Fundamentals of Neuroradiology
Robert E. Morales
Dishant G. Shah
key points
Neuroradiologic images must always be evaluated in a systematic fashion with an understanding of fundamental neuroimaging concepts. Otherwise, not only can certain findings be overlooked, but one may quickly proceed down the wrong diagnostic path.
Only after these basic concepts are understood, should one focus on specific imaging features of particular disease processes.
Once a lesion is identified, it should be initially characterized by its location and by the type of edema that may be present. Only after these features are analyzed, should the CT density characteristics, MR signal characteristics, and possible contrast enhancement patterns be considered.
Neuroradiology has come a long way from the days when intracranial lesions were indirectly imaged. Air was placed into ventricles and contrast was injected into vessels to look for mass effect on these structures that would then suggest adjacent intracranial pathology. Fortunately, however, times have changed. We now have advanced tools to image lesions directly and less invasively. Magnetic resonance imaging (MRI) and computed tomography (CT) have become powerful instruments that not only depict these lesions but also can further characterize them. Often an abbreviated differential diagnosis can be suggested.
This chapter will review some of the fundamental principles of neuroimaging. Initially, the individual modalities of CT and MRI as well as the utility of contrast administration will be discussed. Basic topics will then be reviewed that form the foundation of image interpretation. These include the imaging findings of herniation and mass effect, hydrocephalus and volume loss, cytotoxic and vasogenic edema, and intraaxial and extra-axial masses. A brief discussion of spinal imaging will follow. Finally, due to their relatively common occurrence and particularly critical clinical implications, intracranial hemorrhage and cerebral ischemia/infarction will be specifically addressed.
COMPUTED TOMOGRAPHY VERSUS MAGNETIC RESONANCE IMAGING
The physics of CT and MRI is complex and beyond the scope of this chapter. In its most basic form, CT uses ionizing radiation to generate cross-sectional images from x-ray absorption of the tissues examined. MR images are generated using principles of in vivo magnetism and capturing signal from water and organic molecules that produce a magnetic field.
When discussing CT, structures are characterized with regard to their density or attenuation (of the x-ray beam), just as in the interpretation of plain x-ray films. Metal, bone, and calcification are densest (brightest), acute blood is denser than soft tissue, water is denser than fat, and air is the least dense (darkest). The density is measured in Hounsfield units (HU) with water having a HU of zero. Higher HU are measured in bone, blood, and soft tissue, whereas fat and air have HU in the negative range. This is an important fact to remember as HU measurement and density characteristics are often reported in radiology reports while characterizing findings on CT.
In MRI, structures are characterized with regard to their signal or intensity. There are now more than 20 sequences used in MRI for tissue characterization. In general, the basic sequences are T1 and T2. T1-weighted images are superior in depicting anatomic detail. Postcontrast images are nearly always T1-weighted images. Bright T1 signal is seen in fat, subacute hemorrhage (intracellular and extracellular methemoglobin), proteinaceous fluid, melanin, occasionally calcification and with the use of contrast (gadolinium). Bright T2 signal is seen in substances or tissue with relative higher watercontent such as cerebrospinal fluid (CSF), edematous/inflamed tissue, gliotic/encephalomalacic tissue, certain stages of hemorrhage (oxyhemoglobin and extracellular methemoglobin), and occasionally fat (if a fast technique of obtaining the T2-weighted images is utilized). FLAIR (fluid attenuated inversion recovery) sequence in basic terms, nullifies the bright CSF signal (free water) on the T2-weighted images, hence turning the CSF into a dark signal. Thus, pathology seen on T2-weighted images may be more conspicuous on this sequence. This is particularly evident in the periventricular white matter and at the cortex where, on T2-weighted images, the lesions may be somewhat obscured by the bright signal in the ventricles and sulci, respectively (Fig. 4.1).
▪ SPECIAL CLINICAL POINT: A useful approach when interpreting an MRI study is to initially utilize the T1-weighted images to evaluate the anatomy and underlying structure of the brain including the ventricles and CSF spaces. Since many pathologic processes result in surrounding edema, which is very high in signal and more conspicuous on the T2-weighted images, this sequence is then utilized to evaluate for underlying subtle pathology. If a lesion is detected, the signal characteristics on the T1-and T2-weighted images, as well as the possible presence and pattern of contrast enhancement, are then evaluated to more specifically characterize the lesion.
The T1- and T2-weighted pulse sequences are essentially the foundation of MRI. Other sequences such as FLAIR and diffusion-weighted imaging have been developed to increase the sensitivity of detecting lesions and to add more information when characterizing them.
Diffusion-weighted imaging has revolutionized the ability to image acute infarction. This sequence detects the change in Brownian motion of water molecules in injured tissue. Restricted diffusion signifies cell injury and appears as bright signal on the diffusion-weighted images (with corresponding dark signal on apparent diffusion coefficient [ADC] map images). This sequence generally takes less than a minute to obtain and is highly sensitive in acute infarction. For all practical purposes, diffusion restriction can be seen essentially at the time of onset of an acute infarct. Although diffusion-weighted imaging is usually utilized to evaluate for acute infarction, other lesions/substances, including highly
cellular tumors and purulent material can also restrict diffusion. These characteristics can be particularly helpful when evaluating for possible underlying intracranial abscess or purulent collection.
cellular tumors and purulent material can also restrict diffusion. These characteristics can be particularly helpful when evaluating for possible underlying intracranial abscess or purulent collection.
MRI has superior contrast resolution compared to CT, and in all but a few instances, it is superior to CT in evaluating the soft tissues of the central nervous system (CNS). CT is, however, much better in detecting and characterizing
osseous abnormalities. CT is superior to MRI in evaluating cortical bone and is used commonly in evaluating trauma patients for underlying fractures. In contrast, MRI is more sensitive in evaluating the marrow spaces for underlying edema or other infiltrating processes. As a result, it is useful in the imaging of infection and neoplasms. For all practical purposes, CT has better spatial resolution than MR and can be used in evaluating structures as small as 0.625 mm with current multislice detector scanners.
osseous abnormalities. CT is superior to MRI in evaluating cortical bone and is used commonly in evaluating trauma patients for underlying fractures. In contrast, MRI is more sensitive in evaluating the marrow spaces for underlying edema or other infiltrating processes. As a result, it is useful in the imaging of infection and neoplasms. For all practical purposes, CT has better spatial resolution than MR and can be used in evaluating structures as small as 0.625 mm with current multislice detector scanners.
▪ SPECIAL CLINICAL POINT: Although MRI is superior to CT at imaging nearly all the nonosseous components of the central nervous system, CT remains the imaging study of choice in the evaluation of acute intracranial hemorrhage, particularly acute subarachnoid hemorrhage. MRI, however, is probably more sensitive if the onset of symptoms was a few days prior.
Given the increased availability of CT scanners, faster turnaround time in obtaining the study, and cheaper cost, CT remains the mainstay of emergent imaging.
In a few instances, MRI is the initial study of choice. Since MRI is much better in evaluating the thecal sac and spinal cord, emergent MRI of the spine is indicated for the work-up of possible cord compression or intrinsic cord lesion as well as in the evaluation for spinal infection. In some institutions, MRI with limited sequences, including diffusion-weighted imaging, is performed emergently as part of the stroke center “brain attack” protocol.
Although both CT and MRI have tremendous use in the work-up of neurologic disorders, one must be aware of the potential disadvantages and contraindications when ordering a study. CT uses ionizing radiation, and cumulative doses over time may increase the risk of developing cancer. This risk is higher in the pediatric population due to growing cell lines. Every institution has specific imaging protocols, which use lower radiation in the pediatric population; however, this risk must be kept in mind while ordering any study with ionizing radiation. Likewise, there are also guidelines when performing CT and MRI in pregnant patients.
MRI is also usually contraindicated in patients with implanted electronic devices such as cardiac pacemakers, defibrillators, cochlear implants, and nerve stimulators. It is also contraindicated if the patient has ferromagnetic clips or foreign bodies that lay on or in close proximity to delicate structures such as the globes, brain, spinal cord, and nerves. There are resources, including websites, that can be used as a source of reference to determine if a specific device is MR compatible. All patients need to be screened prior to MRI to ensure safety.
Current scanner and postprocessing technologies have enabled us to further advance our abilities to detect CNS pathology. CT angiography (CTA) and MR angiography (MRA) have become essential tools in the imaging evaluation of neurovascular disorders such as in the work-up for infarcts, vascular injury, and vascular lesions, such as arteriovenous malformations (AVM) and aneurysms. Conventional angiography continues to remain the gold standard for neurovascular evaluation if noninvasive techniques do not provide adequate information. CT and MR perfusion imaging are being used more in the setting of acute stroke to evaluate for salvageable tissue. MR perfusion data can also be helpful in distinguishing recurrent neoplasm from radiation necrosis in neuro-oncology. Advanced MR techniques such as MR spectroscopy, functional MRI, and diffusion tensor imaging are now often being used in daily clinical practice to answer specific questions. The ability to detect and characterize CNS pathology rapidly continues to improve with advances in technology and with the development of newer pulse sequences. The trend is always toward obtaining superior images with faster imaging.
CONTRAST VERSUS NONCONTRAST
The question of whether to administer contrast is a common one. Contrast agents used in CT and MRI have risk factors, and screening for
possible adverse events must be done before ordering a study with contrast.
possible adverse events must be done before ordering a study with contrast.
▪ SPECIAL CLINICAL POINT: Contrast is not typically necessary when evaluating for acute ischemia or in the setting of trauma. Contrast should be considered when an underlying infectious/inflammatory process or neoplasm is suspected.
Iodinated contrast agents used with CT have the potential to elicit allergic reactions and can be nephrotoxic in patients with poor renal function. Preventive measures including steroid pretreatment, in patients with a known contrast allergy, and hydration, in patients with compromised renal function, are often performed. The contrast agents used for MRI are molecular forms of gadolinium. Although gadolinium-based contrast agents have a lower propensity to cause allergic reactions, recent literature has associated nephrogenic systemic fibrosis (NSF) with exposure to gadolinium in patients with renal impairment. As a result, an accurate medical history and renal function tests (blood urea nitrogen and creatinine) are often indicated prior to contrast administration of either iodinated CT contrast or gadolinium-based contrast agents.
▪ SPECIAL CLINICAL POINT: Enhancement in abnormal tissue is related to breakdown of the blood-brain barrier with leakage of contrast. Almost any lesion can demonstrate an element of enhancement, particularly mild, linear enhancement along its periphery. However, nodular, solid enhancement is more suggestive of an underlying neoplasm.
There are, of course, exceptions as certain nonneoplastic lesions can have nodular enhancement such as tumefactive multiple sclerosis. In addition, not all neoplasms enhance. Specifically, low-grade astrocytomas typically do not enhance.
As discussed in the section on CT versus MRI, CTA and MRA are also used to evaluate the vasculature. Of note, MRA can be done without contrast using time of flight (TOF) technique. Contrast-enhanced MRA can be performed in cases where the TOF technique is limited due to artifact or more accurate characterization of a stenosis or lesion is warranted. Another use of contrast is in CT myelography where contrast is injected into the thecal sac followed by CT of the spine. This is particularly useful when MRI is contraindicated or in the postsurgical patient where MRI can be limited due to metallic artifact of underlying hardware.
HERNIATION AND MASS EFFECT
The first step in evaluating the brain is to determine if there is impending herniation that would indicate emergent intervention. On axial imaging, it is useful to evaluate the ventricles and basal cisterns to determine whether they are effaced (compressed), suggesting mass effect or herniation. Sagittal and coronal reconstructions can often also be performed to directly visualize the degree of herniation.
Effacement of the fourth ventricle is a marker for tonsillar herniation, particularly if the process is supratentorial (a lesion in the cerebellum can efface the fourth ventricle without necessarily resulting in overt tonsillar herniation). Evaluation of the posterior fossa with newer CT technology is much better than on older scanners where the foramen magnum was obscured by streak artifact. In addition, if there is any question, sagittal reconstructions can be performed to directly visualize the tonsils. Effacement of the quadrigeminal plate cistern (behind the superior and inferior colliculi) is a marker for transtentorial herniation. If the lesion is supratentorial, it signifies downward herniation across the tentorium. If the lesion is infratentorial, it signifies upward herniation across the tentorium. Effacement of the suprasellar cistern is a marker for uncal herniation (another form of transtentorial herniation), where the medial temporal lobe protrudes into the suprasellar cistern, over the tentorium. Shift of the midline structures and septum pellucidum is usually more obvious with displacement of the lateral ventricles/third ventricle across the midline. This represents subfalcine herniation. When this is more advanced, the foramen of Monro becomes effaced, and dilatation of the contralateral
lateral ventricle develops (ventricular trapping) (Fig. 4.2).
lateral ventricle develops (ventricular trapping) (Fig. 4.2).
Herniation is problematic for a variety of reasons. Parenchyma and other neural structures can be compressed, and vessels can be compromised. Tonsillar herniation compresses the medulla and can depress the centers for respiration and cardiac rhythm control. Transtentorial herniation can compress the posterior cerebral arteries and cause infarction. It can also result in midbrain compression and hemorrhages, likely from tearing of small parenchymal vessels (Duret hemorrhage) (Fig. 4.3).
The mass effect may not be severe enough to result in herniation but can efface local sulci and adjacent cisterns or portions of the ventricles. Although this may be a subtle finding, it is important as it may be the only clue to suggest adjacent pathology that is not otherwise directly visualized. Mass effect in general indicates that the underlying process is active. For example, edema and gliosis/encephalomalacia will both demonstrate decreased density on CT, decreased signal on T1-weighted MRI, and increased signal on T2-weighted MRI. Edema, however, will result in mass effect with displacement/compression of adjacent structures. Gliosis/encephalomalacia will result in the opposite effect with widening of the adjacent CSF spaces due to the loss of parenchymal volume.
HYDROCEPHALUS VERSUS VOLUME LOSS
After determining whether the ventricles and sulci are effaced or displaced, signifying mass effect, one should make a judgment as to whether the ventricles and sulci in general are normal in size. When the ventricles are enlarged, it can be due to volume loss or hydrocephalus. If the ventricles are enlarged and the sulci are also enlarged, it suggests volume loss either due to atrophy or lack of development of the parenchyma (in pediatric patients). In the closed intracranial system, when there is loss of volume of the parenchyma, the CSF spaces diffusely must widen to compensate for the space previously taken up by the parenchyma. If the ventricles are enlarged but the sulci are not widened, or especially when they are narrow, it suggests hydrocephalus with the enlarged ventricles taking up space and causing effacement of the surrounding sulci (Fig. 4.4).
If hydrocephalus is established, the next step is to determine whether the cause is noncommunicating (obstructive) or communicating. Obstructive hydrocephalus refers to hydro cephalus where the obstruction of CSF drainage is along the ventricular outflow. Typically this is at the cerebral aqueduct or foramen of Monro, due to the smaller caliber of these passages. Communicating hydrocephalus refers to obstruction at the level of the arachnoid villi as the CSF drains into the dural venous sinuses. This can be due to such etiologies as infectious/inflammatory meningitis, subarachnoid hemorrhage (SAH), and neoplasm (carcinomatous meningitis). In communicating hydrocephalus, all of the ventricles are enlarged.
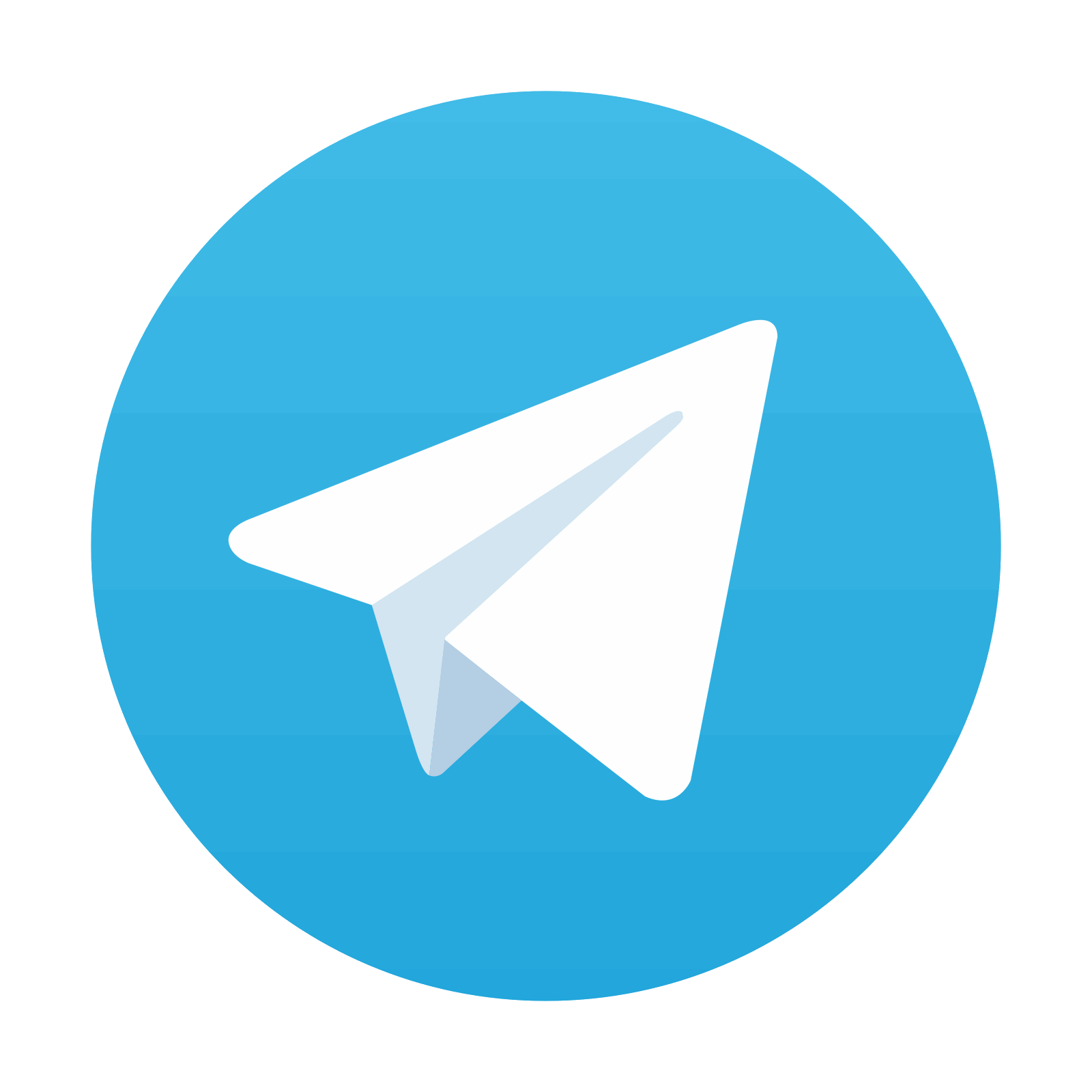
Stay updated, free articles. Join our Telegram channel

Full access? Get Clinical Tree
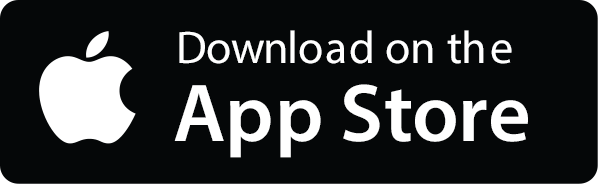
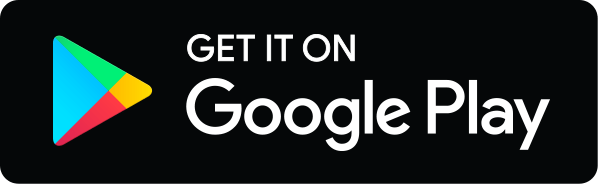