Figure 11.1. Mesial and lateral aspects of the left hemisphere: schematic representation of the three motor areas and their approximate relationship to the surface hemispheric anatomy. The shaded area corresponds to the primary motor cortex (Brodmann area 4). The stippled area illustrates the supplementary sensorimotor cortex on the mesial aspect. On the lateral view, the stippled area represents the premotor cortex. Also note the approximate location of the frontal eye field (hatched area).
The prefrontal and orbitofrontal cortices, as well as the dorsolateral and mesial frontal cortices anterior to the SSMA, are not considered part of the motor cortex. The term prefrontal cortex is used to define the extensive part of the frontal lobe that lies anterior to the motor and premotor zones (12). Modern anatomic and physiologic studies in humans and primates challenge the traditional division of motor areas (13). For example, part of the cingulate cortex, which was previously linked to the limbic system, is now considered as a fourth main motor area, the so-called cingulate motor area (14,15).
Activation studies using positron emission tomography (PET) or functional magnetic resonance imaging (fMRI) allude to the complex organization of the motor system. The breadth of cortical and subcortical areas activated with even the simplest movements attests to the wide distribution and extent of interconnected neural networks underlying motor control (14). Observed movements presuppose a series of parallel or sequential processes involving the selection, planning, preparation, and initiation of action (14,16).
Efferent and Afferent Connections
The familiar hierarchical model of motor control is based on the four levels of spinal cord, brainstem, PMA, and PreMC SSMA. This concept has influenced our understanding of the various motor manifestations of seizures (17). Motor commands are organized hierarchically from the most automatic (e.g., deep tendon reflexes) to the least (e.g., skilled and precise voluntary movements). Each level of motor control retains a somatotopic organization and receives peripheral sensory information that is used to modify the motor output at that level (18). The cerebral cortex exerts its motor control by way of the corticospinal and corticobulbar pathways. The cortex also modulates the action of motor neurons in the brainstem and spinal cord indirectly through its influence on the brain’s various descending systems.
To this day, limited direct information exists about specific neuronal connections between functional brain regions of the human cortex (19). Our knowledge of detailed connectivities is derived from invasive tracer studies in primates. Recent imaging advances are now permitting noninvasive studies of neuronal connections in humans, using the techniques of diffusion tensor imaging (DTI) and functional connectivity MRI (fcMRI)—the latter technique aims to identify highly correlative BOLD signal changes present in different regions of the cortex during the resting state or during specific tasks (20). Importantly, cortical regions identified by fcMRI may reside at a considerable distance from each other. Optogenetic techniques now also allow further in vivo mapping of interactions of motor and premotor areas in animal models (21).
Brainstem Motor Efferents
The brainstem gives rise to several descending motor pathways, which are divided into ventromedial and dorsolateral groups (22,23). The ventromedial system sends fibers through the ventral columns of the spinal cord and terminates predominantly in the medial part of the ventral horn, which contains the motor nuclei controlling proximal limb and axial muscle groups. In contrast, the dorsolateral system descends in the lateral part of the spinal cord and terminates on the lateral motor cell complex, which innervates more distal limb muscles (24). Thus, the dorsolateral motor system places its emphasis on muscles devoted to fine motor control.
Motor Cortex Efferents
The axons that project from layer V of the cortex to the spinal cord run together in the corticospinal tract (a massive bundle of fibers containing approximately 1 million axons). About one-third of corticospinal and corticobulbar fibers arise from the PMA. Another third originate from the SSMA and PreMC, and the rest have their origin in the parietal lobe (arising mainly from the somatosensory cortex of the postcentral gyrus) (25). The corticospinal fibers together with the corticobulbar fibers run through the posterior limb of the internal capsule to reach the ventral portion of the brainstem and send collaterals to the striatum, thalamus, red nucleus, and other brainstem nuclei (26). Many of these relationships can now be visualized noninvasively using combined fMRI and DTI techniques (Fig. 11.2). In the brainstem, the corticobulbar fibers terminate bilaterally in cranial nerve motor nuclei (either directly via a monosynaptic route or indirectly), with the exception of motor neurons innervating the lower face, which receive mostly contralateral corticobulbar input. About three-fourths of the corticospinal fibers cross the midline in the pyramidal decussation at the junction of the medulla and spinal cord and descend in the spinal cord as the lateral corticospinal tract (19). Uncrossed fibers descend as the ventral corticospinal tract. The lateral and ventral divisions of the corticospinal tract terminate in approximately the same regions of spinal gray matter along with corresponding brainstem-originating pathways. The majority of corticospinal tract terminals project on spinal interneurons. An estimated 5% of the fibers synapse directly on (both alpha and gamma) motor neurons (27).
Figure 11.2. Combination of DTI, fMRI, and anatomic imaging demonstrating relationship of the left corticospinal tract to the motor regions using a finger-tapping paradigm. The fMRI and anatomic images are displayed as cut planes, with 3D superimposition of a surface representation of DTI tracks. A seed region for deterministic tracking is placed in the central pons—from this structure, the tracks ascend superiorly through the internal capsule and corona radiata to terminate in pericentral cortex. Note the expected close approximation of central tracks to the hand area.
These anatomic arrangements of descending tracts underlie the contralateral and/or bilateral motor manifestations of focal seizures arising from the motor cortex (17).
Motor Cortex Afferents
The major cortical inputs to the motor areas of cortex are from the prefrontal and parietal association areas (18). These are focused mainly on the PreMC and the SSMA, whereas the PMA receives a large input from the primary somatosensory cortex (28). In addition, the PMA receives direct and indirect inputs from the PreMC and the SSMA. In particular, the SSMA projects bilaterally to the primary motor cortex in a somatotopically organized manner. Other corticocortical inputs arrive from the opposite hemisphere through the corpus callosum (Fig. 11.3), which interconnects heterotopic as well as homologous areas of the two hemispheres (29,30). The major subcortical input to the motor cortical areas comes from the thalamus, where separate nuclei convey modulating inputs from the basal ganglia and the cerebellum (28).
Figure 11.3. Example of corticocortical connectivity using probabilistic tractography from high-resolution DTI images. One seed region is placed in one motor area, and the target region is placed in the contralateral motor area. Computed tracts course across the corpus callosum as expected. The tracts are displayed as a red 3D overlay on black and white anatomic cut planes.
Stimulation Studies
In clinical practice, insights into the functional anatomy of the motor cortex and other eloquent brain cortical areas are afforded by direct cortical stimulation. At the same time, electrical stimulation of the human cortex provides an experimental model that can be used to reproduce the effects of cortical activation after an ictal discharge (31). Several groups use cortical stimulation to trigger habitual auras and/or seizures in an attempt to better delineate the ictal onset zone before epilepsy surgery.
In general, the observed clinical response is assumed to arise from cortex below the stimulated electrode or from the region between two closely spaced electrodes, given that the current density drops off rapidly with increasing distance from the tissue underlying the stimulated electrode (32,33). Electrical stimulation can elicit “positive” responses (such as localized movements resulting from activation of the PMA or SSMA cortex) or “negative” responses (such as inhibition of motor activity). The latter becomes apparent only if the patient engages in specific tasks during stimulation. In areas such as the supplementary motor cortex, both positive responses in the form of bilateral motor movements and negative responses such as speech arrest can be demonstrated. The area of stimulation gives rise to distinctive patterns of motor activation of the PMA, SSMA, or premotor regions. Overlapping clinical manifestations are commonly observed as a result of the highly developed interconnectivity between these regions (34).
Negative motor responses interfere with a person’s ability to perform a voluntary movement or sustain a voluntary contraction when cortical stimulation is applied (35). The patient is unaware of the effects of stimulation unless asked to perform the specific function integrated by the stimulated cortical region. In a systematic review of 42 patients who had subdural electrodes over the perirolandic area, the Cleveland Clinic group observed negative motor responses over both hemispheres, when stimulating the agranular cortex immediately in front of the primary and supplementary face areas (35). To distinguish the two negative motor areas, investigators proposed the terms primary negative motor area (PNMA, in regard to the region of the inferior frontal gyrus immediately in front of the face PMA) and supplementary negative motor area (SNMA, in reference to the mesial portion of the superior frontal gyrus immediately in front of the face SSMA). Other investigators have been able to confirm and extend these observations using similar techniques of direct cortical stimulation with subdural electrodes, and have concluded that negative motor areas are in fact widely distributed throughout the perirolandic region and within the PreMC (36). These areas are considered a part of the cortical inhibitory motor system, the epileptic activation of which may give rise to focal inhibitory motor seizures (also referred to by some authors as “akinetic” seizures). Such seizures can be easily overlooked because patients may remain unaware of their weakness and/or inability to execute specific movements, unless they are carefully examined.
The effects of functional localization and effects of electrical stimulation in the three broad motor areas are briefly discussed below.
Primary Motor Area
The PMA resides in the anterior wall of the central sulcus (see Fig. 11.1) and corresponds to Brodmann area 4. On the basis of cytoarchitectonic criteria, area 4 is recognized primarily by the presence of Betz cells (giant pyramidal cells) in cortical layer V and the absence of a granular layer IV (37). The central sulcus marks the border between the agranular motor cortex and the granular somatosensory cortex (38).
Radiographically, the central sulcus appears as a prominent, almost always continuous, sulcus, which extends from the mesial aspect (near the brain’s apex) along an oblique coronal trajectory toward (and near to) the sylvian fissure. The superior and inferior aspects of the central sulcus are terminated by the paracentral lobule and subcentral gyrus, respectively, which effectively appear as a joining of the pre- and postcentral gyri. The radiographic identification of the central sulcus is often critical to the interpretation of imaging studies and planning surgical procedures, as it provides a central landmark from which other topologies can be located. There are several characteristic features identifying the central sulcus, three of which are shown in the cartoon of Figure 11.4 and on the T1-weighted MRI images of Figure 11.5. Most easily identified is the so-called hand knob, which assumes the form of an upside-down omega (“Ω”) on axial images (39). Formed by a relative gyral hypertrophy corresponding to the PMA of the hand, the “hand knob” appears to project posteriorly from the precentral gyrus into the contour of the central sulcus. Due to anatomic variation, this feature sometimes assumes the shape of a horizontal epsilon (“ε”), rather than the inverted omega (39). Another confirmatory feature of the “hand knob” on the sagittal plane is that it appears as if forming a backward “hook” (see Fig. 11.5), but this appearance is less reliable than is the characteristic axial morphology. A second helpful landmark is the topology of the superior central gyrus, which is easily seen running along an anterior–posterior direction along the medial frontal lobe and whose posterior margin is the precentral gyrus. Identification of the precentral gyrus is further aided by demarcation of the pre- and postcentral sulci. Lastly, axial images nicely portray the pars marginalis, which is the ascending ramus arising from the posterior cingulate sulcus. The left and right ascending rami appear on axial images as bilaterally paired paramedian features that together form the shape of a “bracket” or “smile” (40). This characteristic appearance is often preserved over multiple axial slices and can be used to identify the central sulcus and differentiate it from the adjacent postcentral sulcus.
Figure 11.4. Key features for identifying the sensorimotor cortex. The essential step is identification of the central sulcus (CS), which separates the precentral gyrus anteriorly (motor cortex) from the postcentral gyrus posteriorly (sensory cortex). This schematic of an axial section illustrates three classic features that aid in the identification of the CS. (1) the “hand knob” is a posterior protuberance of the precentral gyrus, which corresponds to relative “hypertrophy” of the primary hand motor area. The shape of the sulcus in this area is often described as that of an upside-down omega (“Ω”). (2) The anterior–posterior orientation of the superior frontal gyrus is often easily identified, as it aligns along a paramedian plane. While the anterior margin of the SFG extends to the frontal poles, the posterior margin merges with the precentral gyrus, such that the most posterior margin often appears as the central sulcus. (3) Posterior to the medial aspect of the central sulcus is the pars marginalis, which often has a slight concavity—when pairing together, the left and right pars marginalis assume the characteristic appearance that resembles a “smile” or “bracket,” which can be seen on multiple axial sections, making identification easy. (IFG, inferior frontal gyrus; MFG, middle frontal gyrus; SFG, superior frontal gyrus; Pre-CG, precentral gyrus; post-CG, postcentral gyrus; CS, central sulcus; PM, pars marginalis.)
Figure 11.5. MRI examples of the landmark features described in Figure 11.4, which help locate the central sulcus. Shown as overlaid white line segments are the “omega” of the hand knob (left image) and the pars marginalis “smile” (middle image). The middle image also demonstrates the architecture of the superior frontal gyrus terminating posteriorly in the precentral gyrus. The right image displays the backward “hook” as described in the text—this feature is appreciated on sagittal images passing through the hand knob.
The somatotopic organization of the PMA was elucidated by the pioneering work of Krause (9), Penfield, Jasper, and Rasmussen (41,42), and others (Fig. 11.6). In this region of the PMA, simple movements were elicited with the lowest intensity of electrical stimulation (43). The resulting motor maps show an orderly arrangement with the tongue and lips near the sylvian fissure and the thumb, digits, arm, and trunk represented successively along the central sulcus, ending with the leg, foot, and toes on the mesial surface. The somatotopic organization of the motor cortex is not fixed and can be altered during motor learning or after injury (44). The layout of the motor homunculus is topographically similar to that of the somatosensory homunculus, which resides immediately behind the PMA. Contemporary noninvasive methods, such as BOLD imaging fMRI, nicely confirm and recapitulate the classic homunculus (see Fig. 11.6). Output of the PMA is directed to the corticospinal and corticobulbar tracts, as well as to the SSMA and homologous areas in the opposite hemisphere via the corpus callosum (45).
Figure 11.6. The motor homunculus after Penfield and Rasmussen depicting the somatotopic arrangement of the primary motor cortex (PMA) (with the tongue and lips near the sylvian fissure and the thumb, fingers, arm, and trunk represented successively along the precentral gyrus ending with the leg, foot, and toes on the mesial surface) occupies the center of this figure. Muscle groups involved in fine movements feature a disproportionately large representation. Surrounding this schematic representation of the motor homunculus are corresponding images of fMRI activation of the primary motor cortex obtained with eight different motor tasks—the fMRI images nicely recapitulate the classic motor homunculus. Images are provided in coronal oblique reformatted planes that are roughly parallel to the motor strip. Significant activity is shown as color overlay. The toe, knee, shoulder, and finger tasks employed flexion/extension or tapping at a rate of about 2 per second, using the right-sided limb only. The eye blink, lip (pursing), and tongue (pressing against palate) tasks were bilateral motions performed at a similar rate. Right lower extremity movements are clearly localized along the left superomedial cortical surfaces, with right upper extremity movements localized along left superolateral cortical surfaces. Note bilateral motions from the eyes, lips, and tongue show corresponding bilateral activation. (Adapted from Penfield W, Rasmussen T. The Cerebral Cortex of Man—A Clinical Study of Localization of Function. New York: The MacMillan Company; 1950, with permission.)
Stimulation Studies
In the PMA, single stimuli typically elicit single clonic movements of the contralateral somatic muscles represented by the area of the motor homunculus being stimulated. High-frequency (50 to 60 Hz) stimulus series result in slower, tonic contralateral motor responses (46). Intraoperative application of electrical stimulation mapping under local or general anesthesia provides the most direct and easy way to localize the perirolandic cortex in most adults (47). When local anesthesia is used, motor responses are usually evoked with currents of 2 to 4 mA. Sensory responses are elicited with stimulation of the postcentral gyrus, often at slightly lower thresholds (48). The threshold for eliciting a motor response in humans is lowest in the PMA. Electrical cortical stimulation studies uncover the individual variability in the topographic organization of sensorimotor maps in humans with structurally normal anatomy (49). The importance of direct cortical stimulation studies in patients with lesions and/or epileptogenic foci encroaching on the sensorimotor cortex cannot be overemphasized (50). Furthermore, bilateral probabilistic map has proven to become a useful tool to map and anticipate cortical epicenters of human brain functions, including motor and language areas (51).
Supplementary Sensorimotor Area
The SSMA is a distinct anatomic region located on the mesial surface of the superior frontal gyrus and its adjacent dorsal convexity (52). The cerebral cortex of the SSMA corresponds to the mesial portion of area 6 of Brodmann cytoarchitectonic map of the brain (41,53) (see Fig. 11.1). Phylogenetically, the SSMA may be viewed as older motor cortex derived from the anterior cingulate periarchicortical limbic system (54). Similar to the primary motor cortex, the SSMA is referred to as agranular cortex, because the internal granular layer (layer IV) is not prominent. In contrast to area 4, area 6 does not contain Betz cells (53). The medial precentral sulcus defines the border between the PMA for the foot and the posterior limit of the SSMA (13,55). No clear cytoarchitectonic or anatomic boundary separates the SSMA from the adjacent PreMC (56).
The macaque and human mesial area 6 (SSMA) is further subdivided into pre-SSMA (rostrally) and SSMA proper (caudally) on the basis of comparable cytoarchitectonic and transmitter receptor studies (38). Studies in primates suggest that the pre-SSMA holds a hierarchically higher role in motor control. The functional properties of the SSMA subdivisions have not been detailed in humans (57). The border between the pre-SSMA and SSMA proper corresponds to the vertical anterior commissure line (VAC line, i.e., the vertical line passing through the anterior commissure and perpendicular to the AC–PC line, which connects the anterior and posterior commissures) (Fig. 11.7). The border between SSMA proper and PMA corresponds approximately to the VPC line (i.e., the vertical line that traverses the posterior commissure and is perpendicular to the AC–PC line) (58).
Figure 11.7. Functional MRI using a finger-tapping paradigm (paramedian sagittal plane) shows activation of the supplementary sensorimotor area (SSMA), which lies within the paracentral lobule and is located anteriorly to medial margin of the central sulcus. In addition, cerebellar activation is also present. Note the anatomical relationships of the SSMA to the AC–PC line, as described in the paragraph on Supplementary Motor Area, above. The border between the pre-SSMA anteriorly and SSMA proper corresponds to the VAC line, and the border between SSMA proper and PMA posteriorly corresponds approximately to the VPC line. AC–PC refers to the line that connects the anterior and posterior commissures, VAC (or VCA as depicted above) refers to the vertical line passing through the anterior commissure and perpendicular to the AC–PC line, VPC (or VCP as depicted above) refers to the vertical line that traverses the posterior commissure and is perpendicular to the AC–PC line. (CS, central sulcus; PM, pars marginalis.)
Stimulation Studies
More than 70 years ago, Foerster was the first to describe motor responses in humans elicited by electrical stimulation of the mesial aspect of the superior frontal gyrus anterior to the primary motor representation of the lower extremity (2). Systematic study of this region with electrical stimulation was carried out at the Montreal Neurological Institute (MNI) during the intraoperative evaluation of patients with intractable focal epilepsy preceding surgical resection (59).
This was the first group to use the term supplementary motor area (SMA). Direct intraoperative electrical stimulation of the SMA produced vocalization, speech arrest, postural movements of all extremities, inhibition of voluntary movements, paresthesias, and autonomic changes. The rich repertoire of combined or postural movements included the so-called fencing posture, a term coined by the MNI group to describe the classical stimulation-induced postural response that consists of elevation of the contralateral arm with the head and eyes turned toward the raised hand (41). The Montreal studies demonstrated that both positive (such as bilateral motor movements) and negative responses (such as speech arrest) could be elicited by stimulating this region. The intraoperative study of the mesial interhemispheric surface carries significant limitations, because of the tedious and potentially dangerous surgical approach (in proximity to the superior sagittal sinus and its cerebral bridging veins), the restricted amount of time, and the relative difficulty in recognizing the specific gyral landmarks during surgery in this region.
With the advent of subdural electrodes, the Cleveland Clinic series of extraoperative stimulation studies showed that positive motor responses were not restricted to the mesial aspect of the superior frontal gyrus, but could also be elicited from its dorsal convexity, the lower half of the paracentral lobule, and the precuneus (60). The same group confirmed the presence of sensory symptoms that were elicited along with the positive motor responses after stimulation of the SMA and coined the term SSMA instead of SMA.
Using depth electrodes, Talairach and Bancaud (61) were the first to describe a somatotopic organization within the SSMA. The Yale group confirmed the presence of somatotopic distribution in the SSMA, where the face, upper extremity, and lower extremity responses are oriented in a rostrocaudal direction, with the lower extremities represented posteriorly, head and face most anteriorly, and the upper extremities between these two regions (62). Likewise, studies of movement-related potentials (MRPs) using subdural electrodes implanted over the SSMA region demonstrated that MRPs for different types of movements (finger, foot, tongue, vocalization, etc.) also have a somatotopic distribution within the SSMA, which is consistent with the organization defined by electrical stimulation (63–65). Notably, fMRI studies using motor activation paradigms, such as finger tapping, for example, demonstrate strong activations of the SSMA in addition to the primary motor cortex (see Fig. 11.7).
Premotor Cortex
Fulton coined the term PreMC in 1935 to describe the third major component of motor cortex (66). This area encompasses the more loosely defined agranular cortex of the lateral frontal convexity rostral to the PMA (11,23), which corresponds to the lateral portion of Brodmann area 6 (see Fig. 11.1). It is difficult to define the anterior border of the agranular PreMC in humans, where a broad zone of progressive transition exists between area 6 and the granular cortex of Brodmann frontal area 9 (67). In the macaque, the PreMC is further subdivided into a dorsal portion on the dorsolateral convexity and a ventral portion on the ventrolateral convexity (11). Despite the lack of direct correlation between microstructure and function in humans, the two subdivisions of the premotor area are considered to have homologous counterparts in the human brain. The motor and premotor cortices, as well as the frontal eye fields (FEFs) and the anterior cingulate cortex of area 24, have reciprocal connections with the SSMA (53). Anatomic labeling experiments in the macaque have demonstrated that the more anterior dorsal PreMC projects to the spinal cord, challenging the notion that the PreMC, unlike the PMA and SSMA, lacks prominent corticospinal connections (23,68,69).
According to the classic schema, the PreMC is responsible for the preparation and organization of movements (56). Several recent studies show that the PreMC also plays a central role in nonmotor attentional and receptive domains. Therefore, our current understanding suggests a dual PreMC function pertaining to motor and cognitive behaviors (70).
Stimulation Studies
On the basis of early electrical stimulation studies of the monkey brain (71), the agranular lateral PreMC (area 6) has been subdivided into a rostral section (6aβ or 6r) and a caudal section (6aα or 6c). Recent quantitative architectonic and neurotransmitter studies have corroborated the presence of similar topographic boundaries in the human brain (38,67). The rostral subdivision covers the anterior part of the precentral gyrus, and its caudal counterpart resides in the posterior part of the superior and middle frontal gyri, in front of the precentral sulcus (72).
Eye movements can be electrically induced from a large area of the human dorsolateral frontal cortex and the precentral gyrus. These stimulation-elicited responses have been attributed to electrical interference with the human homolog of the monkey FEF (73). Electrical stimulation studies in humans have confirmed the functional location of the eye movement sites anterior to the motor representation of the arm and face (73,74). However, some ambiguity exists as regards the exact location of the human FEF within this rather extensive oculomotor region. The divergence is largely caused by the methodologic differences of neuroimaging and electrical cortical stimulation studies.
The electrically defined human FEF is located in the posterior end of the middle frontal gyrus (see Fig. 11.1) immediately anterior to the precentral sulcus (and in proximity to the superior frontal sulcus). Electrical cortical stimulation of this area produces constant oculomotor responses characterized by low stimulation thresholds (73). Conversely, neuroimaging studies of cerebral blood flow (CBF) changes suggest that the homologous region in humans lies posterior to the electrically defined FEF. Indeed, the CBF-defined FEF is located between the central and precentral sulci in front of the primary hand representation, suggesting that the eye movement field lies in Brodmann area 6 (i.e., in a PreMC region homologous to the ventral PreMC) (75,76). More recently, corticocortically evoked potentials have been used to evaluate connections between the premotor and motor cortex in humans implanted with subdural electrodes (77).
FOCAL MOTOR SEIZURES
Focal seizure is the term proposed by the Task Force of the International League Against Epilepsy (ILAE) to describe seizures in which the initial activation involves a limited number of neurons in part of one hemisphere (78). The terms localization- related or partial seizures have been used to describe the same seizure type. However, the more recently proposed diagnostic scheme of the ILAE Task Force prefers the less ambiguous term focal to partial or localization-related seizures (79).
Motor phenomena constitute the main clinical manifestations of motor seizures. As a rule, consciousness is retained in the majority of seizures arising from discrete motor regions. It is possible, however, for an ictal discharge to remain localized and still produce alteration of consciousness. Furthermore, certain motor manifestations and a patient’s anxious reaction to the seizure symptoms may prevent the patient from responding appropriately during seizures. It may, therefore, be difficult to ascertain the level of consciousness in several patients with focal motor seizures. In the past, the presence or absence of altered awareness was used to dichotomize seizures of focal onset into “simple partial” and “complex partial.” It is now proposed to move away from this dichotomy, which seems to have “lost its meaningful precision” (79).
Subsequently, the ILAE provided a glossary of descriptive terminology to describe ictal semiology (80). Ictal motor phenomena may be subdivided into elementary motor manifestations (such as tonic, clonic, dystonic, versive) and automatisms. Automatisms consist of a more or less coordinated, repetitive motor activity (such as oroalimentary, manual or pedal, vocal or verbal, hyperkinetic or hypokinetic) (80). Somatotopic modifiers may be added to describe the body part producing motor activity during seizures.
Another recent seizure classification is based on the clinical symptomatology and is independent of electroencephalographic (EEG), neuroimaging, and historical information (81). This classification uses terms such as focal clonic, focal tonic, or versive, and evolution during the seizure is indicated by arrows. For example, left-hand somatosensory aura → left arm clonic seizure → left versive seizure.
Clinical Semiology
This section reviews the elementary motor phenomena resulting from a variety of focal motor seizures. These seizures typically present with clonic or tonic manifestations. Hyperkinetic manifestations are usually attributed to seizures arising from (or spreading to) the frontal lobe. Other motor automatisms seen with focal seizures (such as oroalimentary, mimetic, or gestural automatisms) are reviewed elsewhere.
In a population-based study conducted in Denmark of 1054 patients with epilepsy who were between the age of 16 and 66 years, 18% had “simple partial” seizures (82). Mauguiere and Courjon examined the presenting seizure type in a large series of 8938 patients with seizures admitted to a single hospital over a 10-year period. They found that 1158 patients (12.9%) had focal tonic or clonic seizures without march (the most common presentation of focal seizures in this series); 582 patients (6.5%) presented with hemitonic or clonic seizures; 461 (5.2%) had adversive seizures; and only 199 (2.2%) had jacksonian seizures (83). Perirolandic epileptogenic lesions often involve both the precentral and postcentral gyri, giving rise to both motor and sensory phenomena. In one video–EEG study of 14 patients with a total of 87 “simple partial” seizures, sensory phenomena were observed in approximately one-third of patients exhibiting focal motor seizures (84).
Postictally, patients may experience a transient functional deficit, such as localized paresis (Todd paralysis), which may last for minutes or hours (up to 48 hours or longer). This interesting clinical phenomenon of “postepileptic paresis” is the signature of a focal seizure and bears the name of Dr. Bentley Todd, who first described it in the mid-19th century (85). Todd paralysis is believed to result from persistent focal dysfunction of the involved epileptogenic region. Postictal Todd paralysis is a clinical sign of substantial value in lateralizing the hemisphere of seizure onset (86).
Clonic Seizures
Clonic seizures consist of repeated, short contractions of various muscle groups characterized by rhythmic jerking or twitching movements (87). These movements recur at regular intervals of <1 to 2 seconds. Most clonic seizures are brief and last for <1 or 2 minutes. During this period, clonic movements may remain restricted to one region or spread in a jacksonian manner. The majority of focal motor seizures tend to involve the hand and face, although any body part may potentially be affected (88). Such predilection is attributed to the large cortical representation of the hand and face area. The typical manifestation of a localized discharge within the precentral gyrus is clonic twitching of specific contralateral muscle groups, as determined by their proportionate somatotopic representation.
The clonic movements are usually limited to the corresponding area of the body, but may spread during the attack. Such spread (e.g., from the muscles of the face to the ipsilateral hand or arm) is known as the “jacksonian march.” During these “jacksonian attacks,” motor symptoms travel slowly from one territory to another, typically following the order of the corresponding somatotopic representation. The term jacksonian seizures was proposed by Charcot in 1887 to describe the characteristic march seen with this particular subtype of clonic seizures (89). The continued use of the term serves to remind us of Hughlings Jackson’s astute clinical observations, which provided the basis for his revolutionary principles of functional localization long before the era of EEG and neuroimaging correlations (90). In his own words:
“The part of the body where the convulsion begins indicates the part of the brain where the discharge begins and where the discharging lesion is situated. But from the focus discharging primarily the discharge spreads laterally to the adjacent “healthy” foci. One focus after the other is seized by the radiating waves of impulses. The march of the attack, the order in which the different parts of the body become involved, reveals the arrangement of the corresponding foci in the precentral convolution.” (2)
The march usually starts from a distal body region (such as the thumb, fingers, great toe, mouth, or eyelids) and spreads toward a more proximal part. Jackson astutely described three variants: (i) “fits starting in the hand (most often in the thumb or both),” (ii) “fits starting in one side of the face (most often near the mouth),” and (iii) “fits starting in the foot (nearly always in the great toe)” (3). Typically, consciousness remains intact, and secondary generalization of jacksonian seizures is uncommon. At times, the march may skip some areas, a phenomenon that may be related to different seizure thresholds within the symptomatogenic region. Holowach et al. reviewed 60 jacksonian seizures in children and found that the majority (25 out of 60) began in the face (8 in the periocular and 5 in the perioral region), 17 in the hand, 7 in the arm, 2 in the shoulder, and 9 in the leg and foot (91).
Lastly, the term hemiconvulsions refers to unilateral clonic seizures (i.e., clonic activity affecting one side of the body). Prolonged unilateral convulsions followed by the onset of hemiparesis are described in the childhood syndrome of hemiconvulsion–hemiplegia–epilepsy.
Myoclonus and Myoclonic Seizures
Many types of myoclonic phenomena (e.g., myoclonus caused by spinal cord disease or essential myoclonus) do not have an epileptic origin and need to be differentiated from (focal) myoclonic seizures. Typically, myoclonic jerks are arrhythmic compared with clonic motor activity. Notable exceptions discussed under the broad definition of myoclonus include the more rhythmic motoric manifestations of epilepsia partialis continua (see below, paragraph on Epilepsia Partialis Continua) and the nonepileptic segmental myoclonus or palatal myoclonus (also called palatal tremor) (92).
Epileptic myoclonus is typically accompanied by an EEG correlate of spike- or multispike-and-wave complexes (93,94). Polygraphic recordings combining EEG with electromyography (EMG) of affected muscles may be necessary to unmask the relationship or the epileptic EEG activity to the myoclonic jerk. Video recordings can be helpful, but cannot replace polygraphy in ambiguous cases. The term myoclonic seizure is reserved for epileptic seizures, whose main components are single or repeated epileptic myoclonias (95). Gastaut (96) distinguished epileptic myoclonic events into generalized, segmental, and focal, according to whether the seizures affected the entire body, one or more limbs, ipsilateral body parts/segments, or only one part of a single limb, respectively.
Although an accurate distinction may oftentimes prove difficult, some authors do not consider epileptic myoclonus as part of focal motor seizures (94). Others view focal cortical myoclonus as one manifestation of focal motor seizures, given that myoclonus in this instance results from a hypersynchronous discharge arising from a distinct population of cortical cells within the PMA and/or premotor areas (97). Focal cortical myoclonus has been described in patients with focal lesions involving the motor cortex, such as tumors, trauma, cortical dysplasia, or vascular lesions (Fig. 11.8). In a report of four children with perirolandic cortical dysplasia presenting with focal cortical myoclonus, the authors observed that localization of the recorded epileptiform discharges correlated with the body part affected by the myoclonus (C3 electrode in two patients whose myoclonus involved predominantly the right upper extremity, C3–T7 electrodes in one patient with myoclonus affecting the face, and Cz electrode in the other patient with focal myoclonus of the left leg) (98).
Figure 11.8. Consecutive scalp electroencephalogram tracings (A to C, longitudinal bipolar montage) during a typical stereotyped event do not disclose a definite evolving ictal pattern, in a 39-year-old patient with exquisitely focal motor seizures since childhood. In the last 3 years, she has been experiencing daily very brief seizures involving the muscles of the lower face on the right side without alteration of awareness. There is no history of jacksonian march or secondarily generalized tonic–clonic seizures. Interictal EEG is normal. Although EEG activity is predominantly obscured by the presence of high- voltage myogenic artifact, the sequence of motor manifestations can be discerned from the appearance of this same artifact. A:Clinical onset in the middle of this 10-second page punctuated by tonic contraction of the right facial musculature with involuntary right eye closure and deviation of the jaw toward the right, associated with voluntary reactive tensing of the entire face, as evidenced by the widespread and asymmetric “tonic muscle artifact.” B: Clinical evolution with a brief cluster of repetitive focal myoclonic seizures involving the right side of the lower face, as evidenced by the corresponding repetitive, nonrhythmic, and almost instantaneous muscle artifact. Because of its very high voltage, the artifact appears widespread on this printed page. Digital reformatting confirms that the myogenic artifact is in fact picked up predominantly by the right-sided EEG electrodes, which are adjacent and susceptible to the contracting ipsilateral facial muscles. C:After the end of this motor sequence, the patient experiences three isolated, less intense, but otherwise identical muscle jerks in the span of 5 seconds, which are associated with myogenic artifact of relatively lower voltage (arrows) interrupting the otherwise normal awake EEG recording. Note that in this instance, the terminal muscle jerk is indeed associated with myogenic artifact primarily involving the right-sided derivations. D:Subtraction ictal SPECT (single-photon emission computed tomography) study coregistered to the patient’s MRI, as depicted in these selected axial images, revealed a small, discrete, and isolated area of hyperperfusion in the left precentral region, ventral to the expected location of the hand motor area. The patient’s MRI was normal except for a small, questionable, but concordant (based on clinical semiology, EEG, and ictal SPECT findings) area of faint signal increase in the depth of the left precentral sulcus at the level of the middle frontal gyrus. Surgical pathology revealed a balloon-cell cortical dysplasia. The location of the lesion (in the depth of the sulcus) and the exquisitely focal nature of the patient’s habitual seizures likely account for the lack of interictal and ictal EEG abnormalities in this case.
Finally, the paradoxical term negative myoclonus is reserved for cases of sudden, brief relaxations in tonic muscle contraction (92). Negative myoclonus (which also encompasses the phenomenon of asterixis typically seen in toxic–metabolic encephalopathies) is a nonspecific manifestation and can be associated with a variety of neurologic disorders. Epileptic negative myoclonus can be either unilateral or bilateral and can be seen in relationship with a number of heterogeneous epilepsies ranging from the benign idiopathic epilepsies to severe epileptic encephalopathies (99).
Tonic Seizures
Tonic seizures consist of sustained muscle contractions that usually last for more than 5 to 10 seconds and result in posturing of the limbs or whole body (100). From the standpoint of clinical semiology, tonic seizures can be described according to the distribution and symmetry of tonic contractions with involvement of the axial (neck, trunk, and pelvis) and limb musculature. Generalized tonic seizures involve axial and limb muscles in a symmetric and synchronous fashion. Unequal or asynchronous contraction of muscle groups involving both sides of the body results in bilateral asymmetric tonic seizures. Contraction restricted to a portion of the body on one side only gives rise to focal tonic seizures (101).
In contrast to focal clonic seizures, which represent epileptic activation of a restricted region of the precentral gyrus, tonic motor seizures may implicate a wider area of motor cortex including the SSMA and the PreMC (17,102). Even though focal tonic seizures are attributed to activation of Brodmann area 6 (and the mesial frontal region in particular), some overlap in symptomatology occurs, with ictal involvement of the premotor and/or PMAs (103).
In a video–EEG study of 481 consecutive patients with focal epilepsy—evaluated at two tertiary epilepsy centers over a period of 4 years—a total of 123 patients were observed to have tonic seizure manifestations during at least one of their video–EEG recorded seizures. The vast majority of these patients had extratemporal epilepsy. Tonic seizures more frequently involved both sides of the body (76% bilateral vs. 24% unilateral). Importantly, when seen at the beginning of the seizure evolution, tonic seizures were more frequently associated with frontal lobe epilepsy as compared to epilepsy arising from the posterior neocortex. Furthermore, auras were more likely to precede tonic seizures originating from the parietooccipital regions and were less frequently reported in frontal lobe epilepsy (104).
Stimulation of the SSMA elicits bilateral, asymmetric tonic contractions affecting primarily the more proximal muscles. Less frequently, focal tonic contractions may be seen. The symptomatogenic zone is less clear in cases of symmetric, bilateral tonic seizures. However, these seizures are believed to be generated by simultaneous bilateral activation of Brodmann area 6, rostral to the precentral region, in both hemispheres (31). It is also possible that generalized tonic seizures result from direct activation of brainstem reticular-activating systems (105,106). The fact that slow-wave and rapid eye movement (REM) sleep, as well as decreased levels of vigilance, appear to facilitate some tonic seizures, for example, in patients with Lennox–Gastaut syndrome, further implicates the brainstem in the generation of these phenomena (107). It becomes evident that different types of tonic seizures utilize different neuroanatomical pathways, which is hardly surprising given that tonic seizures may be a common clinical manifestation resulting from a variety of different pathophysiologies underlying symptomatic and less frequently idiopathic epilepsies.
Nonepileptic focal tonic symptoms can result from subcortical pathology (e.g., spinal cord or brainstem dysfunction in the case of compression or multiple sclerosis). In addition, paroxysmal tonic phenomena may be seen as part of certain movement disorders (such as paroxysmal choreoathetosis or spasmodic torticollis) or other nonepileptic paroxysmal disorders (e.g., in the setting of convulsive syncope) (101).
Oculocephalic Deviation and Versive Seizures
Foerster and Penfield first described versive seizures in 1930. The seizures consist of a sustained, unnatural turning of the eyes and head to one side, as a result of a predominantly tonic contraction of head and eye muscles (108). Although consciousness is often lost by the time a patient experiences version, occasionally, patients may be aware of the forced, involuntary head and eye deviation (45,109).
As discussed, cortical stimulation studies have confirmed the functional location of eye movement sites in proximity to the primary motor representation of the arm and face (74). On stimulating this region, Rasmussen and Penfield (110) observed that the more anterior points were responsible for contralateral rotation. Stimulation of more posterior points (closer to the central sulcus) elicited contralateral, ipsilateral, or upward eye movements. Head rotation was usually seen in conjunction with contralateral eye rotation.
The lateralizing significance of oculocephalic deviation has met with controversy. Indeed, a number of authors use the terms head turning and head version interchangeably (111–113). This lexical ambiguity prompted Wyllie et al. (108) to restrict the term version to “unquestionably forced, involuntary head and eye deviation to one side resulting in sustained unnatural positioning of the head and eyes.” In this important study, the authors reviewed retrospectively all lateral head and eye movements observed during 74 seizures in 37 patients and classified as “nonversive” any mild, unsustained, wandering, or seemingly voluntary head and eye movements. Visual analysis of video recordings was performed without prior knowledge of EEG findings. By adhering to the strict definition of “version,” the authors showed that the presence of a contralateral versive head and eye movement provides reliable lateralizing information (especially when this movement precedes secondary generalization) (108). On the other hand, one should be cautious about interpreting the direction of eye and head turning, if the seizure does not become secondarily generalized (114,115).
Version may result from seizures originating from various locations and spreading to the PreMC. A noteworthy clinical observation is that extratemporal seizures give rise to version earlier in the seizure (within 18 seconds from seizure onset), compared with seizures of temporal lobe origin (in which version is usually seen after 18 seconds or later) (114).
Seizures Manifested by Vocalization or Arrest of Vocalization
Several types of utterances can occur during epileptic seizures. A prolonged continuous or interrupted vocalization may occur in seizures involving the SSMA or lower PMA on either hemisphere (61). Vocalization, when it occurs as part of SSMA seizures, tends to be more sustained (116). Penfield and Jasper produced such phonatory phenomena in humans by stimulating the SSMA or the PMA below the lip or tongue area (41). Finally, the so-called epileptic cry is frequently seen at the onset of generalized tonic–clonic seizures.
Speech arrest, defined as inability to speak during a seizure despite conscious attempts by the patient (45), may result from involvement of the PMA (117) or SSMA in either the dominant or the nondominant hemisphere. Electrical cortical stimulation studies suggest that the speech arrest observed in cases of SSMA stimulation represents a negative motor response (resulting from inhibition of tongue movement) (118).
EEG Findings
The ability of scalp EEG to detect interictal activity depends on the extent of the irritative zone and the orientation of the dipole. Special techniques may be required to demonstrate epileptiform activity in patients with focal seizures. Sleep recordings, for example, have been reported to increase the yield of interictal epileptiform abnormalities (119–121). Special electrodes (such as sphenoidal, anterior temporal, or ear electrodes) and closely spaced additional scalp electrodes (Fig. 11.9A) may help to distinguish temporal from frontal foci and determine whether the electrical field of a midline sharp wave is higher over the left or right hemisphere (122–124).
Figure 11.9. Scalp-EEG tracings (A, B, and C) from a 41-year-old patient with intractable left perirolandic epilepsy of unknown etiology since age 11 years. He presented with daily focal motor seizures involving the right leg and shoulder sometimes preceded by a somatosensory aura (tingling sensation in the right foot) and rare secondarily generalized tonic–clonic seizures. A:Interictal left centroparietal sharp wave as seen on a longitudinal bipolar (upper part) and referential montage (lower part). The P8 reference derivation of the sharp wave shows maximum negativity at electrode CP3. The addition of closely spaced surface electrodes (placed according to the 10–10 electrode placement system) provides for a more accurate distribution map. In this instance, the potential amplitude at electrode C5 is 85% and at electrodes C3 and CP3 is 83% of the amplitude recorded at the maximally involved electrode CP3. B: Periodic epileptiform discharge-like (PLED-like) pattern of left centroparietal sharp waves and polyspikes. This interictal finding was present during the first 24 hours of admission for acute exacerbation of his habitual focal motor seizures. C:The ictal onset is punctuated by the appearance of evolving low- to high-amplitude paroxysmal fast activity arising from the left centroparietal region.
Small epileptogenic foci may be entirely missed with interictal (and even ictal) surface EEG recordings (see Fig. 11.8). On the other hand, interictal epileptiform abnormalities may have a misleadingly widespread appearance because of the large distance and intervening cortical area that separates the epileptogenic zone from the scalp EEG electrodes (125).
Random EEG tracings in patients with focal epilepsy may not show evidence of focal epileptiform activity. In a case series of 19 patients with refractory frontal lobe epilepsy, Salanova and associates reported absence of interictal sharp waves in 7 of 19 (37%) patients (126). Secondary bilaterally synchronous discharges may be seen in up to two-thirds of patients with frontal lobe epilepsy (127). EEG interpretation should take into account the possibility of “secondary bilateral synchrony,” a term introduced by Tükel and Jasper to describe the bilateral discharges seen in patients with parasagittal epileptogenic lesions (128).
Ictal recordings may show regional seizure patterns (Fig. 11.9C) or may have limited localizing or lateralizing value. As a rule, the patterns are more widespread and more difficult to lateralize compared with those seen with seizures of temporal lobe origin. Studies show that ictal EEG may be nonlocalizing in more than half of patients with frontal lobe epilepsy (126,129). False localization may occur with an erroneous temporal ictal pattern on surface EEG as a result of underlying frontolimbic connections (125).
Particular EEG patterns may sometimes be seen in relationship to the corresponding seizure type: generalized tonic seizures, for example, may be associated with ictal EEG activity, which consists of repetitive rhythmic spiking of variable frequency (8 to 25 Hz, or faster) and amplitude (130). More recently, investigators have been able to ascertain the presence of gamma rhythms in the frequency range of 50 to 100 Hz associated with generalized tonic seizures during scalp EEG recordings in patients with Lennox–Gastaut syndrome (131).
Invasive recordings using subdural electrodes in frontal lobe epilepsies may only show evidence for a focal onset in a relatively small number of patients; often, a more diffuse “regional” pattern may be seen (126,132). This, of course, depends on the nature, location, extent, and connectivity of the underlying epileptogenic substrate and its relationship to the recording electrodes. In a careful invasive study of patients presenting with circumscribed focal clonic seizures, investigators observed that these seizures were always associated with a localized polyspike-and-wave intracranial ictal EEG pattern involving the subdural electrodes overlying the PMA, while neighboring subdural electrodes not overlying the precentral gyrus showed various other ictal patterns (133). Such ictal patterns associated with focal clonic seizures may occasionally be discernible by scalp EEG recordings.
EPILEPSIA PARTIALIS CONTINUA
Status epilepticus can be broadly divided into status epilepticus with motor or without motor phenomena (134). Subcategories of motor status epilepticus include generalized and secondarily generalized status, as well as focal motor status epilepticus. The latter is characterized by repetitive typical somatomotor seizures with or without jacksonian march originating from the perirolandic region. This condition may occur at the onset or during the course of epilepsies manifesting with focal motor seizures. Consciousness is usually preserved, and cerebral function of the uninvolved cortex remains intact. A variety of motor phenomena may be observed in the context of focal motor status ranging from overt to more subtle motor manifestations, such as epileptic nystagmus, for example, which may be seen in patients with oculoclonic status epilepticus (135,136).
Epilepsia partialis continua of Kojevnikov (EPC or partial continuous epilepsy) constitutes one form of focal motor status epilepticus, characterized by localized unremitting myoclonus. The condition was first described by Kojevnikov in 1895 as a disorder of persistent localized motor seizures (137). In published literature, EPC has been referred to as “Kojevnikov,” “Kojewnikow,” or “Kozhevnikov” syndrome.
Clinical Semiology
EPC has been regarded by some as “the semiologic epitome of a focal seizure” (138), as it usually involves a restricted area of the motor cortex of one hemisphere and presents with clinically localized motor manifestations. EPC is defined by the occurrence of almost continuous and rhythmic or semirhythmic muscular contractions (myoclonic jerks) that remain localized to a limited area on one side of the body and persist for hours, days, or even years (139,140). The definition has undergone several revisions in the past, reflecting differences of opinion among various authors. In 1989, the ILAE Commission defined EPC as a specific form of continuous somatomotor seizures involving the Rolandic cortex (141). Any muscle group may be involved, but distal musculature is more commonly affected. The myoclonic jerks may appear isolated or in clusters, with a regular or irregular occurrence at a frequency of 1 to 2 per second. In general, unilateral involvement with synchronous activation of agonists and antagonists is observed. The jerks are predominantly seen involving the muscles of the upper half of the body. In a study of 151 patients presenting with EPC, the authors observed that during its course, the disease involved the head in 16% of patients, the head and upper limb in 14%, the upper limb only in 40%, the trunk in 5%, the lower extremity in 14%, and the whole side of the body in 11% (142).
By definition, the jerks are spontaneous, although they may be aggravated by physical activity, psychic exertion, and/or sensory stimuli (143). In most cases, the jerks may be reduced in amplitude but persist during sleep (144,145). Other seizure types (such as jacksonian or generalized seizures) and a variety of neurologic deficits may be seen in these patients depending on the underlying etiology.
The pathophysiology of EPC is not well understood. It has been postulated that the absence of seizure propagation is associated with the specific anatomical location of the epileptic focus within a neocortical area of sufficiently preserved inhibition. Virtually, all authors today agree that involvement of the PMA is indispensable for the generation and sustainment of EPC (146). Bancaud and coworkers divided EPC into two broad categories based on the presence (type 2) or absence (type 1) of a progressive brain lesion (139): Type 1 was associated with a regional nonprogressive lesion in the sensorimotor cortex, whereas type 2 was typically seen in the setting of Rasmussen syndrome.
EPC may develop at any age. The usual etiology of EPC is a focal lesion involving the cortex (principally the sensorimotor cortex) that results from stroke, trauma, infection, metastasis, or primary tumor. A hypoxic, metabolic, or septic encephalopathy may predispose patients with a preexisting focal lesion to develop EPC. All patients presenting with EPC should be carefully evaluated for an underlying lesion that may be amenable to curative resective surgery. In one series, EPC was caused by a variety of different pathologens, including inflammatory and immune-mediated (52%), metabolic (13.7%), structural brain abnormalities (11.8%), cryptogenic (7.8%), vascular (5.9%), dual (5.9%), and postoperative (2%) causes (147). EPC is, of course, a common manifestation of Rasmussen syndrome (seen in almost 50% of cases) (148). Despite its focal expression, EPC in Rasmussen syndrome may be associated with MRI and EEG evidence of more diffuse hemispheric abnormalities. In the UK series of 36 patients from ages 1 to 84 years, who presented with EPC over the period of 1 year, the commonest isolated etiology was Rasmussen syndrome in seven (19%; five were children), followed by stroke in five (14%). In seven patients, the cause of their presentation remained undetermined (140). EPC and its variants have also been reported in the setting of multiple sclerosis (149), human immunodeficiency virus infection (150,151), Creutzfeldt–Jakob disease (152), and other neurodegenerative diseases such as mitochondrial disorders (153,154) or Alpers syndrome (155).
Focal motor seizures or EPC, or both, may be the presenting feature of nonketotic hyperglycemia or may occur as a later complication, especially in the presence of an underlying focal cerebral lesion (156). Hyperglycemia, hyponatremia, mild hyperosmolarity, and lack of ketoacidosis were found to contribute to the development of EPC predominantly in areas of preexisting focal cerebral damage in 21 patients with evidence of nonketotic hyperglycemia (157). One should note, however, that EPC has also been reported in the setting of ketotic hyperglycemia (140,158). Depending on the etiology, EPC may be an early or late feature in the course of the underlying disease and may be seen either in isolation or in association with other seizure types (159). Similar to EPC, focal somatomotor status may reflect an underlying focal brain lesion (secondary to a vascular, neoplastic, traumatic, or infectious etiology) or may present in the context of toxic–metabolic abnormalities. In patients without preexisting epilepsy, the onset of focal motor status may signify underlying “asymptomatic” ischemia or interterritorial cerebral infarction (infarction in watershed territories).
Subcortical or spinal myoclonus and certain forms of tremor and other extrapyramidal movement disorders should be considered in the differential diagnosis of EPC. Clinical differentiation is often challenging, and special neurophysiologic examinations may be necessary (159,160).
EEG Findings
The conventional scalp EEG may be unrevealing or misleadingly normal. There may be very few or no paroxysmal abnormalities; and the background rhythm may be normal. In some cases, time-locked EEG events preceding the EPC-associated myoclonic jerks can be detected by back averaging. In addition, special stereoencephalographic or electrocorticographic recordings may prove helpful in resolving the underlying spike focus.
In other cases, irregular 0.5- to 3-Hz slowing may be seen in the frontocentral region along with reduction of the beta activity in the same area (45), but there are no characteristic EEG findings to aid in the diagnosis of EPC. In a study of 32 cases, the most common EEG finding was regional spiking (144). Other abnormalities included bursts of sharp waves or spike-and-wave discharges and unilateral or bilateral runs of abnormal rhythms. In a study of 21 adults presenting with EPC, the authors found EEGs to be abnormal in all but one patient. Each patient underwent at least two EEGs in the course of the disease; consequently more than one pattern was seen in some patients. The most common EEG finding was the presence of unilateral lateralized and/or localized spike or sharp wave discharges in ten patients (48%). Other lateralized abnormalities ranged from periodic lateralized epileptiform discharges (PLEDs) in three, paroxysmal slow-wave activity in two, and lateralized continuous slow activity in four patients. Four patients exhibited diffuse, continuous slow activity, one showed paroxysmal generalized slow-wave activity, and one patient had periodic generalized epileptiform discharges (161). In this study, only seven patients (33%) were found to have epileptiform discharges during EPC that correlated to the myoclonic jerks.
On rare occasions, PLEDs and PLED-like patterns that are time locked to the jerks are observed in the course of EPC (162). PLEDs are commonly viewed as a transient interictal pattern (see Fig. 11.9B), which usually disappears within a few days, but may last as long as 3 months or longer (163). PLEDs can occur in a variety of structural and metabolic disorders usually of acute or subacute nature (164,165). It is important to note that depending on the clinical scenario, there are occasions when PLEDs in fact represent an ictal EEG pattern (166). Furthermore, one should not overlook the common occurrence of seizures in association to the presence of PLEDs on EEG recordings—seizures have been reported to occur with a frequency ranging from 58% to 100% in some studies (164). The most common seizure type seen in the presence of PLEDs is focal motor seizures affecting the contralateral body (167), often presenting as status epilepticus or as repetitive focal motor seizures (164,167,168).
In Rasmussen encephalitis, it is important to identify and document MRI evidence of progressive atrophy, which usually involves only one hemisphere. An abnormal EEG with progressive regional or lateralized slowing and ipsilateral regional or multiregional spiking is the rule. In a case report of an 11-year-old girl with Rasmussen syndrome, and a 5-month history of EPC, fluorodeoxyglucose positron emission tomography (FDG-PET) studies demonstrated an area of hypermetabolism in the right central cortex and ipsilateral thalamus. A congruent sharp wave focus was present in the same region on scalp EEG recordings. Using simultaneously recorded EMG of the left tibialis anterior muscle, the authors demonstrated regular jerks, time locked with the right central sharp waves (169). With time, the EEG abnormalities of Rasmussen encephalitis may become bilateral or more widespread, multiregional, or synchronous suggesting progression of a more diffuse process than indicated by the clinical manifestation of EPC.
SUPPLEMENTARY SENSORIMOTOR SEIZURES
Clinical Semiology
Seizures arising from the SSMA are of brief duration, usually lasting only 10 to 40 seconds. Rapid onset of asymmetric tonic posturing involving one or more extremities is characteristically observed (52,170). While it is common for both sides of the body to be affected simultaneously, unilateral tonic motor activity may occur (171). The typical seizure is frequently referred to as a bilateral asymmetric tonic seizure.
Speech arrest and vocalization are common. Somatosensory symptoms, such as numbness, tingling, or pressure sensation, may precede the phase of tonic posturing (41); these body sensations are not well localized in contradistinction to somatosensory symptoms that result from epileptic activation of the postcentral gyrus (172). Common descriptions include a feeling of tension, pulling, or heaviness in an extremity or a sense that the extremity is “about to move” (173). In addition, the sensation of either an urge to perform a movement or an anticipation that some movement is about to occur has been reported in response to electrical stimulation (62). Although consciousness is usually preserved, patients may not be able to respond verbally during the tonic phase. Toward the end of the seizure, a few rhythmic clonic movements of the extremities may be observed (173). Postictal confusion is absent in the majority of SSMA seizures.
Asymmetric involvement of the upper extremities usually manifests with abduction at the shoulders, flexion of one elbow, and extension of the other upper extremity. As a rule, the lower extremities are also involved in the tonic posturing, with abduction at the hips and flexion or extension at the knees (52). Even patients, in whom tonic posturing appears to be unilateral, have bilaterally increased (or decreased) tone (34).
In their original report and illustrations of “somatic sensory seizures” arising from the SSMA, Penfield and Jasper (41) described head turning to the side of the flexed upper extremity. They observed that the head and eyes appear as if looking toward the flexed and raised arm with the patient adopting the so-called fencing posture—a motor response reminiscent of the asymmetric tonic neck reflex (34). Ajmone-Marsan and Ralston (174) subsequently coined the term “M2e” to describe the abduction and elevation of the contralateral arm with external rotation at the shoulder and slight flexion at the elbow. The patient’s head and eyes deviate as if looking at the raised arm, while both lower extremities remain extended or slightly flexed at the hips and knees.
In contrast to these early reports, further analysis of SSMA seizures showed that assumption of the classic “fencing” or “M2e” posture is not common (103,175). Among the less common motor manifestations, coarse movements of the tonic postured extremities may be observed (176). If present, vocalization may be prominent during the tonic phase reflecting the tonic involvement of the diaphragm and laryngeal muscles, which contract against semiclosed vocal cords (173).
Ictal activity may spread to involve the PMA of the face on the dorsolateral convexity, resulting in unilateral clonic movements or contralateral head version. Secondary generalization may lead to a generalized tonic–clonic seizure. Clonic movements can be seen toward the end of the tonic seizure (173). Unusual hyperkinetic automatisms (involving the ipsilateral upper extremity) have been described with seizures involving the SSMA (177). Finally, writhing movements may be seen as some patients attempt to move around or sit up during the tonic seizure.
SSMA seizures may be frequent (up to 5 to 10 per day) and can occur in clusters. They tend to occur predominantly during sleep (172,178). In a systematic review of their relationship with sleep, almost two-thirds of a total of 322 SSMA seizures in 24 patients occurred during sleep, almost exclusively during non–rapid eye movement (NREM) sleep stages I and II—as demonstrated by video–EEG recordings (179).
It should be emphasized that only a minority of patients with seizures displaying the clinical features of SSMA activation (“SSMA seizures”) actually have “SSMA epilepsy” (180). In most cases, the SSMA functions as the symptomatogenic zone: the observed seizures reflect the expression of ictal discharges originating from clinically silent regions that have anatomical or functional proximity to the SSMA, such as the basal frontal regions, the dorsolateral convexity of the frontal lobe, and the mesial parietal regions (52). This important point is illustrated by a recent stereo-EEG study of 14 patients with intractable focal epilepsy presenting with SSMA seizure semiology. Invasive EEG recordings showed evidence of seizure origin within the SSMA region in only six (43%) patients. The eight remaining patients were found to have diffuse unilateral or bilateral seizure onset. The authors concluded that SSMA semiology is suggestive of early involvement of this region, but is by no means a reliable indicator that the SSMA itself contains the seizure focus (181). Consequently, the SSMA itself may not need to be sacrificed in patients presenting with intractable SSMA seizures. Moreover, unless the location of the epileptogenic focus/generator has been carefully defined, resection of the SSMA may not be associated with a favorable postoperative outcome (182). Furthermore, the clinical picture of SSMA seizures with involvement of all four extremities and simultaneous preservation of awareness may be misleading, and it is not unusual for patients with this type of paroxysmal activity to be misdiagnosed as having psychogenic nonepileptic seizures (116). Of note, stereo-EEG (SEEG) studies now also suggest involvement of the SMA in startle seizures. Three patients with startle seizures became seizure free after epilepsy surgery in the SMA (183). In these patients resection was guided by SEEG suggesting prominent but not exclusive involvement of the SMA in startle seizures (183).
EEG Findings
Interictal sharp waves, when present, are usually found at the midline, maximum at the vertex, or just adjacent to the midline in the frontocentral region (Fig. 11.10A). Only 50% of 16 patients with supplementary sensorimotor seizures, who underwent evaluation with subdural electrodes, had shown scalp EEG evidence of midline frontocentral interictal epileptiform activity (184).
Figure 11.10. Interictal (A) and ictal (B and C) scalp electroencephalogram tracings with a transverse bipolar montage from a 12-year-old girl with left SSMA epilepsy. Bilateral asymmetric tonic seizures arising from sleep and wakefulness were captured during video–electroencephalograph evaluation. A:This awake recording shows sharp waves at the vertex (virtually confined to the Cz electrode). Note that the potential amplitude is slightly higher on the left (C3) than on the right (C4) central electrode. B:Clinical onset of typical seizure occurring out of sleep coincides with the appearance of bilateral myogenic artifact. Scalp EEG does not reveal a clear ictal pattern at the time of clinical onset. C: Rhythmic, repetitive sharp waves are present at the vertex within 15 seconds of clinical onset.
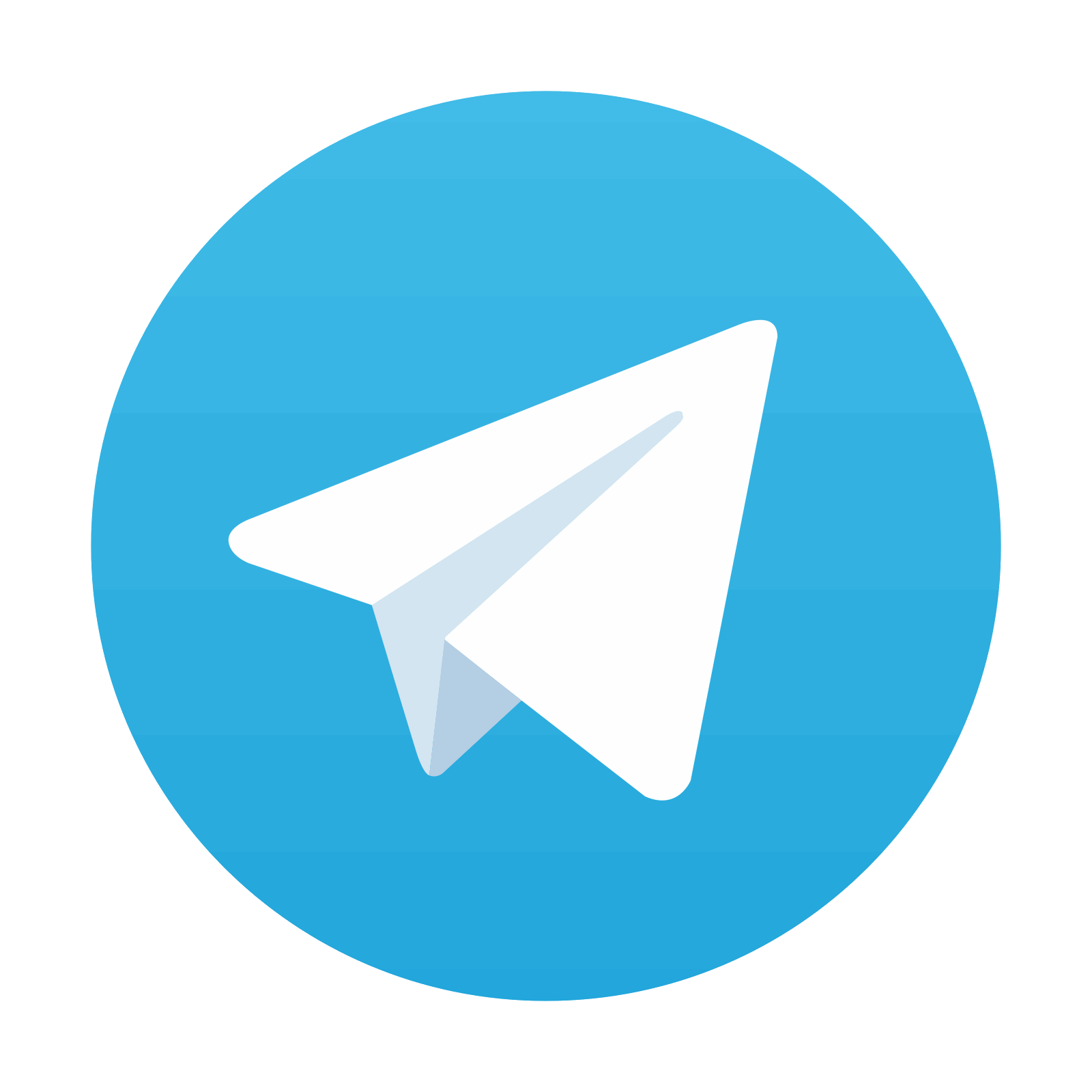
Stay updated, free articles. Join our Telegram channel

Full access? Get Clinical Tree
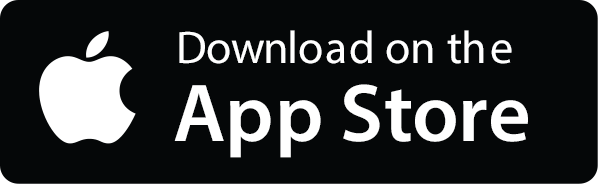
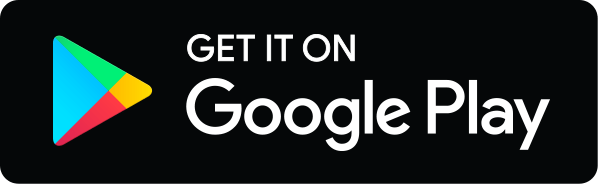