a curtain coming down or sideways), rapidly affecting vision with possible total visual loss. It may last from a few seconds to minutes (usually <10 minutes). It may also start as a progressive darkening of vision in the affected eye. These transient phenomena are caused by hypoperfusion of the ophthalmic artery or microembolisms from the ICA to the central retinal artery with posterior recanalization mediated by the endogenous fibrinolytic system [32].
Clinical manifestations such as dizziness, vertigo or syncope are not attributable to carotid artery disease [33].
47.1.6 Diagnosis of Carotid Artery Disease
Patients with a history of ischemic stroke should be screened for the presence or absence of both extra- and intracranial carotid disease. In the general population without a history of stroke or TIA, the indication for carotid artery disease evaluation is questionable. According to the U.S. Preventive Services Task Force (USPSTF), the potential benefit derived from the identification and later surgical resolution of a carotid artery stenosis >70% in asymptomatic patients does not outweigh the risk of postoperative brain digital subtraction angiography (DS-A) and the surgery itself. In conclusion, the USPSTF recommends not screening the general population for carotid disease (Level D evidence) [34].
Over the past years, considerable progress in the visualization and identification of the vulnerable plaque components has been achieved with ultrasound techniques such as intravascular Doppler ultrasound (IVUS) and other imaging methods, including magnetic resonance angiography (MRA) and multislice angiotomography. These procedures have become daily practice in specialized national and international medical centres (Figure 47.2).
Figure 47.2. Doppler ultrasound with power doppler and angiotomography of the internal carotid artery. (A, B). CT-A of the ICA showing a bulbar stenosis by a plaque with fibrolipidic component (pointed arrows), scarce calcium component (black arrows) and a large ulcer (white arrows). (C, D). Doppler and power doppler ultrasound scans of the same artery.
Nevertheless, their real clinical and epidemiological value needs to be confirmed by large prospective studies before they can be formally recommended, given the fact that they still lack a defined role in the algorithm for the evaluation of patients with carotid artery disease (Level B evidence, class IIa) [35]. For the time being, of all the arterial abnormalities that can be documented by diagnostic tests, only the degree of stenosis is consistently significant in multivariate models, yielding the highest predictive value for ipsilateral ischemic stroke [36].
Several strategies or diagnostic algorithms for the evaluation of patients with carotid artery disease have been proposed. The method used for determining stenosis degree is not a minor detail. Many of the discrepancies in the results of randomized studies on the surgical approach to carotid artery disease can be explained by differences in the measurements [37,38].
Carotid artery color Doppler ultrasound (CDUS), because of its relatively low cost and wider availability, is the most widely used technique in clinical practice [39]. Nevertheless, it is highly operator dependent. Another of this method’s drawbacks is the impossibility to evaluate proximal CCA and distal ICA, which limits the detection of tandem stenosis [40]. Moreover, by not assessing intracranial circulation, the possibility of detecting aneurysms is lost, these being findings that might radically change the choice of treatment [40]. It should be noted that intracranial circulation could be assessed by transcranial Doppler (TCD). But because the sound beam is hampered by osseous interposition, TCD requires additional training, which results in a lower rate of use. Finally, the quality of the printed images is usually not sufficient for evaluation by a professional who was not present during the test, which is why the attending clinician frequently has to trust the report blindly. These remarks aside, there is a growing tendency to plan surgical treatment based on CDUS scans performed by two different operators or on imaging findings from CDUS and another non-invasive method instead of DS-A. Although excellent correlations between CDUS and DS-A have been reported, they were never perfect, an essential criterion for the choice of surgical strategy [41]. Several studies confirm that surgical choice cannot be made based upon information obtained exclusively from CDUS [42,43].
MRA with time of flight (TOF) requires no use of paramagnetic contrast material. Still, dynamically administered contrast material to visualize the whole arterial tree, from its beginning in the aortic arch to its terminal branches, has substantially improved image quality. This method is known as contrast-enhanced MRA (CEMRA). Like non-contrast MRA, it overestimates stenosis degree in severe pre-occlusive obstructions. Apart from this, it requires longer acquisition periods (albeit shorter than TOF MRA), which may result in a reduction of image quality if the patient moves. Some authors suggest that it is the most accurate non-invasive diagnostic method, having an excellent anatomic correlation and allowing an adequate evaluation of intracranial circulation [40,44].
Cerebral angio-tomography (CT-A) affords the possibility of obtaining images of the whole arterial tree much quicker than with CEMRA, thus avoiding movement artifacts. It requires the administration of iodinated contrast material by a pump operated by trained personnel, exposing the patient to high doses of radiation. This technique allows more exact visualization of the anatomical features of the arteries, as well as plaque components. Based on our experience, the diagnostic yield seems to be higher when evaluating the images dynamically from the CT console monitor than when analyzing the slides in a negatoscope or through a selection of digitalized images. Image reconstruction, which may entail some hours, is a disadvantage but it allows visualization of the intracranial circulation with remarkable clarity and detail.
DS-A is considered the gold standard for the assessment of extra- and intracranial cerebral arteries. It requires an intra-arterial catheter for the injection of iodinated contrast material and involves a considerable radiation burden. Between 1% (incapacitating and/or fatal) and 4% (mild) of ischemic strokes may arise as complications [45]. Other complications such as catheter insertion site hematoma may occur. Another disadvantage is that, because it can only identify plaque ulceration, it provides no information on plaque morphology. There are several methods for measuring the degree of stenosis. The two most widely used are those described in the NASCET and ECST (European Carotid Surgery Trial) studies (Figure 47.3) [46,47]. The more widely used is NASCET’s [48], although both are reliable and correlate well with one another. The following formula must be applied to convert measurements: ECST = 40 + (0.6 x NASCET).
Figure 47.3. Measurement of extracranial carotid stenosis according to NASCET and ECST.
In conclusion, CDUS is an adequate method for carotid artery disease screening in patients with a history of ischemic stroke or TIA. It is preferable over other methods because of its wider availability and lower cost. When the potential presence of intracranial disease may result in therapy modification, appropriate non-invasive tests will be necessary (MRA or CT-A). Both NASCET and ECST used DS-A to confirm the degree of stenosis [46,47]. Extrapolation of the two trials’ conclusions to patients evaluated with non-invasive tests such as MRA, Doppler or CT-A may be risky, hastening wrong decisions. Nonetheless, the American Heart Association/American Stroke Association (AHA/ASA) suggests that when consistent results are obtained from two non-invasive methods (CDUS, MRA, CT-A), DS-A is not necessary. This imaging study may be reserved for those cases in which non-invasive methods do not correlate. (Level B evidence, class IIa) [35].
The AHA/ASA guidelines for the evaluation of extracranial ICA stenosis degree and selection of candidates for CEA are: a) CDUS should not be used as the only diagnostic method when considering endarterectomy (Level A evidence, class III); b) the method of choice for measuring stenosis degree is DS-A (Level A evidence, class I); c) two non-invasive methods may be used (CDUS, MRA, CT-A) for the assessment of stenosis degree, although less accurate than DS-A, which may increase the chance of indicating an erroneous treatment (Level B evidence, class IIa); d) subocclusive lesions are more accurately detected by DS-A, closely followed by CT-A (Level B evidence, class IIa) [49].
47.2 Carotid Endarterectomy
Just 7 months after Fisher envisaged the first carotid surgery [2], Raúl Carrea performed the first operation on a patient with carotid artery obstruction, removing the atherosclerotic plaque and anastomosing the external carotid artery (ECA) to the ICA[50,51]. The first CEA with the presently used technique (except for slight modifications) was performed by Michal DeBakey in 1953, but the case report was not published until 1975 [52]. The patient had a 19-year follow-up and died from an acute myocardial infarction. In 1954, Eascott performed carotid artery repair by partial resection of a previously thrombotic ICA and CCA and posterior direct anastomosis [53]. With time, the number of endarterectomies gradually increased until the 1980s, when studies began to warn about the increased rates of postoperative stroke and death [54-56]. In the early 1990s, following publication of the NASCET and ECST, enthusiasm for CEA was rekindled, opening a new chapter in primary and secondary stroke prevention [46,47,57].
47.2.1 Endarterectomy
There are two surgical techniques: the classical approach and the eversion technique (Figure 47.4). The first consists of longitudinal resection of the ICA to remove the atheromatous plaque [58]. The second involves transversal resection of the ICA origin around its entire circumference, followed by eversion of the adventitia and tunica media. With this procedure, the ICA atheroma is removed, followed by endarterectomy of the CCA with the classical technique; finally, the ICA bulb is re-anastomosed at the bifurcation [59].
Figure 47.4. Classic endarterectomy and eversion endarterectomy. (A). Classic endarterectomy with longitudinal resection along the ICA and CCA. (B). Eversion endarterectomy.
The advantages and disadvantages of both techniques are summarized in Table 47.2. A systematic revision of randomized studies comparing classical endarterectomy to eversion technique endarterectomy (2590 surgeries) showed no significant difference in perioperative stroke, death or local complications [60].
Classic endarterectomy | Eversion endarterectomy |
Advantages | |
Shunting from the beginning of surgery | Lower re-stenosis rate |
Distal atheroma resolution possible | No need for patching |
Continuous view of distal intimal segment | Redundant segment resection possible (prevents kinking) |
Shorter surgical time | |
Disadvantages | |
Longer surgical time | Inability to place shunt until plaque is removed |
Predisposition to long-term kinking (due to elongation of the ICA during surgery) | Distal intimal segment might not be visualized |
Risk of failure to detect intimal flaps | |
Technical difficulties with plaques with distal extension |
Table 47.2. Advantages and disadvantages of classic endarterectomy and eversion endarterectomy.
47.2.2 Patch Use
One of the risks or potential complications of CEA is re-stenosis. Long-term re-stenosis is usually due to inadequate control of risk factors. On the other hand, re-stenosis during the first year is generally caused by neointimal hyperplasia [61]. Various different patch types have been used to prevent neointimal hyperplasia. Although patches may be associated with infection or hematoma (in case of rupture), their use has reduced the rats of severe complications.
A systematic review of 10 randomized studies comprising 2157 procedures in 1967 patients compared the result of endarterectomy performed with autologous (venous) or synthetic patches vs. primary closure surgeries (without patch) [62]. Patch use was associated with a reduction in the risk of ipsilateral stroke at 30 days (OR 0.31, 95% CI 0.2-0.6; p <0.001). Long-term follow-up also evidenced a lower re-stenosis rate (OR 0.24, 95% CI 0.2-0.3; p <0.00001) and lower risk of stroke or death (OR 0.59, 95% CI 0.4-0.8; p <0.0001).
47.2.3 Intraoperative Shunting
During the surgical procedure, while the atheromatous plaque is being removed, it is necessary to stop ICA circulation. This may be done with or without shunting. With the first option, hemispheric blood flow is maintains by placing a shunt between the CCA and the ICA distal to the clamping site. Without shunting, brain blood flow from the clamped ICA is maintained by collateral circulation from the circle of Willis or through extracranial circulation from the ECA. A study using continuous monitoring of brain blood flow and electroencephalography (EEG) during CEA showed that without the use of shunting, the hemispheric blood flow in some patients was close to zero [63]. This resembles the effect of cardiorespiratory arrest on the brain. Use of intraoperative shunting allows the surgeon more time and might work as a brain protection system to reduce the risk of ischemic stroke due to hypoperfusion. Nevertheless, most post-CEA ischemic strokes are produced by embolism of particulate matter detaching from the arterial walls and not by hypoperfusion. In fact, placing a shunt might favor embolism from the CCA as well as dissection. Two randomized studies assessing the potential benefit of shunting involved 590 patients and showed a non-significant trend toward a reduction in the risk of stroke or perioperative death [64,65]. Due to the lack of conclusive results, no recommendations on the use of shunt during CEA can be made yet.
47.2.4 Local or General Anesthesia
CEA can be performed with either local or general anesthesia. Local anesthesia has several theoretical advantages. First, shunting might not be necessary because the patient is awake and therefore available for constant evaluation throughout the surgery. This would allow the use of intraoperative shunting only when there is evidence of focal neurological deficits. For the same reasons, continuous brain blood flow monitoring or EEG would not be necessary. Nevertheless, it should be noted that not all patients tolerate the procedure, many requiring conversion to general anesthesia.
Despite the theoretical advantages of local anesthesia, the randomized General versus Locoregional Anesthesia in Carotid Surgery (GALA) study that compared local anesthesia vs. general anesthesia in CEA showed no differences in the risk of stroke, acute myocardial infarction or death at 30 days [66]. A systematic review of 4335 surgeries including the 3526 cases in the GALA study revealed no difference in the risk of stroke or death related to the type of anesthesia used [67]. Based on these results, the choice of anesthetic modality should be at the discretion of the surgical team.
47.2.5 Postoperative Complications
As with any surgical procedure, local, neurological or systemic complications may arise after CEA. Reported complication rates differ across studies [68]. This derives mainly from methodological issues. A relatively reliable approach is to analyze studies under two criteria: a) those on patients with symptomatic carotid disease vs. asymptomatic carotid disease; and b) those in the context of randomized studies vs. observational studies (Table 47.3) [69-74].
Study (no. of subjects) | Stroke | Death | AMI | Stroke or death | Stroke, AMI or death | Hematoma | Local infection | Cranial nerves |
Randomized studies in asymptomatic patients | ||||||||
ACAS (814) [69] | 1.2 | 0.1 | 0.4 | 1.5 | 1.7 | ND | ND | ND |
ACST (1405) [70] | 2.5 | 1.1 | 1.0 | 2.8 | 3.6 | ND | ND | ND |
Total (2219) | 2.0 | 0.8 | 0.8 | 2.3 | 2.9 | ND | ND | ND |
Randomized studies in symptomatic patients | ||||||||
NASCET (1415) [71] | 5.4 | 1.1 | 1.0 | 6.5 | 7.3 | 7.1 | 2.0 | 8.6 |
ECST (1729) [72] | 6.7 | 1.0 | 0.5 | 7.1 | 7.3 | 3.1 | 0.2 | 6.4 |
Total (3144) | 6.1 | 1.0 | 0.7 | 6.8 | 7.3 | 4.9 | 1.0 | 7.4 |
Observational studies in asymptomatic patients | ||||||||
NYCAS (6655) [73] (1377) [74] | 2.5 [24] | ND | ND | 3.0 [73] | ND | 4.7 [74] | 0.7 [74] | 5.3 [74] |
Observational studies in asymptomatic patients | ||||||||
NYCAS (2653) [73] (611) [74] | 5.3 [73] | ND | ND | 6.4 [73] | ND | 6.3 [74] | 0.9 [74] | 7.7 [74] |
Table 47.3. Complications associated with CEA. All values are expressed as percentage.
AMI = acute myocardial infarction; ND = no data.
47.2.6 Hyperperfusion Syndrome and Revascularization Hemorrhage
Hyperperfusion syndrome (HPS) after carotid endarterectomy or carotid angioplasty is generally reported to be between 0 and 3%. It may present clinically as a diffuse or facial, periorbital or fronto-temporal headache, vomiting, confusion, seizures or focal motor deficits. Hemodynamically, it is defined as an increase >100% in revascularized hemisphere blood flow compared to preoperative values [79]. Endothelial dysfunction mediated by free radicals and the subsequent disruption of cerebral autoregulation are thought to be the central phenomena of the pathophysiological cascade.
The principal risk factors are postoperative hypertension, diminished cerebrovascular reserve, prolonged hyperperfusion (↑cerebral blood flow >100%) after the procedure, contralateral ICA occlusion, scant collateral circulation and severe stenosis of the operated ICA, among others [80]. Thus, the best means of prevention is with strict control of postoperative arterial pressure. Neuroimaging showing diffuse, unilateral cerebral edema is a characteristic feature, although edema can also be observed in the contralateral hemisphere (Figure 47.5).
Figure 47.5. Digital subtraction angiography and non-contrast enhanced computed tomography of a patient with hyperperfusion syndrome after left carotid artery stenting. Digital angiography and the brain computed tomographies of a 65-year-old patient before (PRE), 1 hour, and 5 days after angioplasty with stenting of the left ICA. Note the stenosis in the left carotid. Evident left hemispheric edema on tomographies obtained 1 hour and 5 days after angioplasty.
Less frequently, HPS can be complicated by intracranial hemorrhage (ICH). In a survey including a total of 122,856 endarterectomies and 13,047 angioplasties performed in the United States in 2005, the frequency of ICH was 0.016 and 0.15%, respectively [81], indicating this complication was ten times more frequent in angioplasties than in endarterectomies. In this study, angioplasty was an independent predictor for ICH (OR 5.9, 95% CI 3.1-11.1; p <0.001).
47.2.7 Procedural Risk Stratification
The risk of complications secondary to CEA depends on a number of variables. As noted in Table 47.3, complications are more frequent in procedures for symptomatic stenosis than for asymptomatic stenosis. This observation is consistent with a review of 59 studies that revealed a higher risk for symptomatic patients (OR 1.62, 95% CI 1.45 – 1.81; P <0.00001) [82]. Table 47.4 summarizes the main variables influencing the risk of post-CEA complications [83,84].
Variable | HR | 95% CI | p |
Ipsilateral postoperative stroke in stenosis ≥70%, symptomatic [77] | |||
Female gender | 0.79 | 0.64-0.97 | 0.03 |
Age 65 to 74 years | 1.23 | 1.00-1.51 | 0.001 |
Age ≥75 years | 1.70 | 1.28-2.56 | 0.001 |
Diabetes | 1.31 | 1.05-1.65 | 0.02 |
Ulcerated or irregular plaque | 1.35 | 1.11-1.64 | 0.003 |
Cerebral TIA vs. retinal TIA | 1.88 | 1.38-2.55 | <0.0001 |
Stroke vs. retinal TIA | 2.33 | 1.74-3.13 | <0.0001 |
Contralateral ICA occlusion | 1.30 | 098-1.88 | 0.16 |
Previous stroke or TIA | 1.20 | 0.99-1.46 | 0.07 |
Stroke or postoperative death in stenosis 50 to 69%, symptomatic [77] | |||
Female gender | 1.50 | 1.14-1.97 | 0.004 |
Age 65 to 74 years | 0.99 | 0.76-1.32 | 0.78 |
Age ≥75 years | 0.83 | 0.49-1.41 | 0.78 |
Diabetes | 1.45 | 1.05-2.02 | 0.03 |
Ulcerated or irregular plaque | 1.37 | 1.03-1.83 | 0.03 |
Cerebral TIA vs. retinal TIA | 2.62 | 1.68-4.09 | <0.0001 |
Stroke vs. retinal TIA | 1.91 | 1.22-3.01 | <0.0001 |
Contralateral ICA occlusion | 2.21 | 1.33-3.67 | 0.002 |
Previous stroke or TIA | 1.59 | 1.21-2.09 | 0.001 |
Postoperative death in symptomatic and asymptomatic patients [76] | |||
Age ≥75 years | 1.36 | 1.07-1.68 | 0.02 |
Age ≥80 years | 1.80 | 1.26-2.45 | <0.001 |
Postoperative stroke or death in symptomatic and asymptomatic patients [76] | |||
Female gender | 1.31 | 1.17-1.47 | <0.001 |
Table 47.4. Variables affecting risk of complications at 30 days post CEA.
CI = confidence interval; HR = hazard ratio.
Based on independent risk predictors, two models for CEA-associated risk stratification have been developed [85,86]. The Halm model seems to be more appropriate for predicting neurological, medical and surgical complications (Table 47.5) [74,86]. The risk score is obtained by adding a point for each of the following factors: a) active coronary disease (grade IV angina or unstable angina); b) stroke (non TIA) related to surgery; and c) ≥50% contralateral ICA stenosis. One point is to be subtracted if local anesthesia is given. The maximum score is 3 and the minimum -1. The model is used to predict the complications until postoperative day 30: a) stroke or death; b) cardiologic complications (ventricular tachycardia, unstable angina, acute myocardial infarction, congestive heart failure); c) non-cardiologic medical complications (assisted mechanical ventilation, postoperative pneumonia, sepsis, kidney failure, pulmonary thromboembolism, deep vein thrombosis or gastrointestinal hemorrhage); d) minor neurological complications (TIA, seizures, cranial nerve damage); e) surgical wound complications (hematoma or infection).
Halm score | Stroke or death | Cardiac | Medical, non cardiac | Minor neurological | Surgical wound |
-1 | 0.7 | 2.1 | 1.4 | 4.3 | 3.3 |
0 | 1.7 | 3.0 | 2.4 | 6.4 | 5.3 |
1 | 5.9 | 6.1 | 4.9 | 8.7 | 8.3 |
≥2 | 13.0 | 8.8 | 7.8 | 12.8 | 9.8 |
Table 47.5. Halm score for predicting post-CEA complications. All values are expressed as percentage.
A neurologist is usually consulted to aid in deciding whether a patients with carotid artery disease should undergo endarterectomy. Complication rate and risk stratification is useful in the decision-making process for predicting the likelihood of postoperative complications but not whether a patient should undergo surgery or not. Instead, a decision for or against surgery should be based on two fundamental criteria: a) individual recurrence risk stratification when given the best medical treatment; and b) the applicability of randomized study results to the patient based on such diverse criteria as stenosis degree, age, gender, etc. (see below) [87].
47.2.8 Indications for CEA in Symptomatic Patients
The results of NASCET were published In 1991. This study sought to demonstrate the unequivocal benefit of surgical treatment in reducing stroke recurrence in symptomatic patients [46]. Seven years later, the final results of ECST (the European counterpart) were published with very similar results [47]. That same year, an analysis of the long-term NASCET results was published [88]. The Veterans Affairs Trial 309 (VA309) had to be interrupted when the NASCET results and a preliminary ECST analysis were published in 1991 [88]. To sustain these results, all the studies required compliance to a surgical complications rate <6%.
The three large, randomized studies proved the utility of CEA in symptomatic patients with stenosis >70%. To understand the implications of these conclusions and their applicability to clinical practice, the concept of “symptomatic carotid disease” needs to be clarified, given that not all symptoms should be considered related to the ICA. Both NASCET and ECST defined symptomatic carotid disease as a history of ischemic stroke or TIA in a carotid territory appearing up to 6 months previous to randomization. Although not exactly the same, the definition relevant for clinical practice should be “any ischemic stroke or TIA with clinical manifestations attributable to a carotid territory in the 6 months prior to the first visit”. The temporal parameter, as we will see, is not a minor detail. Although no evidence exists on this subject, we believe that carotid disease in patients with silent ischemic lesions on neuroimaging should not be considered “symptomatic” unless it can be proved (by MRI diffusion-weighted images or by comparison with previous studies) that these lesions have been present for less than 6 months.
47.2.9 Individual Risk Stratification Under Medical Treatment
There are several instruments to predict the risk under medical treatment. The most sophisticated is the Carotid Stenosis Prediction Tool available online (http://www.stroke.ox.ac.uk). This tool was developed by Rothwell and Oxford University collaborators, based on the analysis of ECST data, and later validated with NASCET’s. This system classifies patients in quintiles. Those in the three lower quintiles would not benefit from CEA at year 5 (RAR5a [risk-adjusted ratio] 0-2%), those in the fourth quintile would have a moderate benefit (RAR5a 10.8%, 95% CI 1.0-20.6), and those in the highest quintile would have the maximum benefit (RAR5a 32.0%, 95% CI 21.9-42.1). Alternatives to this model are the tables for risk stratification by color available on the same website.
47.2.10 Applicability or External Validity of Randomized Trials
Once the patient’s individual risk is assessed, the next question is whether they might benefit from CEA. To answer this question, it is necessary to know if the patient meets the qualifying criteria that emerged from an analysis of the randomized studies. Specifically, we must determine whether age, gender, stenosis degree and other variables in our patient match the characteristics of those subgroups that benefited from CEA in NASCET and ECST.
As stated above, the degree of stenosis remains the most relevant piece of information when deciding on the surgical strategy in patients with carotid artery disease [36]. Nevertheless, it is not the only criterion. Several other variables may significantly interact with stenosis degree, modifying the potential impact of surgery on the reduction of risk for ischemic stroke recurrence [37]. Table 47.6 shows the number needed to treat with CEA to prevent a stroke or postoperative death over the next 5 years after surgery (NNT5a) and the absolute risk reduction for the same outcome for the same follow-up period (RAR5a), stratified by stenosis degree and the interaction with other variables [84].
The data from Table 47.6 should be analyzed cautiously. Except for subocclusive lesions, CEA reduces the risk of stroke or death in all patients with stenosis >70%. Besides the degree of stenosis, to define a lesion as subocclusive it should meet the following angiographic criteria: a) poststenotic ICA collapse; b) faster filling of the ECA than the ICA; and c) preferential filling of intracranial circulation through the collateral circulation [7]. These are usually associated with stenosis >99%. A benefit from CEA could not be proved for this specific group in the combined analysis of NASCET; ECST and VA309 (RAR5a -0.1, 95% CI -10.3-10.2) [37]. Despite this seeming lack of benefit, some patients with subocclusive lesions might benefit from this intervention. Those with recurrent TIA despite optimal medical treatment might be among them. In the ECST, in fact, CEA was associated with a significant TIA RAR5a in patients with subocclusive lesions. (RAR5a 15%, NNT5a 7; p <0.007) [92]. As shown in Table 47.6, patients with stenosis <50% do not benefit from CEA. The group with carotid artery stenosis between 50 and 69% requires further analysis. In such patients the presence of other variables may tilt the balance toward or against surgery. In this subgroup analysis, CEA was not found to be effective in women, diabetics or patients with contralateral ICA occlusion. Nor was it beneficial for those whose qualifying symptom was a retinal event or TIA [84].
These data informed the AHS/ASA recommendations: a) CEA is recommended in patients with ischemic stroke or TIA in the last 6 months and ipsilateral carotid stenosis between 70 and 99%, as long as the surgeon’s documented perioperative morbimortality rate is <6% (Level A evidence, class I); b) endarterectomy is recommended in patients with ischemic stroke or TIA in the last 6 months and carotid stenosis between 50 and 69%, depending on patient-specific factors such as age, sex, co-morbidities and severity of initial symptoms (Level A evidence, class I); c) CEA is not recommended when carotid artery stenosis is <50% (Level A evidence, class III) [93].
Variables | NNT5a | RAR5a |
All patients | 24 | 4.1 (2.3-6.0) |
Stenosis >70% | 7 | 13.5 (9.8-20.7) |
Males | 6 | 6.0 (3.8-8.2) |
Females | 10 | 9.9 (1.8-18.0) |
Age <65 years | 10 | 9.8 (3.8-15.7) |
Age 65-74 years | 7 | 13.5 (6.5-20.5) |
Age ≥75 years | 3 | 37.2 (22.9-51.5) |
History of diabetes | 6 | 16.7 (6.0-27.4) |
No history of diabetes | 8 | 12.9 (8.1-17.7) |
Ulcerated plaque | 6 | 17.1 (11.6-22.6) |
Contralateral ICA occlusion | 4 | 23.6 (3.2-44.0 |
Without contralateral ICA occlusion | 8 | 12.7 (8.3-17.1) |
<14 days between symptoms and surgery | 4 | 23.0 (13.6-32.4) |
14-30 days between symptoms and surgery | 6 | 15.9 (6.6-25.2) |
30-90 days between symptoms and surgery | 13 | 7.9 (1.3-14.4) |
<90 days between symptoms and surgery | 14 | 7.4 (-3.3-18.1) |
Ocular symptoms (amaurosis fugax or infarction) | 18 | 5.5 (-1.2-12.1) |
Qualifying symptom = TIA | 7 | 15.4 (7.7-23.1) |
Qualifying symptom = stroke | 18 | 17.7 (9.9-25.5) |
Stenosis 50-69% | 13 | 7.8 (3.1-12.5) |
Males | 13 | 8.0 (3.4-12.5) |
Females | NA | -2.7 (-8.8-3.5) |
Age <65 years | 77 | 1.3 (-4.0-6.7) |
Age 65-74 years | 19 | 5.4 (-0.4-11.2) |
Age ≥75 years | 9 | 10.7 (-0.2-21.6) |
History of diabetes | 16 | 6.2 (-4.1-16.5) |
No history of diabetes | 23 | 4.3 (0.4-8.2 |
Ulcerated plaque | 18 | 5.7 (0.7-10.6) |
Contralateral ICA occlusion | NA | -16.0 (-34.8-2.8) |
No contralateral ICA occlusion | 18 | 5.7 (1.9-9.5) |
<4 days between symptoms and surgery | 7 | 14.8 (6.2-23.4) |
14-30 days between symptoms and surgery | 30 | 3.3 (-6.3-13.0) |
30-90 days between symptoms and surgery | 25 | 4.0 (-1.7-9.7) |
<90 days between symptoms and surgery | NA | -2.9 (-10.2-4.3) |
Ocular symptom (amaurosis fugax or infarction) | 67 | 1.5 (-5.5-8.4) |
Qualifying symptom = TIA | 26 | 3.8 (-2.6-10.1) |
Qualifying symptom = stroke | 13 | 7.5 (1.6-13.5) |
Stenosis <50% | 39 | 2.6 (-1.7-6.9) |
Table 47.6. NNT5a and RAR5a of CEA to prevent postoperative stroke or death in symptomatic patients stratified according to degree of stenosis and other variables. RAR5a (risk-adjusted ratio) values are expressed as percentage with 95% confidence interval.
NA = not applicable; NNT = number need to treat.
47.2.11 Age and CEA
The relationship between the age of a patient with symptomatic carotid disease and the potential benefit of endarterectomy deserves specific mention. On the one hand, postoperative mortality is higher in patients between 75 years (OR 1.36, 95% CI 1.07-1.68; p=0.02) and 80 years of age (OR 1.80, 95% CI 1.26 – 2.45; p <0.001) [83]. A similar though not significant trend has been observed for the risk of stroke and postoperative death in both age groups. Nonetheless, as shown in Table 47.6, the benefit of endarterectomy increases with age. These data might seem contradictory. The higher benefit of surgery in patients aged 75 years and older is explained by the fact that, in the long run, the recurrence rate for medical treatment overweighs the risk of perioperative stroke or death. Based on this, there are no reasons to contraindicate CEA in patients aged older than 75 years with a life expectancy >5 years.
Despite this consideration, some guidelines (see section on angioplasty) recommend carotid angioplasty in patients >80 years. An interesting meta-analysis reports the following risk factors for patients >80 years who underwent carotid angioplasty and CEA, respectively: acute myocardial infarction 0.93 vs. 2.20% (p=0.083), mortality 1.98 vs. 1.11% (p=0.253), and 7.04 vs. 1.91% (p <0.01), According to the authors, the only significant difference was evidenced in stroke frequency in patients who underwent carotid angioplasty [94]. This notwithstanding, reanalyzing the study data, the OR of CEA for different outcomes shows a lower risk of stroke and death but a higher risk of acute myocardial infarction with CEA. In summary, CEA seems to be associated with a lower risk for stroke and death but a somewhat higher frequency of acute myocardial infarction in the elderly.
47.2.12 Relevance of Time Elapsed Between Symptom Presentation and Surgery
The time between symptoms onset and surgery influences the expected benefit, which will be higher the earlier the procedure is performed. An inverse relation between time to surgery and intervention benefit documented in randomized studies since the 1990s can be explained by several mechanisms.
On the one hand, the recurrence risk is highest during the first days after the first neurological event and then decreases with time, possibly due to the effect of medical treatment on atheromatous plaque stabilization [14,95]. On the other, it is reasonable to presume that time might also favor the development of collateral circulation. Finally, and after some weeks, by balancing the potential beneficial effect of CEA with the recurrence risk, the result is neutral, counterbalancing the usefulness of surgical intervention.
As shown in Table 47.6, the most beneficial period for CEA is the first 14 days. In patients with symptomatic carotid stenosis >70%, the favorable effect of CEA is lost if deferred beyond 90 days, while in those with symptomatic stenosis 50 to 69%, it is lost after the first 14 days, making it not reasonable to indicate surgery beyond these time windows.
The meaning of “the first 14 days” warrants careful analysis. First, this period corresponds to the time between randomization and surgery in studies such as NASCET and ECST. This time window should not be considered as a synonym for a “period between initial symptoms and surgery”. In both NASCET and ECST, the patients were randomized by symptoms occurring up to 6 months earlier. This means that the period between the occurrence of symptoms and randomization (up to 6 months) should be added to the randomization-surgery time the studies reported. Hence, we could assume that the real time window in which CEA was beneficial in the randomized studies was more than 14 days.
Second, the fact that CEA might be more effective during the first 14 days does not necessarily imply that all patients should undergo surgery during that period. Paradigmatic cases that escape the standard of immediate surgery are crescendo TIA and stroke in evolution. Crescendo TIA can be defined in several ways but such events usually fit into one of the following categories: a) 2 or more episodes in the last 24 hours [96]; b) 3 or more episodes in the last 3 days [73]; and c) 3 or more episodes in the last 7 days [97,98].
Nevertheless, a systematic review revealed that urgent CEA in crescendo TIA or stroke in evolution was associated with a higher risk of stroke and perioperative death than delayed surgery (OR 4.6, 95% CI 3.4-6.3; p=0.001) [101]. The absolute risk for stroke or postoperative death attributable to urgent CEA was 20.2 (95% CI 12.0-28.4) for stroke in evolution and 11.4 (95% CI 6.1-16.7) for crescendo TIA (95% CI 6.1-16.7). Emergency surgery in patients with minor stroke or TIA with stable neurological syndromes was not associated with a higher perioperative risk (OR 1.2, 95% CI 0.9-1.7, p=0.17).
47.2.13 Indications for CEA in Asymptomatic Patients
The annual risk for ipsilateral stroke in patients with carotid stenosis >50% varies between 2 and 3% [102], increasing to 16.6% (95% CI 1-32) [103] during for a 15-year follow-up period. Acute myocardial infarction and nonvascular-related death risk also seem to be higher: 24% (95% CI 14-34) [103]. Age and presence of diabetes increase the risk [103].
Analyzing the results of large randomized studies on CEA in asymptomatic patients is much less complex than studies on symptomatic patients. The most relevant are the Asymptomatic Carotid Atherosclerosis Study (ACAS) [104] and the Asymptomatic Carotid Surgery Trial (ACST) [70]. The Veterans Affairs Cooperative Study (VACS) recruited only a male population [105]. The design of CASANOVA (Carotid Artery Stenosis with Asymptomatic Narrowing: Operation Vs. Aspirin) was criticized for its low number of patients and for excluding those with >90% carotid artery stenosis [106]. Finally, MACE (Mayo Clinic Asymptomatic Carotid Endarterectomy), comparing aspirin vs. CEA, had to be suspended for safety reasons when an excess of deaths due to acute myocardial infarction was detected in the randomized endarterectomy arm [107].
ACAS enrolled asymptomatic patients with carotid stenosis between 60 and 99% [104]. ACST enrolled patients with no history of stroke or TIA in the previous 6 months and with stenosis ≥60% [70]. Both studies showed a reduction in stroke risk in CEA patients.
Although both ACAS and ACST formed the basis for the analysis of the utility of CEA in asymptomatic patients, they are not identical. The most controversial discrepancy was that in the ACAS, the participating surgeons had to demonstrate results with a perioperative morbimortality rate <3%, whereas in the ECST, NASCET and ACST the surgeons were required to report a complication rate <6% Moreover, some of the surgeons who were originally selected were later excluded from the study due to the adverse outcomes in their first patients [108].
Noteworthy is that the ACAS reported a strikingly low rate of surgical complications. Table 47.7 compares stroke and postoperative death rates in this study with those of ACST (1.5 vs. 2.8%; p=0.07) and those of an observational study involving 6655 asymptomatic patients in New York (1.5 vs. 3.0%; p=0.02) [73]. As shown in the table, the postoperative mortality rate in the ACAS was 8 times lower than that of the two other studies. A meta-analysis of outcome after CEA not performed in randomized trials also showed a complications rate 8 times lower than that reported for ACAS [109]. The low complications rate observed in the latter might limit the reproducibility of its conclusions in clinical practice, particularly when considering the lack of information from many centres about their surgical complications rates.
In the ACST, the most questionable fact is that patients were randomized and underwent surgery with a non-centrally audited carotid artery CDUS [110]. This might have resulted in increased underreporting of subocclusive lesions, as well as mistakes in estimating the degree of stenosis [111].
Unlike symptomatic carotid studies, both the ACAS and ACST concluded that increasing degrees of stenosis are not related to a higher benefit from endarterectomy. This merits careful interpretation. Based on these data, the only variable related to stenosis degree that should be considered in the surgical indication is whether it is higher or lower than 60%.
As with symptomatic carotid disease, other variables can tilt the decision for or against surgical intervention. Gender seems to be a determinant with a higher impact on CEA outcome in asymptomatic patients. A meta-analysis of the ACAS and ACST results evidenced a reduction in the risk of perioperative stroke and death at 2.3 years in men (OR 0.49, 95% CI 0.36-0.66) but not in women (OR 0.86, 95% CI 0.63-1.45) [112]. Hence, CEA would not be advisable in asymptomatic women.
Endpoints | ACAS | ACST | ||||
NNT5a | RAR5a | p | NNT5a | RAR5a | p | |
Stroke | 17 | 5.9 | 0.004 | 19 | 5.4 | 0.0001 |
Disabling stroke or death | 37 | 2.7 | 0.26 | 40 | 2.5 | 0.004 |
Table 47.7. NNT5a and RAR5a of CEA to prevent different endpoints in asymptomatic patients.
Carotid atheromatous plaque progression can occur in approximately 9% of patients within 6 to 9 months. It is defined as moving from a lower to a higher category on a scale that stratifies the degree of stenosis according to 6 levels: 0 to 29%, 30 to 49%, 50 to 69%, 70 to 89%, 90 to 99%, and 100%. It is not only associated with a higher risk of ipsilateral stroke (HR 2.0, 95% CI 1.0-4.1; p=0.04) [113-115] but also with a higher risk of acute myocardial infarction (HR 2.4, 95% CI 1.1-5.4; p=0.04) and cardiovascular death (HR 1.8, 95% CI 1.0-3.0; p=0.04) [113]. Although the postulated association is clear, there is still no evidence to recommend endarterectomy solely on the criterion of carotid plaque progression, although it might be an element to be considered in the decision process.
With regard to age, according to the ACST, the difference in the risk of stroke in the carotid territory between those who underwent surgery and those who did not was 7.8% (95% CI 4.3-11.3, p <0.0001) in patients <65 years and 7.5% (95% CI 4.7-10.3; p <0.0001) in patients 65 to 74 years of age [70]. Due to the low number of patients involved, determining whether endarterectomy might have been of benefit for patients >77 years was impossible. The way in which these data were presented should be carefully considered, given that the RAR5a for the combined outcome of stroke of any cause and perioperative death were not reported.
Another risk marker in asymptomatic patients is the presence of microemboli detected by carotid artery ultrasound or TCD imaging. In a recent study, the annual event rate in asymptomatic patients with carotid stenosis between 60 and 99% and microemboli was 4.6%, while the rate was 2.4% (p=0.032) [116] for those without microemboli. The value of microemboli detection in clinical practice is yet to be determined.
In conclusion, CEA in asymptomatic patients should be reserved for a select subgroup. So far, this group has been defined as men <75 years of age, with stenosis >60%, as long as the surgical team’s documented morbidity and mortality rate is <3%. It is highly likely that more evidence supporting non-surgical medical treatment over surgery will emerge very soon. In that eventuality, CEA might not be the first option for a great majority of asymptomatic patients and the most important approach will be to optimize medical treatment and interventions to reduce the risk of coronary disease, which remains the leading cause of mortality in this population.
47.3 Carotid Angioplasty
Although CEA is the most commonly performed procedure to reduce stroke risk in patients with symptomatic and asymptomatic carotid stenosis, the advent of percutaneous techniques has lead to the development of carotid angioplasty (CA) and CA with stent (SCA) techniques. The first CA procedure was described by Mathias in 1977 [117,118].
Among the potential advantages of CA and SCA are their less invasive nature is emphasized (they can be performed under local anesthesia and sedation), shorter hospital stay, and lower probability of complications associated with concomitant coronary disease. Unlike CEA, which is limited to the cervical carotid, endovascular procedures can be performed on much more cephalic lesions, and even in the intracranial carotid. A subgroup of patients who might benefit from CA and SCA are those with “tough or hostile necks”, presenting a higher risk of complications with CEA. Patients with a history of radiotherapy or cervical surgery or tracheostomy are included in this group [119,120].
Notwithstanding its advantages, percutaneous procedures also carry the risk that mobilization and eventual displacement of the atherosclerotic plaque might result in a higher incidence of neurological events [121].
For CA and SCA, some other considerations should be taken into account:
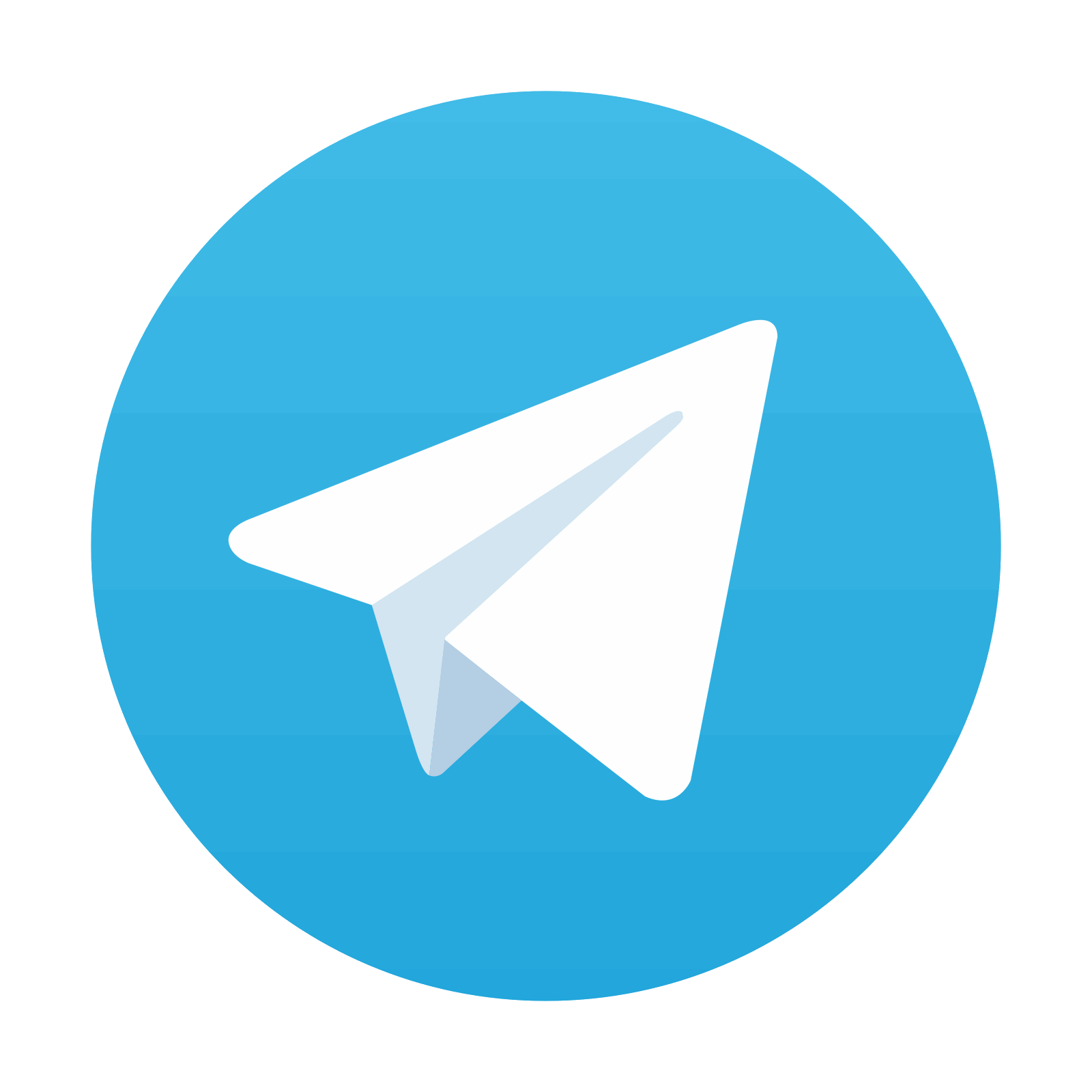
Stay updated, free articles. Join our Telegram channel

Full access? Get Clinical Tree
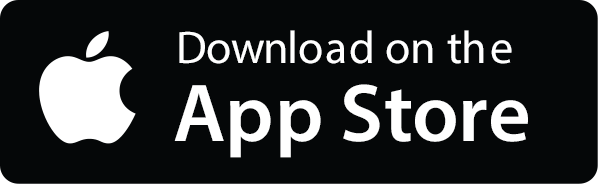
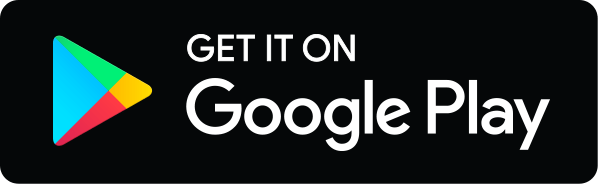