Focal Motor Seizures, Epilepsia Partialis Continua, and Supplementary Sensorimotor Seizures
Andreas V. Alexopoulos
Dudley S. Dinner
HISTORY
Focal motor seizures have been recognized since the time of Hippocrates, who first observed seizures affecting the body contralateral to the side of head injury (1). Hughlings Jackson was the first to hypothesize that focal seizures are caused by “a sudden and excessive discharge of gray matter in some part of the brain” and that the clinical manifestations of the seizure depend on the “seat of the discharging lesion” (2,3).
During the second half of the 19th century, Fritsch and Hitzig pioneered stimulation of the brain in animals (4). They discovered that electrical stimulation of the exposed cerebral cortex produced contralateral motor responses in dogs (5,6). Experimental faradic stimulation of the human cerebral cortex was first performed by Bartholow in 1874 (7). In 1909. Cushing reported that faradic stimulation of the postcentral gyrus could be used to determine the anatomic relationship of the sensory strip to an adjacent tumor (8). Motor responses elicited by electrical stimulation in humans were first described by Krause in the beginning of the 20th century (9) and by Foerster more than 60 years ago (3). These early observations led to the fundamental work of Penfield and Brodley, who used electrical stimulation to describe the motor and sensory representation of the human cerebral cortex and pioneered the techniques for the functional localization of the sensorimotor cortex during surgery (10).
FUNCTIONAL ANATOMY OF THE MOTOR CORTEX
Strictly speaking, the motor cortex (Fig. 17.1) consists of three motor areas: the primary motor cortex (PMA or M1) in the precentral gyrus, which houses a complete representation of body movements; the supplementary sensorimotor area (SSMA or SMA) on the mesial surface rostral to the PMA, also containing a complete motor representation (hence the term supplementary); and a more loosely defined premotor cortex (PMC) on the lateral cortical convexity (11).
The prefrontal and orbitofrontal cortex, as well as the dorsolateral and mesial frontal cortex anterior to the SSMA, are not considered part of the motor cortex. The term prefrontal cortex is used to define the extensive part of the frontal lobe that lies anterior to the motor and premotor zones (12). Recent studies in humans and primates challenge the traditional division of motor areas. For example, part of the cingulate motor cortex, which was previously linked to the limbic system, is now viewed as a potential fourth motor area. Moreover, the use of modern
anatomic and physiologic techniques has enabled researchers to explore and define additional subdivisions of motor areas in both humans and primates (13).
anatomic and physiologic techniques has enabled researchers to explore and define additional subdivisions of motor areas in both humans and primates (13).
Activation studies using positron emission tomography (PET) or functional magnetic resonance imaging (fMRI) allude to the complex organization of the motor system. The breadth of cortical and subcortical areas activated with even the simplest movements attests to the wide distribution and extent of interconnected neural networks underlying motor control (14). Observed movements presuppose a series of parallel or sequential processes involving the selection, planning, preparation, and initiation of action (14,15).
Efferent and Afferent Connections
The familiar hierarchical model of motor control is based on the four levels of spinal cord, brainstem, PMA, and premotor cortex-SSMA. This concept has influenced our understanding of the various motor manifestations of seizures (16). Motor commands are organized hierarchically from the most automatic (e.g., deep tendon reflexes) to the least (e.g., skilled and precise voluntary movements). Each level of motor control retains a somatotopic organization and receives peripheral sensory information that is used to modify the motor output at that level (17). The cerebral cortex exerts its motor control by way of the corticospinal and corticobulbar pathways. The cortex also modulates the action of motor neurons in the brainstem and spinal cord indirectly through its influence on the brain’s various descending systems.
To this day, limited direct information exists about specific neuronal connections between functional brain regions of the human cortex (18). Our knowledge of detailed connectivities derives from studies of nonhuman primates using invasive tracer techniques. Such invasive studies, however, cannot be performed in humans, and animal results cannot always be extrapolated to human systems (19,20).
Brainstem Motor Efferents
The brainstem gives rise to several descending motor pathways, which are divided into ventromedial and dorsolateral groups (21,22). The ventromedial system sends fibers through the ventral columns of the spinal cord and terminates predominantly in the medial part of the ventral horn, which contains the motor nuclei controlling proximal limb and axial muscle groups. In contrast, the dorsolateral system descends in the lateral part of the spinal cord and terminates on the lateral motor cell complex (23), which innervates more distal limb muscles.
The rubrospinal tract comprises the main dorsolateral pathway and has its origin in the midbrain (in the magnocellular portion of the red nucleus). The red nucleus receives input from the motor cortex and cerebellum and provides an indirect route for these areas to influence the spinal cord.
The ventromedial system consists of four major descending tracts. Two arise from the midbrain—tectospinal tract (from the superior colliculus) and interstitiospinal tract (from the interstitial nucleus of Cajal in the rostral mesencephalon)—while the other two have their origin in the medulla and pons (vestibulospinal and reticulospinal tracts). The pontine and medullary vestibulospinal pathways play a role in extensor muscle control and maintenance of posture (24). Finally, the reticulospinal pathways modulate postural reflexes and crude voluntary movements. Their excitatory connections with spinal motor neurons innervating axial and proximal limb muscles are largely ipsilateral (23) and are implicated in the production of tonic and atonic motor phenomena (16).
Motor Cortex Efferents
The axons that project from layer V of the cortex to the spinal cord run together in the corticospinal tract (a massive bundle of fibers containing approximately 1 million axons). About one third of corticospinal and corticobulbar fibers arise from the PMA. Another third originate from the SSMA and PMC, and the rest have their origin in the parietal
lobe (arising mainly from the somatosensory cortex of the postcentral gyrus) (17,22,25). The corticospinal fibers together with the corticobulbar fibers run through the posterior limb of the internal capsule to reach the ventral portion of the brainstem and send collaterals to the striatum, thalamus, red nucleus, and other brainstem nuclei (26). In the brainstem, the corticobulbar fibers terminate bilaterally in cranial nerve motor nuclei (either directly via a monosynaptic route or indirectly), with the exception of motor neurons innervating the lower face, which receive mostly contralateral corticobulbar input. About three-fourths of the corticospinal fibers cross the midline in the pyramidal decussation at the junction of the medulla and spinal cord and descend in the spinal cord as the lateral corticospinal tract (17). Uncrossed fibers descend as the ventral corticospinal tract. The lateral and ventral divisions of the corticospinal tract terminate in approximately the same regions of spinal gray matter along with corresponding brainstem-originating pathways. The majority of corticospinal tract terminals project on spinal interneurons. An estimated 5% of the fibers synapse directly on (both alpha and gamma) motor neurons (27).
lobe (arising mainly from the somatosensory cortex of the postcentral gyrus) (17,22,25). The corticospinal fibers together with the corticobulbar fibers run through the posterior limb of the internal capsule to reach the ventral portion of the brainstem and send collaterals to the striatum, thalamus, red nucleus, and other brainstem nuclei (26). In the brainstem, the corticobulbar fibers terminate bilaterally in cranial nerve motor nuclei (either directly via a monosynaptic route or indirectly), with the exception of motor neurons innervating the lower face, which receive mostly contralateral corticobulbar input. About three-fourths of the corticospinal fibers cross the midline in the pyramidal decussation at the junction of the medulla and spinal cord and descend in the spinal cord as the lateral corticospinal tract (17). Uncrossed fibers descend as the ventral corticospinal tract. The lateral and ventral divisions of the corticospinal tract terminate in approximately the same regions of spinal gray matter along with corresponding brainstem-originating pathways. The majority of corticospinal tract terminals project on spinal interneurons. An estimated 5% of the fibers synapse directly on (both alpha and gamma) motor neurons (27).
These anatomic arrangements of descending tracts underlie the contralateral and/or bilateral motor manifestations of focal seizures arising from the motor cortex (16).
Motor Cortex Afferents
The major cortical inputs to the motor areas of cortex are from the prefrontal and parietal association areas (17). These are focused mainly on the PMC and the SSMA, whereas the PMA receives a large input from the primary somatosensory cortex (28). In addition, the PMA receives direct and indirect input from the PMC and the SSMA. In particular, the SSMA projects bilaterally to the primary motor cortex in a somatotopically organized manner. Other corticocortical inputs arrive from the opposite hemisphere through the corpus callosum, which interconnects heterotopic as well as homologous areas in the two hemispheres (29). The major subcortical input to the motor cortical areas comes from the thalamus, where separate nuclei convey modulating inputs from the basal ganglia and cerebellum (17,28).
Stimulation Studies
In clinical practice, insights into the functional anatomy of the motor cortex and other eloquent brain cortical areas are afforded by direct cortical stimulation. The development of subdural electrodes, which are implanted long term and allow recordings over several days or weeks in patients with epilepsy, has provided the opportunity to perform electrical stimulation studies of the cortex, including the PMA and SSMA, before surgery. At the same time, electrical stimulation of the human cortex provides an experimental model that can be used to reproduce the effects of cortical activation after an ictal discharge (30). In fact, several groups have used cortical stimulation to trigger habitual auras and/or seizures, in an attempt to better delineate the ictal onset zone before epilepsy surgery.
In general, the observed clinical response is assumed to arise from cortex below the stimulated electrode or from the region between two closely spaced electrodes, given that the current density drops off rapidly with increasing distance from the tissue underlying the stimulated electrode (31,32). Electrical stimulation can elicit “positive” responses (such as localized movements resulting from activation of the PMA or SSMA cortex) or “negative” responses (such as inhibition of motor activity). The latter becomes apparent only if the patient engages in specific tasks during stimulation.
In areas such as the supplementary motor cortex, both positive responses in the form of bilateral motor movements and negative responses such as speech arrest can be demonstrated. The area of stimulation gives rise to distinctive patterns of motor activation of the PMA, SSMA, or premotor regions. In addition, however, overlapping clinical manifestations may be observed as a result of the highly developed interconnectivity between these regions (33).
Negative motor responses interfere with a person’s ability to perform a voluntary movement or sustain a voluntary contraction when cortical stimulation is applied (34). The patient is unaware of the effects of stimulation unless asked to perform the specific function integrated by the stimulated cortical region. In a systematic review of 42 patients who had subdural electrodes implanted over the perirolandic area, the Cleveland Clinic group observed negative motor responses when stimulating the agranular cortex immediately in front of the primary and supplementary face areas. Negative motor responses were seen over both hemispheres (34). To distinguish the two negative motor areas, investigators proposed the terms primary negative motor area (PNMA, in regard to the region of the inferior frontal gyrus immediately in front of the face PMA) and supplementary negative motor area (SNMA, in reference to the mesial portion of the superior frontal gyrus immediately in front of the face SSMA).
The effects of electrical stimulation on the different components of the motor cortex are discussed below.
Primary Motor Area
The PMA resides in the anterior wall of the central sulcus (Fig. 17.1) and corresponds to Brodmann’s area 4. On the basis of cytoarchitectonic criteria, area 4 is recognized primarily by the presence of Betz cells (giant pyramidal cells) in cortical layer V and the absence of a granular layer IV (35). The central sulcus marks the border between the agranular motor cortex and the granular somatosensory cortex (36).
The somatotopic organization of the PMA was elucidated by the pioneering work of Krause (9), Penfield and Jasper, and others (37,38) (Fig. 17.2). In this region of the
PMA, simple movements were elicited with the lowest intensity of electrical stimulation (39). The resulting motor maps show an orderly arrangement with the tongue and lips near the sylvian fissure and the thumb, digits, arm, and trunk represented successively along the central sulcus, ending with the leg, foot, and toes on the mesial surface. The layout of the motor homunculus (Fig. 17.2) is topographically similar to that of the somatosensory homunculus, which resides immediately behind the PMA. The somatotopic organization of the motor cortex is not fixed and can be altered during motor learning or after injury. Output of the PMA is directed to the corticospinal and corticobulbar tracts, as well as to the SSMA and homologous areas in the opposite hemisphere via the corpus callosum (40).
PMA, simple movements were elicited with the lowest intensity of electrical stimulation (39). The resulting motor maps show an orderly arrangement with the tongue and lips near the sylvian fissure and the thumb, digits, arm, and trunk represented successively along the central sulcus, ending with the leg, foot, and toes on the mesial surface. The layout of the motor homunculus (Fig. 17.2) is topographically similar to that of the somatosensory homunculus, which resides immediately behind the PMA. The somatotopic organization of the motor cortex is not fixed and can be altered during motor learning or after injury. Output of the PMA is directed to the corticospinal and corticobulbar tracts, as well as to the SSMA and homologous areas in the opposite hemisphere via the corpus callosum (40).
![]() Figure 17.2 The motor homunculus after Penfield and Rasmussen (37), depicting the somatotopic arrangement of the primary motor cortex (PMA) (with the tongue and lips near the sylvian fissure and the thumb, fingers, arm, and trunk represented successively along the precentral gyrus ending with the leg, foot, and toes on the mesial surface). Muscle groups involved in fine movements feature a disproportionately large representation. (Adapted from Penfield W, Rasmussen T. The cerebral cortex of man—a clinical study of localization of function. New York: Macmillan, 1950 p. 57) |
Stimulation Studies
In the PMA, single stimuli typically elicit single clonic movements of the contralateral somatic muscles represented by the area of the motor homunculus being stimulated. High-frequency (50 to 60 Hz) stimulus series result in slower, tonic contralateral motor responses (41). Intraoperative application of electrical stimulation mapping provides the most direct and easy way to localize the somatosensory cortex (42). In most adults, motor responses can be evoked from the precentral rolandic cortex under local or general anesthesia. When local anesthesia is used, these effects are usually observed with currents of 2 to 4 mA. Sensory responses are elicited with stimulation of the postcentral gyrus, often at slightly lower thresholds (43). The threshold for eliciting a motor response in humans is lowest in the PMA.
Supplementary Sensorimotor Area
The SSMA is a distinct anatomic region located on the mesial surface of the superior frontal gyrus and its adjacent dorsal convexity (44). The cerebral cortex of the SSMA corresponds to the mesial portion of area 6 of Brodmann’s cytoarchitectonic map of the brain (Fig. 17.1) (38,45). Similar to the PMA cortex, the SSMA is referred to as agranular cortex, because the internal granular layer (layer IV) is not prominent. In contrast to area 4, area 6 does not contain Betz cells (45). The medial precentral sulcus defines the border between the primary motor area for the foot and the posterior limit of the SSMA (13,46). No clear cytoarchitectonic or anatomic boundary separates the SSMA from the adjacent PMC (47).
The macaque and human mesial area 6 (SSMA) is further subdivided into pre-SSMA (rostrally) and SSMA proper (caudally) on the basis of comparable cytoarchitectonic and transmitter receptor studies (36). Studies in primates suggest that the pre-SSMA holds a hierarchically higher role in motor control. However, the functional properties of the SSMA subdivisions have not been detailed in humans (48). The border between the pre-SSMA and SSMA proper corresponds to the VCA line (the vertical line passing through the anterior commissure and perpendicular to the AC-PC line, which connects the anterior and posterior commissures). The border between SSMA proper and PMA corresponds approximately to the VCP line (the vertical line that traverses the posterior commissure and is perpendicular to the AC-PC line) (49).
Stimulation Studies
More than 60 years ago, Foerster was the first to describe motor responses in humans elicited by electrical stimulation of the mesial aspect of the superior frontal gyrus anterior to the primary motor representation for the lower extremity (3). Systematic study of this region with electrical stimulation was carried out at the Montreal Neurological Institute during the intraoperative evaluation of patients with intractable focal epilepsy preceding surgical resection (50,51).
This was the first group to use the term supplementary motor area. Direct electrical stimulation of the SMA produced vocalization, speech arrest, postural movements of all extremities, inhibition of voluntary movements, and autonomic
changes. The Montreal studies demonstrated that both positive (such as bilateral motor movements) and negative responses (such as speech arrest) could be elicited by stimulating this region. Intraoperative studies of the SMA may be limited because of the restricted amount of time and relative difficulty of accessing the mesial aspect of the hemisphere and recognizing the specific gyral land-marks during surgery.
changes. The Montreal studies demonstrated that both positive (such as bilateral motor movements) and negative responses (such as speech arrest) could be elicited by stimulating this region. Intraoperative studies of the SMA may be limited because of the restricted amount of time and relative difficulty of accessing the mesial aspect of the hemisphere and recognizing the specific gyral land-marks during surgery.
With the advent of subdural electrodes, the Cleveland Clinic series of extraoperative stimulation studies showed that positive motor responses were not restricted to the mesial aspect of the superior frontal gyrus, but could also be elicited from its dorsal convexity, the lower half of the paracentral lobule, and the precuneus (52). The same group confirmed the presence of sensory symptoms that were elicited along with the positive motor responses after stimulation of the SMA and coined the term supplementary sensorimotor area instead of SMA.
Using depth electrodes, Talairach and Bancaud were the first to describe a somatotopic organization within the SSMA (53). The Yale group confirmed the presence of somatotopic distribution in the SSMA, where the face, upper extremity, and lower extremity responses are oriented in a rostrocaudal direction, with the lower extremities represented posteriorly, head and face most anteriorly, and the upper extremities between these two regions (54). Likewise, studies of movement-related potentials (MRPs) using subdural electrodes implanted over the SSMA region demonstrated that the MRPs for different types of movements (of the finger, foot, and tongue as well as vocalization) also have a somatotopic distribution within the SSMA, which is consistent with the SSMA organization defined by electrical stimulation (55, 56, 57).
Premotor Cortex
Fulton coined the term premotor cortex in 1935 (58) to describe the third major component of the motor cortex. This area encompasses the more loosely defined agranular cortex of the lateral frontal convexity rostral to the PMA (11,22), which corresponds to the lateral portion of Brodmann’s area 6 (Fig. 17.1). It is very difficult to define the anterior border of the agranular PMC in humans, where a broad zone of progressive transition exists between area 6 and the granular cortex of Brodmann’s frontal area 9 (59). In the macaque, the PMC is further subdivided into a dorsal portion on the dorsolateral convexity and a ventral portion on the ventrolateral convexity (11). Despite the lack of direct correlation between microstructure and function in humans, the two subdvisions of the premotor area are considered to have homologous counterparts in the human brain.
The motor and premotor cortices, as well as the frontal eye fields and the anterior cingulate cortex of area 24, have reciprocal connections with the SSMA (45). Anatomic labeling experiments in the macaque have demonstrated that the more anterior dorsal premotor cortex projects to the spinal cord, challenging the notion that the PMC, unlike the PMA and SSMA, lacks prominent corticospinal connections (22,60,61).
According to the classic schema, the PMC is responsible for the preparation and organization of movements (47). Several recent studies show that the PMC also plays a central role in nonmotor attentional and receptive domains. Therefore, our current understanding suggests a dual PMC function pertaining to motor and cognitive behavior (62).
Stimulation Studies
On the basis of early electrical stimulation studies of the monkey brain (63) the agranular lateral PMC (area 6) has been subdivided into a rostral (6aβ or 6r) and a caudal section (6aα or 6c). Recent quantitative architectonic and neurotransmitter studies have corroborated the presence of similar topographic boundaries in the human brain (36,59). The rostral subdivision covers the anterior part of the precentral gyrus, and its caudal counterpart resides in the posterior part of the superior and middle frontal gyri, in front of the precentral sulcus (64).
Eye movements can be electrically induced from a large area of the human dorsolateral frontal cortex and the precentral gyrus. These stimulation-elicited responses have been attributed to electrical interference with the human homologue of the monkey frontal eye field (65). Electrical cortical stimulation studies in epileptic patients undergoing presurgical evaluation have confirmed the functional location of the eye movement sites anterior to the motor representation of arm and face (65,66). However, some ambiguity exists as regards the exact location of the human frontal eye field within this rather extensive oculomotor region. The divergence is largely caused by the methodologic differences of neuroimaging and electrical cortical stimulation studies.
The electrically defined human frontal eye field is located in the posterior end of the middle frontal gyrus (Fig. 17.1) immediately anterior to the precentral sulcus (and in proximity to the superior frontal sulcus). Electrical cortical stimulation of this area produces constant oculomotor responses characterized by low stimulation thresholds (65).
Conversely, neuroimaging studies of cerebral blood flow (CBF) changes suggest that the homologous region for the human frontal eye field lies posterior to the electrically defined frontal eye field. Indeed, the CBF-defined frontal eye field is located between the central and precentral sulci in front of the primary hand representation, suggesting that the eye movement field lies in Brodmann area 6 (in the region of the PMC homologous to the ventral PMC) (67,68).
FOCAL MOTOR SEIZURES
Focal seizure is the term proposed by the Task Force of the International League Against Epilepsy (ILAE) to describe
seizures in which the initial activation involves a limited number of neurons in part of one hemisphere (69). The terms localization-related or partial seizures have been used to describe the same seizure type. However, the more recently proposed diagnostic scheme of the ILAE Task Force prefers the less ambiguous term focal to partial or localization-related seizures (70).
seizures in which the initial activation involves a limited number of neurons in part of one hemisphere (69). The terms localization-related or partial seizures have been used to describe the same seizure type. However, the more recently proposed diagnostic scheme of the ILAE Task Force prefers the less ambiguous term focal to partial or localization-related seizures (70).
Motor features constitute the main clinical manifestations of motor seizures. As a rule, consciousness is retained in the majority of seizures arising from discrete motor regions. It is possible, however, for an ictal discharge to remain localized and still produce alteration of consciousness. Furthermore, certain motor manifestations and a patient’s anxious reaction to the seizure symptoms may prevent the patient from responding appropriately during seizures. It may therefore be difficult to ascertain the level of consciousness in several patients with focal motor seizures. In the past, the presence or absence of altered awareness was used to dichotomize seizures of focal onset into “simple partial” and “complex partial.” It is now proposed to move away from this dichotomy, which seems to “have lost its meaningful precision” (70).
The established International Classification of Epileptic Seizures (69) divides focal motor seizures into those with or without a march, versive, postural, and phonatory seizures. The diagnostic scheme proposed in 2001 is based on the use of a system of five axes (levels) intended to provide a standardized description of individual patients (70). Axis 2 now defines the epileptic seizure type or types experienced by the patient. Hence, focal motor seizures may present with elementary clonic motor signs, with asymmetric tonic motor seizures (a term commonly used to describe seizures arising from the SSMA), typical automatisms (a term that refers to seizures arising from the temporal lobe), with hyperkinetic automatisms, with focal negative myoclonus, and, finally, with inhibitory motor seizures. The addition of axis 1 allows for the systematic description of ictal semiology observed during seizures utilizing a standardized glossary of descriptive terminology (71). Ictal motor phenomena may be subdivided into elementary motor manifestations (such as tonic, clonic, dystonic, versive) and automatisms. Automatisms consist of a more or less coordinated, repetitive motor activity (such as oroalimentary, manual or pedal, vocal or verbal, hyperkinetic or hypokinetic) (71). Somatotopic modifiers may be added to describe the body part producing motor activity during seizures.
Another recent seizure classification is based on clinical symptomatology and is independent of electroencephalogram (EEG), neuroimaging, and historical information (72). This classification uses terms such as focal clonic, focal tonic, or versive, and evolution during the seizure is indicated by arrows. For example: left hand somatosensory aura → left arm clonic seizure → left versive seizure.
Clinical Semiology
This section reviews the elementary motor phenomena resulting from a variety of focal motor seizures. These seizures typically present with clonic or tonic manifestations. Hyperkinetic manifestations are usually attributed to seizures arising from (or spreading to) the frontal lobe. Other complex motor automatisms seen with focal seizures (such as oroalimentary, mimetic, or gestural automatisms) are reviewed elsewhere.
In a population-based study conducted in Denmark of 1054 patients with epilepsy who were between the ages of 16 and 66 years, 18% had “simple partial” seizures (73). In a large series of patients admitted to a single hospital over a period of 10 years, Mauguiere and Courjon (74) reviewed the types of focal seizures defined in 8938 patients with focal and/or generalized seizures. They found that 1158 patients (12.9%) had focal tonic or clonic seizures without march; 582 (6.5%) presented with hemitonic or clonic seizures; 461 (5.2%) had adversive seizures; and only 199 (2.2%) had jacksonian seizures.
Perirolandic epileptogenic lesions often involve both the precentral and postcentral gyri, giving rise to both motor and sensory phenomena (in one study of 87 “simple partial” seizures in 14 patients studied by video-electroencephalography, sensory phenomena were observed in approximately one-third of patients with focal motor seizures [75]). In a study examining the relationship between early ictal motor manifestations and interictal abnormalities of cerebral glucose consumption, Shlaug and colleagues (76) analyzed 48 consecutive patients with neocortical seizures. Patients with unilateral clonic seizures primarily had a hypometabolic defect in the contralateral perirolandic region. Patients with predominantly focal tonic manifestations showed hypometabolism within the frontomesial and perirolandic regions, whereas patients with versive seizures showed contralateral hypometabolism without a consistent regional pattern. Finally, patients with hypermotor seizures had areas of depressed metabolism involving the frontomesial, perirolandic, anterior cingulate, and anterior insular regions.
Postictally, patients may experience a transient functional deficit, such as localized paresis (Todd paralysis), which may last for minutes or hours (up to 48 hours or longer). This interesting clinical phenomenon of “postepileptic paresis” is the signature of a focal seizure and bears the name of Dr. Bentley Todd, who first described it in the mid-19th century (77). Todd paralysis is believed to result from persistent focal dysfunction of the involved epileptogenic region. Postictal Todd paralysis is a clinical sign of substantial value in lateralizing the hemisphere of seizure onset (78).
Clonic Seizures
Clonic seizures consist of repeated, short contractions of various muscle groups characterized by rhythmic jerking or twitching movements (79). These movements recur at
regular intervals of less than 1 to 2 seconds. Most clonic seizures are brief and last for less than 1 or 2 minutes. During this period, clonic movements may remain restricted to one region or spread in a jacksonian manner. The majority of focal motor seizures tend to involve the hand and face, although any body part may potentially be affected (80). Such predilection is attributed to the large cortical representation of the hand and face areas.
regular intervals of less than 1 to 2 seconds. Most clonic seizures are brief and last for less than 1 or 2 minutes. During this period, clonic movements may remain restricted to one region or spread in a jacksonian manner. The majority of focal motor seizures tend to involve the hand and face, although any body part may potentially be affected (80). Such predilection is attributed to the large cortical representation of the hand and face areas.
In accordance with the somatotopic arrangement within the precentral sulcus, clonic twitching of a corresponding group of muscles is the typical ictal manifestation of a localized discharge within the contralateral precentral gyrus. Penfield and Jasper (38) also noted that the angle of the mouth was involved first in seizures affecting the face; seizures of the upper extremity began in the thumb and index finger, whereas seizures of the lower extremity often began in the great toe.
The clonic movements are limited initially to the corresponding area of the body but may spread during the attack. Such a spread (e.g., from the muscles of the face to the ipsilateral hand or arm) is known as the “jacksonian march.” In these “jacksonian attacks,” the symptoms travel slowly from one territory to another, following the order of the corresponding somatotopic representation. The march usually starts from a distal body region (such as the thumb, fingers, great toe, or eyelids) and spreads toward a more proximal part. At times, the march may skip some areas, a phenomenon that may be related to different seizure thresholds in the symptomatogenic region. Holowach and associates (81) reported that 25 of 60 jacksonian seizures in children began in the face (8 in the periocular and 5 in the perioral region), 17 in the hand, 7 in the arm, 2 in the shoulder, and 9 in the leg and foot.
The term jacksonian epilepsy was proposed by Charcot in 1887 to describe seizures that begin and spread with a similar march of symptoms (82). Its continued use today serves to remind us of Hughlings Jackson’s astute clinical observations, which provided the basis for his revolutionary principles of functional localization (83). In his own words:
The part of the body where the convulsion begins indicates the part of the brain where the discharge begins and where the discharging lesion is situated. But from the focus discharging primarily the discharge spreads laterally to the adjacent “healthy” foci. One focus after the other is seized by the radiating waves of impulses. The march of the attack, the order in which the different parts of the body become involved, reveals the arrangement of the corresponding foci in the precentral convolution (3).
The term hemiconvulsions refers to unilateral clonic seizures (i.e., clonic activity affecting one side of the body). Prolonged unilateral convulsions followed by the onset of hemiparesis are described in the childhood syndrome of hemiconvulsion-hemiplegia-epilepsy (HHE), discussed briefly in the section on selected epilepsy syndromes at the end of this chapter.
A variety of myoclonic phenomena need to be differentiated from focal clonic seizures. Typically, myoclonic jerks are arrhythmic compared with clonic motor activity. Notable exceptions of more rhythmic motoric manifestations, which are usually discussed under the broad definition of myoclonus, include the terms epilepsia partialis continua (see below), segmental myoclonus, and palatal myoclonus (also called palatal tremor) (84). Many types of myoclonic phenomena (e.g., myoclonus caused by spinal cord disease or essential myoclonus) do not have an epileptic origin. In contrast, epileptic myoclonus is typically accompanied by an EEG correlate of spike or multispike-wave complexes (85,86). Therefore, the term myoclonic seizure is reserved for seizures whose main components are single or repeated epileptic myoclonias (87). Focal motor seizures are generally not considered to be myoclonic, although sometimes an accurate distinction may prove difficult (86). In fact, focal cortical myoclonus has been described in four children who had dysplastic lesions of the motor cortex and coexistent focal motor seizures (88). Finally, the paradoxic term negative myoclonus is reserved for cases of sudden, brief relaxations in tonic muscle contraction (84). Epileptic negative myoclonus can be seen in relationship with a number of heterogeneous epilepsy syndromes (89,90).
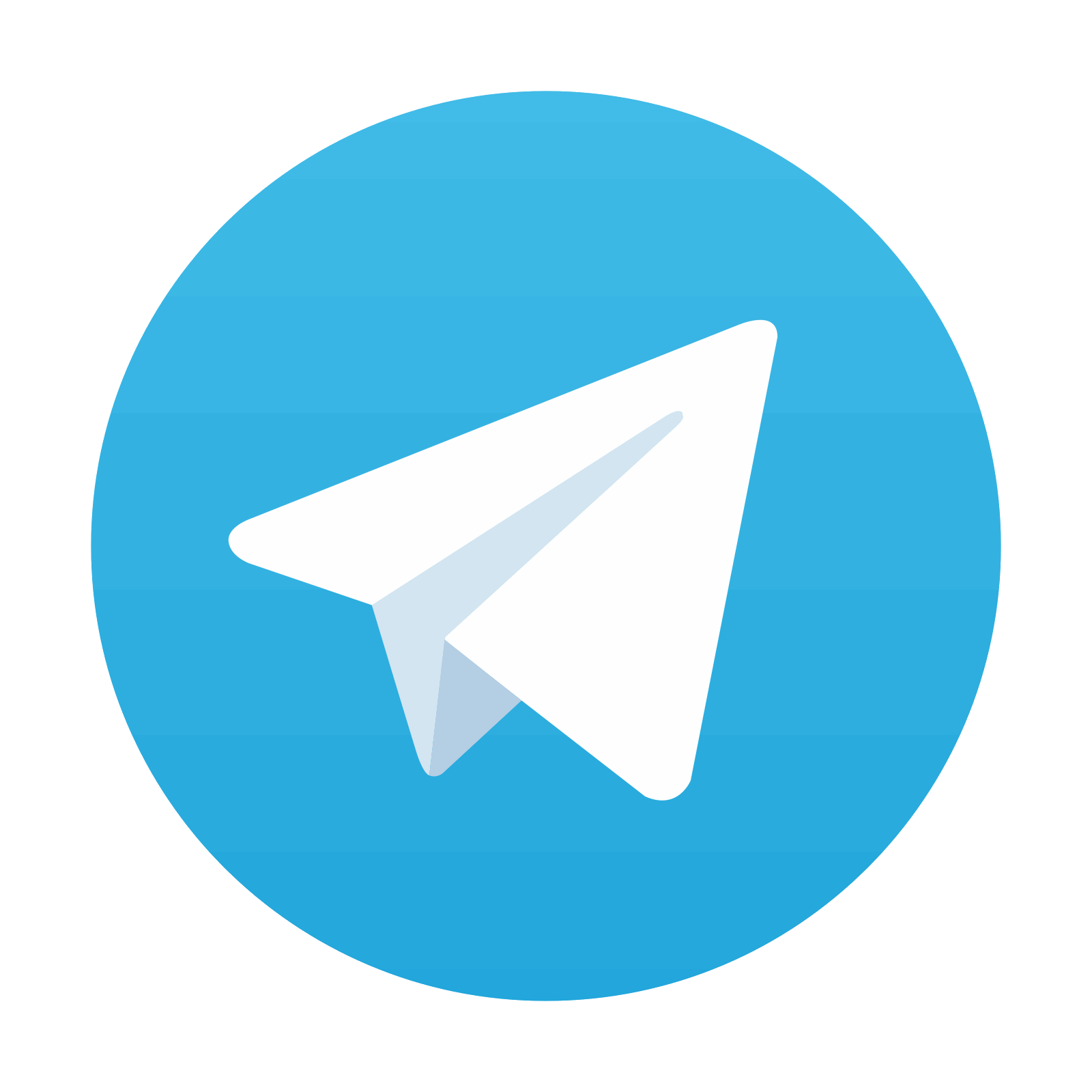
Stay updated, free articles. Join our Telegram channel

Full access? Get Clinical Tree
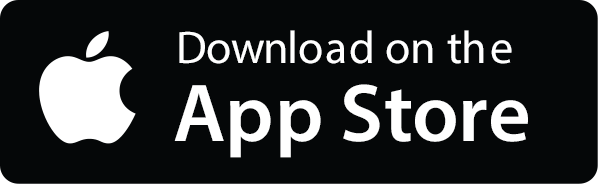
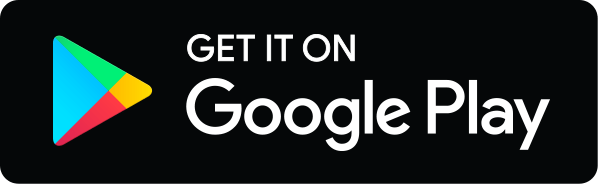