Eye Signs in Neurologic Diagnosis
Robert K. Shin
James A. Goodwin
key points
Specific patterns of vision loss correlate with specific lesions along the visual pathway from the eye to primary visual cortex.
Distinct higher-order visual processing disorders (e.g., visuospatial neglect or an inability to recognize faces) are associated with specific cortical regions.
The pupils are under autonomic control. Afferent (sensory) lesions cause poorly reactive pupils while parasympathetic and sympathetic efferent (motor) lesions cause anisocoria (unequal pupils).
Eye movement abnormalities may be conjugate (eyes remain aligned) or disconjugate (the eyes are no longer aligned). Disconjugate eye movement abnormalities are associated with diplopia (double vision).
Nystagmus (rhythmic, conjugate eye movements) are associated with vertigo (subjective feeling of movement) and oscillopsia (shaking of the visual field). Different forms of nystagmus suggest specific disorders of the brainstem or cerebellum.
Humans are highly visual animals. In order to fully scan the environment, our two spherical, lensed eyes synchronously rotate in three spatial dimensions under the control of 12 extraocular muscles, guided by the parietal lobes, frontal lobes, cerebellum, midbrain, and pons. More than half of our cranial nerves are involved in coordinating, directing, and protecting these delicate visual organs, which can move faster than any other part of the human body. Over a million retinal ganglion cells carry information from over 100 million photoreceptors from each exquisitely formed eye to multiple brainstem and subcortical nuclei,
from which myriad neuronal axons splay out into the temporal and parietal lobes and down as far as the thoracic spinal cord to connect these nuclei to a network of autonomic structures and to primary visual cortex. Visual information radiates from the occipital lobe in concentric, widening circles across the neocortex, approximately one third of which is involved in higher-order visual processing, providing us with real-time, full-color, panoramic, stereoscopic views of the world around us.
from which myriad neuronal axons splay out into the temporal and parietal lobes and down as far as the thoracic spinal cord to connect these nuclei to a network of autonomic structures and to primary visual cortex. Visual information radiates from the occipital lobe in concentric, widening circles across the neocortex, approximately one third of which is involved in higher-order visual processing, providing us with real-time, full-color, panoramic, stereoscopic views of the world around us.
Because this elegant but complex system involves every lobe of the brain, multiple subcortical structures, multiple brainstem nuclei, multiple peripheral and cranial nerves, the autonomic nervous system, and even the spinal cord, it is not surprising that many different neurologic problems (stroke, migraine, seizures, multiple sclerosis, movement disorders, dementia, traumatic brain injury, neuromuscular disorders, alcoholism, CNS infections, and cancer) affect the visual system in some way, leading to a wide variety of neuro-ophthalmologic disorders.
This chapter is a survey of a number of visual signs and symptoms that are useful in localization and diagnosis in neurologic disease. Broad categories of neuro-ophthalmologic complaints that neurologists and non-neurologists may encounter include: vision loss (blindess or blurred vision), higher-order visual processing disorders (cognitive disorders), pupillary abnormalities (poorly reactive or unequal pupils), eye movement problems (gaze palsies and double vision), and nystagmus (shaking eye movements). Each of these broad categories is organized into smaller subsections, with each subsection focusing on a limited number of related neuro-ophthalmologic disorders.
VISION LOSS
Vision loss may range from mild blurred vision to complete blindness and may involve one or both eyes. When approaching a patient who complains of blurred vision or vision loss, the first step is to make sure that the patient has been evaluated by an eye doctor (ophthalmologist or optometrist) to rule out an ocular disorder (a problem with the cornea, lens, vitreous, or retina). Once disorders such as refractive error, glaucoma, cataract, vitreous hemorrhage, retinal artery or vein occulusion, and retinal detachment have been ruled out, a neurologic evaluation can begin.
▪ SPECIAL CLINICAL POINT: Every patient with vision loss should be evaluated by an eye doctor to rule out ocular problems before the neurologic evaluation begins.
Evaluation of Vision Loss
The evaluation of vision loss involves tests of visual acuity and color vision, the swinging flashlight test, an evaluation of the optic disc appearance, and testing for visual field defects. The information gleaned from these tests must be combined in order to accurately localize and diagnose the source of the vision loss.
Visual Acuity Visual acuity, tested with handheld or wall-mounted Snellen eye charts, is the most basic part of the visual examination. The best corrected visual acuity possible is desired (patients should wear their glasses or contact lenses). A pinhole may be used to minimize refractive error if lenses are not available.
Color Vision Color perception is an extremely sensitive, although subjective, measure of optic nerve dysfunction. If specialized color plates are not available, present a fairly large bright red object and ask the patient if there is any apparent difference in the redness of the object between the two eyes. Patients may report a subjective loss of color intensity (color desaturation) in one eye compared to the other. Reds may be shifted to a darker amber color or bleached toward a lighter, pinkish, or yellowish color. Patients can be asked to subjectively quantitate the degree of color
desaturation—“If this (covering one eye) is a dollar’s worth of red, how much is this (covering the other eye) red worth?”
desaturation—“If this (covering one eye) is a dollar’s worth of red, how much is this (covering the other eye) red worth?”
Swinging Flashlight Test Damage to the anterior light pathway (optic nerves, optic chiasm, and optic tract) can be detected by illuminating each eye separately with a focal light source. Swinging the flashlight back and forth provides a sensitive means of comparing the afferent function of the two eyes (Fig. 26.1).
Normally, the amount of pupillary constriction will be the same regardless of which eye is being stimulated by the flashlight. In the setting of an afferent defect, however, both pupils may dilate when the light is swung to the affected eye, with both pupils constricting when the flashlight is swung to the normal eye. (Remember that even if only the eye illuminated by the flashlight can be seen, the unseen fellow eye is undergoing the same consensual pupillary constriction and dilation that is observed in the illuminated eye.) This asymmetry is the relative afferent pupillary defect (RAPD) or Marcus Gunn pupil (see section Relative Afferent Pupillary Defect).
If a patient has decreased visual acuity in one eye with normal visual acuity and a full visual field in the other eye, but has no RAPD, the possibility of refractive error, cataract, or other media opacity should be considered. If the ocular examination is normal, the absence of a RAPD could suggest that the monocular vision loss is functional (nonorganic).
▪ SPECIAL CLINICAL POINT: Cataracts and other opacities do not produce a significant RAPD even with major visual loss. This is because such opacities diffuse light within the eye and blur the visual image but do not significantly reduce the total quantity of light that reaches the retina.
Optic Disc Appearance
Ophthalmoscopy, though a challenging skill to master, is an essential part of any evaluation for vision loss (see Chapter 1). At a minimum, clinicians should be able to distinguish between a normal optic disc, optic disc pallor, and optic disc swelling with a handheld direct ophthalmoscope (Fig. 26.2).
Normal Optic Disc When decreased vision is accompanied by other signs of optic neuropathy (decreased color vision, RAPD, field loss) but the optic disc is normal, consider the possibility of acute optic neuritis or a posterior ischemic optic neuropathy, both of which may present without disc pallor or swelling. Retinal disorders may also be associated with a normal optic disc, but rarely present with decreased color vision or a RAPD.
Optic Disc Pallor Damage to the optic nerve is associated with optic disc pallor (a loss of color). Optic disc pallor generally takes time to develop, and may be associated with a history of prior optic neuropathy. Gradually progressive vision loss accompanied by optic disc pallor is concerning for a compressive optic neuropathy from an aneurysm or tumor. An optic disc that is both pale and swollen is concerning for ischemic optic neuropathy, particularly arteritic ischemic optic neuropathy caused by giant cell arteritis.
Optic Disc Swelling Swelling or edema of the optic disc may be unilateral or bilateral. Ischemic, inflammatory, and demyelinating optic neuropathies may cause disc swelling, as can increased intracranial pressure. The key to distinguishing between disc swelling from optic neuropathy and disc swelling from increased intracranial pressure (papilledema) is comparing the visual function of the nerve to its appearance.
Disc swelling due to optic neuropathy will be associated with decreased visual acuity, decreased color vision, a RAPD (if unilateral or asymmetric), and characteristic optic nerve visual field defects (see section Visual Pathway and Visual Field Defects). Disc swelling due to optic neuropathy may be unilateral or bilateral depending on its etiology.
Papilledema (which refers specifically to optic disc swelling due to increased intracranial pressure) is typically associated with relatively little visual dysfunction, especially early on. Visual acuity and color vision are often normal or only mildly affected. No RAPD is usually seen unless significant nerve damage has occurred. Visual fields may show enlargement of the blind spot or nonspecific constriction. Although papilledema is usually bilateral, it is often asymmetric (see section Papilledema).
The combination of a pale optic disc on one side and optic disc swelling on the other is the Foster Kennedy syndrome, signifying a mass lesion (e.g., olfactory groove meningioma) that compresses one optic nerve (causing disc
pallor) and raising intracranial pressure (causing papilledema of the opposite nerve). More commonly seen is the pseudo-Foster Kennedy syndrome in which sequential optic neuropathies (e.g., optic neuritis or ischemic optic neuropathy) result in a pale optic disc on one side (due to an old optic nerve injury) and a swollen optic disc on the other (due to an acute optic neuropathy).
pallor) and raising intracranial pressure (causing papilledema of the opposite nerve). More commonly seen is the pseudo-Foster Kennedy syndrome in which sequential optic neuropathies (e.g., optic neuritis or ischemic optic neuropathy) result in a pale optic disc on one side (due to an old optic nerve injury) and a swollen optic disc on the other (due to an acute optic neuropathy).
Visual Pathway and Visual Field Defects
Each eye has its own visual field. These visual fields are slightly different from each other, but overlap substantially. The retina does not directly project to the occipital lobe. Instead, visual information travels along a six-part visual pathway (Fig. 26.3).
Retinal gangion cell (RGC) axons converge to form the optic nerves, which “punch through” the back of the eye nasal (medial) to the macula, forming the optic disc. Because there are no photoreceptors on the optic disc itself and due to the optics of the eye (images are reversed and upside-down), a blind spot is created temporal (lateral) to central fixation in each eye.
Both optic nerves cross, forming the optic chiasm. Within the chiasm are RGC fibers from both eyes, some of which cross, others of which remain uncrossed.
Beyond the chiasm, crossing and uncrossed RGC fibers join to form the optic tracts. Unlike an optic nerve, which carries information from a single eye, each optic tract carries binocular information from the opposite (left or right) visual hemifield.
RGC axons (having formed the optic nerves, chiasm, and tracts) finally project onto the lateral geniculate nucleus (LGN) of the thalamus.
Axons from each LGN spread out and separate into the optic radiations. Visual information from the upper half of the visual hemifield travels through the inferior or temporal radiations (Meyer’s loop); visual information from the lower half of the visual hemifield travels through the superior or parietal radiations.
The radiations converge on the visual cortex, where higher-order visual processing begins.
Due to this complicated anatomic arrangement, lesions within different parts of the visual pathway are associated with a variety of specific visual field defects.
Optic Nerve Lesions of the optic nerve always cause unilateral visual field defects (Fig. 26.4). Optic nerve lesions are generally also associated with decreased visual acuity, decreased color vision, and a relative afferent pupillary defect.
Optic nerve damage may cause monocular blindness, a central scotoma (blurring only in the center of vision), or a centrocecal scotoma (vision loss in the center of vision that extends to the blind spot).
Reflecting the organization of RGC axons within the retina (Fig. 26.5), visual field defects due to optic nerve damage often respect the horizontal meridian (midline). Superior and inferior altitudinal defects affect the entire upper or lower field of vision respectively. Superior
and inferior arcuate defects are curved scotomas that follow the anatomic organization of the arcuate bundles.
and inferior arcuate defects are curved scotomas that follow the anatomic organization of the arcuate bundles.
In general, these patterns of visual field loss are not specific for any particular type of optic neuropathy (see section Optic Neuropathy). Central scotomas, however, are commonly seen with optic neuritis. Bilateral centrocecal scotomas strongly suggest toxic-metabolic optic neuropathy. Inferior altitudinal defects are often associated with ischemic optic neuropathy. Arcuate defects (Bjerrum scotomas) can be seen in glaucoma.
Optic Chiasm Chiasmal injury is classically associated with bitemporal visual field defects due to damage to crossing fibers that carry temporal visual field information from the nasal retinas (Fig. 26.6). Retinal ganglion cells axons from either side (right or left) of the macula travel together through the optic nerve but separate at the optic chiasm. This divergence creates the hemianopic midline and is the reason that all chiasmal and retrochiasmal visual field defects respect the vertical meridian.
Though the chiasm may be affected by ischemia, infection, or demyelination, most symptomatic lesions of the chiasm are compressive. Pituitary macroadenomas are the most common cause of chiasmal compression, but other tumors (e.g., craniopharyngioma and meningioma) and aneurysms may also injure the chiasm.
Bitemporal hemianopias may be complete (with no vision temporal to fixation in either eye), but when compression is early or mild, the hemianopia may be denser superiorly
(when the chiasm is compressed from below) or inferiorly (when the chiasm is compressed from above).
(when the chiasm is compressed from below) or inferiorly (when the chiasm is compressed from above).
Because crossing macular fibers travel posteriorly, a lesion of the posterior chiasm may result in a central bitemporal hemianopia, sparing the more peripheral temporal fields.
Rarely, an anterior lesion at the junction of the optic nerve and the optic chiasm can injure crossing fibers from the opposite inferior retina (which loop forward into the optic nerve, forming Wilbrand knee before projecting posteriorly to the optic tract). As a result, the optic nerve visual field defect in one eye may be accompanied by a superior temporal scotoma in the other eye—the junctional scotoma
Optic Tract Each optic tract is comprised of uncrossed RGC axons from the temporal retina of the ipsilateral eye and crossed RGC axons from the nasal retina of the contralateral eye. The optic tract, therefore, carries visual information from both eyes but only from the contralateral hemifield (as opposed to the optic nerve, which carries information from both hemifields but only from one eye). Like the optic chiasm, the optic tract is most commonly injured by compression from a mass lesion.
A lesion of the optic tract results in a homonymous hemianopia—a loss of vision in the contralateral visual field of both eyes (Fig. 26.7). (For example, a left optic tract lesion will cause an inability to see to the right of midline in both eyes—a right homonymous hemianopia.) In fact, all retrochiasmal visual field defects are homonymous, meaning that the visual field defects will be in the same hemifield (left or right) of both eyes.
In order to distinguish a homonymous hemianopia due to a tract lesion from one caused by a lesion of visual cortex, it is important to check for a relative afferent pupillary defect and to assess the congruity of the visual field.
Although the optic tract primarily projects to the lateral geniculate nucleus of the thalamus (see below), some RGC axons are diverted from the tract to the posterior midbrain to control the pupillary light response (see section Pupil Anatomy and Function). Furthermore, because the temporal field is larger than the nasal field, a homonymous hemianopia causes relatively more visual field loss in one eye than the other. In the case of a lesion of the left optic tract, the resulting right homonymous hemianopia will cause a larger defect in the right eye than in the left eye and may therefore be accompanied by a right relative afferent pupillary defect.
Also, homonymous hemianopias caused by lesions of the optic tract are often incongruous, meaning that the density and shape of the visual field defects do not match completely. As a rule, visual field defect congruity increases as the visual pathway approaches the occipital lobe, with visual cortex lesions typically producing highly congruous visual field defects. Congruity can only be assessed if the homonymous hemianopia is incomplete.
Lateral Geniculate Nucleus As with tract lesions, lesions of the lateral geniculate nucleus (LGN) of the thalamus will result in homonymous visual field defects (Fig. 26.8). Thalamic lesions associated with visual field defects are most commonly vascular.
Lateral (posterior) choroidal artery occlusion has been associated with a wedge-shaped homonymous scotoma along the horizontal
midline, sparing the upper and lower quadrants—a horizontal sectoranopia.
midline, sparing the upper and lower quadrants—a horizontal sectoranopia.
Anterior choroidal artery occlusion may result in the exact complement of the other geniculate syndrome, superior and inferior homonymous quadrantic defects that spare the area along the horizontal meridian—a quadruple sectoranopia.
Optic Radiations Axons radiating outward from the lateral geniculate nucleus toward primary visual cortex form two thin but wide bands that travel separately within the deep white matter of the cerebral hemispheres. Visual information from the upper visual field travels inferiorly through the temporal lobes, while information from the lower visual field travels superiorly through the parietal lobes. As a result of this separation, it is unusual for both sets of optic radiations to be injured simultaneously.
A lesion of a single set of optic radiations affects half of the contralateral hemifield, resulting in a homonymous quadrantanopia (Fig. 26.9). Temporal lobe lesions, including strokes, tumors, encephalitis, and demyelination, may damage the inferior radiations as they loop forward and then back toward the visual cortex, producing a superior quadrantanopia, also known as a “pie in the sky” defect. Similar lesions of the parietal lobe may damage the superior radiations, causing an inferior quadrantanopia.
Visual Cortex The visual pathway ends in the occipital lobe as the superior and inferior optic radiations converge on primary visual cortex (also known as calcarine cortex or striate cortex).
Lesions of the visual cortex, like lesions of the optic tract, frequently result in a contralateral homonymous hemianopia (Fig. 26.10). The most common causes of homonymous hemianopias due to an occipital lobe lesion are posterior cerebral artery infarction, hemorrhage, trauma, and tumor (Fig. 26.11). Rarely, patients present with homonymous hemianopias but no abnormalities on MRI, which raises the possibilies of Alzheimer disease or the Heidenhain variant of Creutzfeld-Jakob disease.
Homonymous hemianopias caused by visual cortex lesions are frequently complete. When incomplete, homonymous hemianopias tend to be congruous. Homonymous quadrantanopias can also be seen when damage to the visual cortex is limited.
Occasionally, central vision may be spared within the hemianopic field. The classic explanation for this macular sparing is collateral blood supply to the occipital lobe from a branch of the middle cerebral artery, though there are other possible explanations as well.
Rarely, a lesion may selectively affect only the deepest, most anterior part of the visual cortex, producing a visual field defect that is limited to the peripheral portion of the temporal field of the contralateral eye. Involvement of the temporal crescent is the only situation in which a retrochiamsal lesion results in a monocular visual field defect. (Sparing of the temporal crescent in the setting of a homonymous hemianopia is also possible.)
Optic Neuropathy
Vision loss that is only present in one eye is generally either an ocular disorder affecting the structures of the eye itself (above) or an optic neuropathy, a lesion of the optic nerve. There are many different kinds of ischemic, inflammatory, infectious, infiltrative, demyelinating, and toxic-metabolic causes of optic neuropathy (only a few are discussed here), but in all cases of optic neuropathy, there should be decreased visual acuity, decreased color vision, a RAPD, and visual field defects.
Optic Neuritis Optic neuritis is a specific demyelinating optic neuropathy, which may be idiopathic, but is strongly associated with multiple sclerosis (see Chapter 11). Optic neuritis typically occurs in young adults (ages 20 to 40) and is characterized by decreased vision in one eye that presents subacutely (over hours to days) accompanied by a relative afferent pupillary defect. Pain or discomfort exacerbated by eye movement is present more than 90% of the time. Patients with optic neuritis usually note poor color vision in the affected eye. The classic visual field defect associated with optic neuritis is a central scotoma, though other patterns are frequently seen. Funduscopic examination may reveal mild optic disc swelling or may be completely normal.
Optic neuritis is a clinical diagnosis; MRI of the orbits is not necessary when the presentation is classic (a young adult with subacute vision loss in one eye associated with decreased color vision and pain on eye movements). MRI of the brain, however, is useful as a way to identify patients at higher risk to go on to develop multiple sclerosis (MS). Patients with a single episode of optic neuritis who have demyelinating lesions on brain MRI are much more likely to go on to develop MS in the future than patients whose initial brain MRI shows no lesions.
The prognosis for recovery from optic neuritis is excellent. Most patients recover on their own. Intravenous corticosteroids may speed up the recovery of vision but do not provide any lasting benefit. Oral corticosteroids should not be used to treat optic neuritis due to an apparent increased risk of recurrence of optic neuritis.
▪ SPECIAL CLINICAL POINT: Optic neuritis should not be treated with oral corticosteroids, which provide no benefit and may increase the risk of recurrence.
Ischemic Optic Neuropathy Ischemia to the optic nerve head may result in sudden, painless loss of vision. Ischemic optic neuropathy may occur in adults over the age of 50, particularly those with vascular risk factors. Patients often
wake up with vision loss. Decreased visual acuity, decreased color vision, a RAPD, and visual field defects (often altitudinal defects) are the norm. The optic disc is swollen (often with peripapillary hemorrhages) in anterior ischemic optic neuropathy (AION) but appears normal in posterior ischemic optic neuropathy (PION). Anterior ischemic optic neuropathies are further subdivided into arteritic AION (caused by giant cell arteritis) and nonarteritic AION (NAION). Ischemic optic neuropathy can usually be differentiated from optic neuritis by clinical features, such as the age of the patient, the acuity of the vision loss, the presence or absence of pain, and the presence or absence of disc swelling.
wake up with vision loss. Decreased visual acuity, decreased color vision, a RAPD, and visual field defects (often altitudinal defects) are the norm. The optic disc is swollen (often with peripapillary hemorrhages) in anterior ischemic optic neuropathy (AION) but appears normal in posterior ischemic optic neuropathy (PION). Anterior ischemic optic neuropathies are further subdivided into arteritic AION (caused by giant cell arteritis) and nonarteritic AION (NAION). Ischemic optic neuropathy can usually be differentiated from optic neuritis by clinical features, such as the age of the patient, the acuity of the vision loss, the presence or absence of pain, and the presence or absence of disc swelling.
▪ SPECIAL CLINICAL POINT: In acute monocular vision loss, ischemic optic neuropathy is more likely that optic neuritis if (1) the vision loss presents very suddenly (within seconds or minutes), (2) the vision loss is completely painless, or (3) funduscopy reveals pronounced swelling of the optic disc with disc hemorrhages.
Amaurosis Fugax
Transient, painless monocular vision loss (amaurosis fugax) lasting for minutes is highly suggestive of an embolic transient ischemic attack (TIA) related to internal carotid artery disease. Patients often describe the vision loss as a “shade coming down” over one eye. As with any other form of TIA, an urgent vascular workup is recommended, given a greatly increased risk of stroke in these patients (see Chapter 7).
Papilledema
Disc swelling secondary to increased intracranial pressure, unlike disc swelling from optic neuropathies, is often associated with surprisingly mild visual symptoms at first. Papilledema may be accompanied by headache, pulsatile tinnitus, transient visual obscurations, or horizontal double vision—all signs of increased intracranial pressure. The workup of papilledema includes imaging (to rule out a mass lesion, hemorrhage, or hydrocephalus), lumbar puncture (to confirm the presence of increased intracranial pressure), and visual fields (to track any subclinical visual field loss).
The terms pseudotumor cerebri and idiopathic intracranial hypertension are sometimes used interchangably if no structural lesion is found on imaging, although the latter term should probably be reserved for cases in which no explanation for the increased intracranial pressure can be found. Known causes of “secondary” intracranial hypertension include a variety of medications (e.g., retinoids and tetracycline antibiotics), sleep apnea, cerebral venous thrombosis, and dural arteriovenous malformations. Patients with truly idiopathic intracranial hypertension are almost always young, overweight women who benefit from weight loss, but may require medical and surgical interventions to prevent vision loss.
Diplopia
Sometimes patients present complaining of “blurred vision” but actually have diplopia (double vision). The first question to ask in such a situation is, “Does the blurred vision go away if you close either eye?”
If the blurred or double vision resolves when either eye is closed, the patient has binocular diploia (only present when both eyes are open), a sign of ocular misalignment or strabismus (see section Vertical Double Vision and Horizontal Double Vision).
If the blurred vision goes away if one eye is covered but is still present when the other eye is covered, the patient has monocular diplopia (only present in one eye). For example, if the blurred or double vision goes away if the right eye is covered, but is present when the left eye is covered, the source of the monocular diplopia is the right eye.
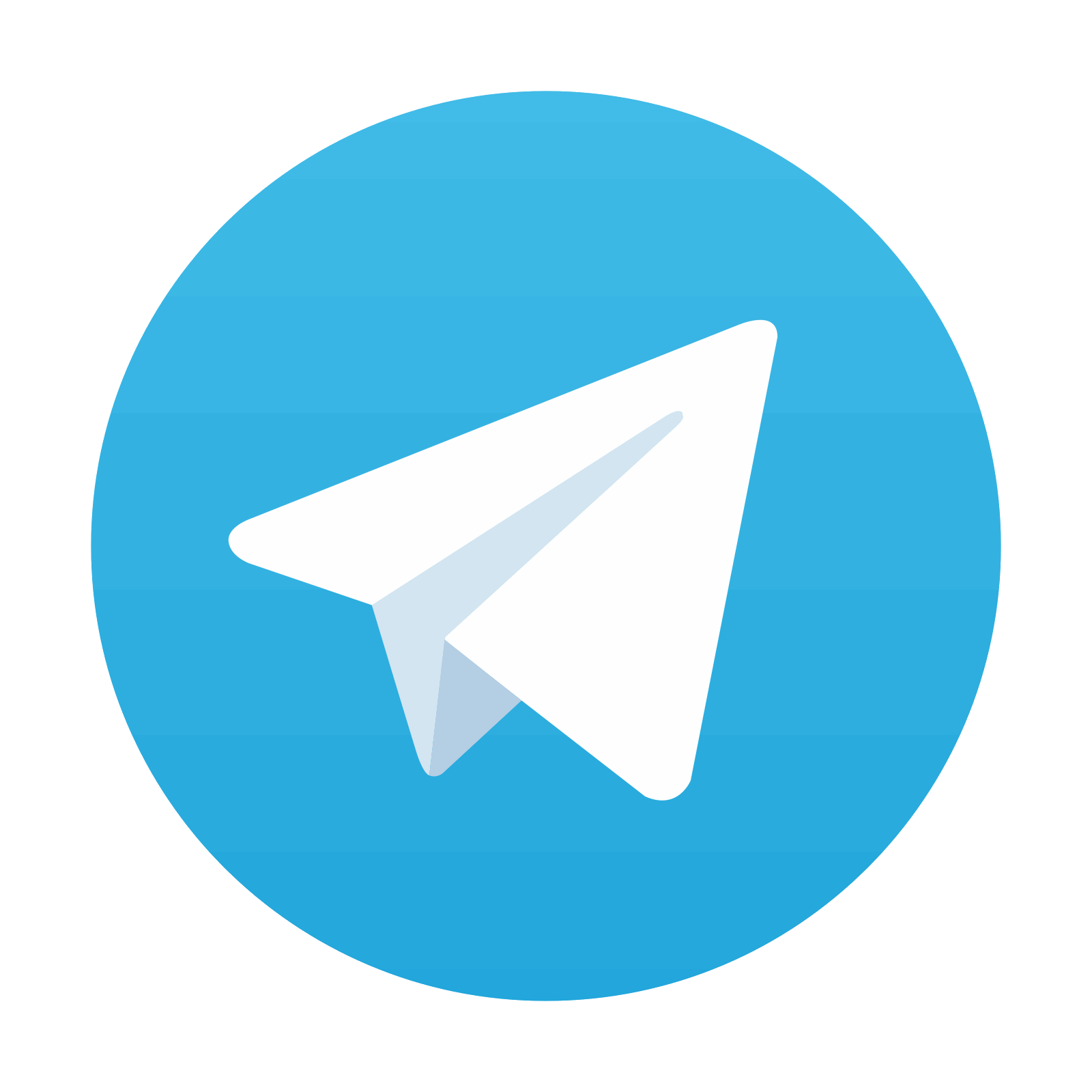
Stay updated, free articles. Join our Telegram channel

Full access? Get Clinical Tree
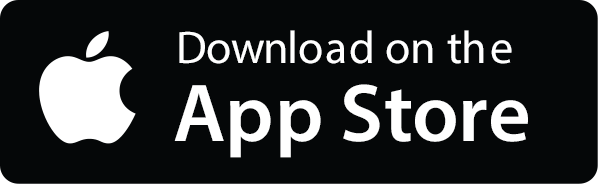
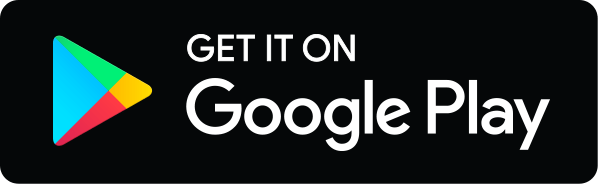