GABAA and GABAB Receptor-Mediated Inhibitory Synaptic Transmission
Robert L. MacDonald
Istvan Mody
Introduction
The neurotransmitter γ-aminobutyric acid (GABA) is the most abundant inhibitory neurotransmitter in the central nervous system (CNS). GABAergic inhibition is the primary form of fast inhibition in the forebrain, and can mediate both presyn- aptic and postsynaptic inhibition. GABA-mediated presynaptic inhibition occurs when GABAergic nerve terminals release GABA onto presynaptic nerve terminals, resulting in a reduction of neurotransmitter release. In contrast, GABAergic postsynaptic inhibition is mediated by the interaction of the neurotransmitters with specific postsynaptic receptors. GABA binds to two distinct types of GABA receptor, GABAA,C receptors and GABAB receptors, to produce neuronal inhibition. GABAA and GABAC receptors are ligand-gated chloride ion (Cl–) channels from the cys loop family of receptors, and GABAB receptors are seven-transmembrane domain receptors from the G-protein–coupled receptor (GPCR) family. Reduction of GABAergic inhibition because of mutation of GABAA receptors has been shown to be associated with several types of idiopathic generalized epilepsies, including childhood absence epilepsy, generalized epilepsy with febrile seizures plus, and juvenile myoclonic epilepsy.70,71 Enhancement of GABAergic inhibition is the basis of action of a number of antiepileptic drugs (AEDs).
γ-Aminobutyric Acid
GABA is synthesized in and released from the presynaptic terminals of GABAergic neurons. Glucose is the primary precursor of GABA, but pyruvate and other amino acids can also serve as precursors when metabolized in the Krebs cycle.79 The immediate precursor of GABA is glutamate, which can be produced from glutamine by glutamate synthetase or from α-ketoglutarate. Glutamic acid is then decarboxylated to form GABA. GABA is degraded by the enzyme GABA transaminase. GABA transaminase converts GABA into succinicsemialdehyde. This reaction involves removal of an amine group from GABA and transformation of α-ketoglutarate into glutamate. Therefore, as the GABA is degraded, its precursor molecule is formed, ensuring precursor availability for further synthesis. Succinicsemialdehyde is then degraded by the enzyme succinicsemialdehyde dehydrogenase (SSADH) to form succinic acid. This synthetic and catabolic pathway is called the GABA shunt. The shunt is thought to operate by an interaction with adjacent glia. GABA is released presynaptically and is taken up by high-affinity GABA transporters in nerve terminals and glia. In glia, GABA is degraded to succinicsemialdehyde, thus generating glutamate. Glutamate is then converted to glutamine by glutamine synthetase. It is believed that glutamine can then diffuse from the glia into nerve terminals, where it then again converted by glutaminase into glutamate, thus providing a substrate for GABA synthesis. GABA transaminase and SSADH are thought to be bound to mitochondria, whereas glutaminase and GAD are free in the terminal cytoplasm. Glutamine synthetase occurs free in the glial cytoplasm.
GABAA Receptors
GABAA receptors are macromolecular proteins from the cys loop family of ligand-gated ion channels that include the nicotinic acetylcholine receptor (nAChR), glycine receptor, and serotonin 5–HT3 receptor. GABAA receptors contain multiple specific binding sites including sites for GABA, antagonists such as bicuculline and picrotoxin, AEDs including barbiturates and benzodiazepines, and the anesthetic steroids. GABAA receptors form a Cl–-selective channel.73,90
GABAA Receptor Molecular Biology
GABAA receptors are hetero-oligomeric pentamers that form a transmembrane Cl–channel. Seven GABAA receptor subunit families (α, β, γ, δ, π, ε, θ) have been identified,90 and each subunit family is composed of one or more subtypes: (α1–α6), (β1–β3), (γ1– γ3), (δ1), (ε1), (π1), and (θ1). In addition, several splice variants have been reported. An additional subunit, the ρ subunit, is highly homologous to the GABAA receptor subunits but primarily forms retinal receptors that have been classified as GABAC receptors. Members of the same GABAA receptor family share approximately 70% to 80% sequence homology, whereas members of different families share 30% to 40% sequence identity. The number of potential GABAA receptor isoforms that could be formed from the 12 α, β, and γ subtypes alone is staggering. However, it has been demonstrated that not all potential subtype combinations assemble to create functional GABAA receptors,3,4 and the α1β2γ2 receptor is likely the dominant native benzodiazepine-sensitive isoform. The stoichiometry of native GABAA receptors has shown to be 2α2β1γ, with an assembly pattern of γ-β-α-β-α, as seen from the synaptic cleft.6,26,57,110
GABAA Receptor Structure
GABAA receptor subunits are about 450 amino acids in length and have a large N-terminus of approximately 200 amino acids that is shaped by the signature cysteine disulfide bridge and is likely an extracellular domain. The GABA binding site is at the two α/β subunit interfaces.101 Hydropathy analysis predicted four transmembrane-spanning domains (M1–M4) of about 20 amino acids in length, which have the highest degree of amino acid sequence homology across subunit
families and across the cys loop family in general. The M2 domain is thought to form the GABAA receptor channel pore.26 A short (eight amino acids) cytoplasmic segment (M1–M2 linker) connects M1 and M2, and a slightly longer extracellular segment (M2–M3 linker) connects M2 to M3. Also, a highly variable large cytoplasmic loop spans between M3 and M4 and contains consensus sequences for protein phosphorylation and protein–protein interaction that vary among subtypes. This domain is important for receptor trafficking and surface clustering through interactions with cytoplasmic proteins.
families and across the cys loop family in general. The M2 domain is thought to form the GABAA receptor channel pore.26 A short (eight amino acids) cytoplasmic segment (M1–M2 linker) connects M1 and M2, and a slightly longer extracellular segment (M2–M3 linker) connects M2 to M3. Also, a highly variable large cytoplasmic loop spans between M3 and M4 and contains consensus sequences for protein phosphorylation and protein–protein interaction that vary among subtypes. This domain is important for receptor trafficking and surface clustering through interactions with cytoplasmic proteins.
GABA Binding Site
Detailed structural information is available for the extracellular domain of cys loop receptors based on homology with the acetylcholine binding protein (AChBP),100 which has been crystallized and solved.21 The acetylcholine-binding pocket is formed at AChR subunit interfaces by three loops (A–C) from the positive side and three β-strands (D–F) from the negative side of the protomer. Using mutagenesis and the substituted cysteine accessibility method (SCAM) approach,1,15,48,86,120 the GABAA receptor binding pocket has been shown to be similar to that of the AChBP binding pocket.
GABAA Receptor Conduction Pathway
M2 likely lines the channel, and the amino acids lining the pore are likely α helical126 as is the nAChR M2.82 Using electron microscopy of crystalline postsynaptic membranes, the nAChR channel has been shown to be tapered, as viewed from the synaptic cleft, and is formed by five α-helical segments.82 The pore has a minimum diameter in the middle of M2 at residues L251 and V255, and this region has been identified as the channel gate.82 GABAA receptors also have a 9′L in the middle of M2, and mutation of the M2 9′L causes destabilization of the closed state,13 similar to the nAChR. Thus, the GABAA receptor gate is likely to be similar in location to that of the nAChR.
Coupling of GABA Binding to Channel Gating
Recent insights have emerged into the coupling of binding of GABA to GABAA receptors and subsequent gating of the channel. The extracellular N-terminal domain is directly connected to the membrane by M1; however, evidence from several sources has suggested that the N-terminal domain also interacts with the extracellular M2–M3 linker. Several disease mutations in the M2–M3 linker have been identified. In the nAChR, these mutations are associated with congenital myasthenic syndromes38; in the GABAA receptor, they are associated with a generalized epilepsy7,14; and in the glycine receptor, these mutations are associated with hyperekplexia.61 All these mutations decreased receptor current, suggesting a disruption in the coupling of agonist binding to channel gating. Based on the AChBP, it was suggested that loop 2 (and possibly loop 7) interact with the M2–M3 linker to couple GABA binding to gating.54 Using SCAM, gating was shown to induce a conformational change in and/or around the N-terminal half of the M2–M3 linker.9 Using mutagenesis of pairs and triplets of amino acids in the nAChR α subunit, a network of interacting amino acid residues have been shown to connect the preM1 region, loop 2, and the M2–M3 loop.64 Thus, coupling of agonist binding to channel gating may involve both direct (pre M1) and indirect (loop 2) pathways that connect to the M2–M3 loop. The gating process has been further clarified using electron microscopy of nAChRs.82,116 Loop 2 of both α subunits is positioned such that it contacts the distal M2, just before the beginning of the M2–M3 loop. Binding of ACh induces both loop 2s rotate by 15 degrees about an axis passing through the disulfide bridge, normal to the membrane. The loop 2 rotations are associated with M2 rotations, which are translated down M2 to the gate, presumably causing the gate to open. Thus the major transduction of binding to gating appears to pass in the α subunits from loop 2 to the distal M2 and then to the M2 gate.
GABAA Receptor Desensitization
Receptor desensitization occurs when agonist-evoked current declines during continued agonist application. No clear structural basis exists for desensitization of cys loop receptors. Desensitization of nAChRs has been studied using the SCAM technique.124 The “desensitization gate” was suggested to include the closed gate, but to extend further (more toward the extracellular portion) into M2. GABAA receptor subunit composition influences current desensitization rates.37,97,117 In glycine receptors, desensitization is influenced by amino acids at the distal end of M1, leading to the hypothesis that this region and the M1–M2 linker served as a hinge involved in the gating of the channel.69 Using subunit chimeras constructed with N-terminal δ sequence spliced to γ2L sequence at various points within M1 and M2, and using rapid agonist application, fast desensitization was demonstrated to depend on the structure of M1 and proximal N terminus.12
GABAA Receptor Pharmacology
GABAA receptor pharmacology has been shown to be quite complex.73,90 To fully activate the receptor, two molecules of GABA are required to bind to two independent sites on the GABAA receptor channel at the two α/β interfaces. In addition to GABA, a number of GABA analogs can activate the receptor, including the plant alkaloid muscimol and the synthetic GABA agonist tetrahydroisoxazolopyridinol (THIP). GABAA receptor currents can be competitively antagonized by the convulsant drug bicuculline and noncompetitively antagonized by the convulsant drug picrotoxin. GABAA receptor current can also be noncompetitively reduced by the convulsant antibiotic penicillin, which enters the GABAA receptor channel to produce a fast open-channel block. The antiepileptic benzodiazepines and barbiturates enhance GABAA receptor currents, but through different binding sites on the GABAA receptor. In contrast, the convulsant β-carbolines, such as methyl 6,7–dimethoxy-4–ethyl-β-carboline-3–carboxylate (DMCM), decrease GABAA receptor current. A number of compounds, including CL 218872, inverse agonist β-carbolines, and imidazolpyridines (zolpidem), bind to the benzodiazepine site. In addition to binding benzodiazepine receptor agonists, such as diazepam, and inverse agonists, such as DMCM, the receptor binds a benzodiazepine receptor antagonist, flumazenil, which binds to the receptor and antagonizes the actions of benzodiazepine receptor antagonists and inverse agonists but has little intrinsic activity. In addition to the preceding allosteric regulators, certain anesthetic and naturally occurring neuro- steroids, including alfaxalone, pregnenolone, and androsterone, alter GABAA receptor current. Most of these anesthetic steroid compounds bind to a specific site on the GABAA receptor to enhance current.
GABAA Receptor Physiology
GABA binds to GABAA receptors and evokes openings that occur in bursts.74 When the channel opens, it opens to a main conductance level of 27 pS and to two smaller, subconductance levels.16 GABAA receptor channels are permeable to a number of anions and exhibit a conductance sequence of CI–>Br–>SCN–>F–. GABAA receptor channels are almost exclusively Cl selective, with a permeability ratio of potassium (K) to Cl ions smaller than 0.05. The permeability sequence for large polyatomic anions suggests that open
GABAA receptor channels have effective pore diameters of about 5.6 Å.
GABAA receptor channels have effective pore diameters of about 5.6 Å.
Rapid application of GABA to outside-out patches from hippocampal neurons or to HEK293 cells expressing α1β3γ2L receptors evokes rapidly rising (1–2 msec) currents that then decay over three to four phases of desensitization.14,25,45 Following GABA application, the currents deactivate over two phases. GABAA receptor subunit composition influences current desensitization rates.14,37,97,117 For example, addition of the δ subunit to α1 and β3 subtypes significantly reduced both the rate and extent of desensitization relative to α1β3γ2L receptor currents.45
Ensemble currents (whole cell or macropatch currents, inhibitory postsynaptic currents [IPSCs]) observed experimentally represent the summed behavior of individual channels. A complete mechanistic understanding of GABAergic inhibition requires analysis at the level of single channels. GABAA receptor single channels exhibit complex patterns of activity. GABA increases the probability of channel opening and, after the channel opens, it closes and rapidly reopens in bursts of openings. Increasing GABA concentration increases average open and burst durations. Detailed kinetic analyses of channel behavior have been obtained for native74,87,115,122 and recombinant23,39,45 GABAA receptors, demonstrating multiple open and closed states occurring in bursts. Kinetic models were proposed to account for these properties, with connections of discreet kinetic states (Markov modeling) and incorporating two sequential GABA binding sites, three open states, and ten closed states.115
Recombinant α1βχγ2 receptors also exhibit multiple open and closed states.3,39,45 However, α1β1δ GABAA receptors have different gating properties, with only two open states that are similar to the two briefest open states of native and α1βχγ2 GABAA receptors. The model was used to interpret actions of modulators of GABAA receptor function, including benzodiazepines, barbiturates, neurosteroids, picrotoxin, penicillin, and β-carbolines.111,112,113,114 Using rapid application of GABA to outside-out patches from hippocampal neurons, Celentano and Wong25 demonstrated three desensitization phases. Jones and Westbrook52 demonstrated that GABAA receptors entered long closed states and subsequently reopened, suggesting that desensitized states prolong currents evoked by brief, synaptic-like GABA applications. The combination of single-channel properties and macroscopic kinetic properties, not easily extractable from single-channel recordings (such as activation, desensitization, deactivation), led to a comprehensive GABAA receptor model.45
Channel gating and desensitization have also been shown to affect GABA binding. GABA is locked onto GABAC and GABAA receptors by channel opening27 and, once bound to α1β3γ2L GABAA receptors, GABA is “trapped” by open, preopen, and desensitized states.14 This trapping of GABA on the receptor prolongs currents following brief GABA application, as occurs during IPSCs.52 In contrast, it has been reported that ACh is not locked on nAChRs by open states.43
GABAA Receptor Anatomic Distribution and Development
The widespread and overlapping distribution of GABAA receptor subunit cDNAs identified using in situ hybridization65,125 and the large number of subunits suggests that GABAA receptors exist in vivo in multiple isoforms. However, the colocalization of subtype mRNAs does not provide information on subunit assembly. Immunoprecipitation has been used to determine subtypes that coassemble in vivo.50,89,94,95,108 In the cerebellum, the most likely isoforms are α1βχγ2, α6βχγ2, α1α6βχγ2, α6βχ2δ, and α1α6βχδ receptors. The δ subunit is likely to combine with the α6 subtype in the cerebellum and with the α4 subtype in the thalamus, and based on coexpression, it may also combine with the α1 subtype in the cortex, thalamus, hippocampus, and olfactory bulb. Characterization of GABAA receptor subunit expression patterns using in situ hybridization has been reported in the developing63 and adult62,125 rodent brain. A detailed immunohistochemical study identified general anatomic patterns as well as cell-type specificity within regions and gross subcellular localization (soma versus neurite membranes).94
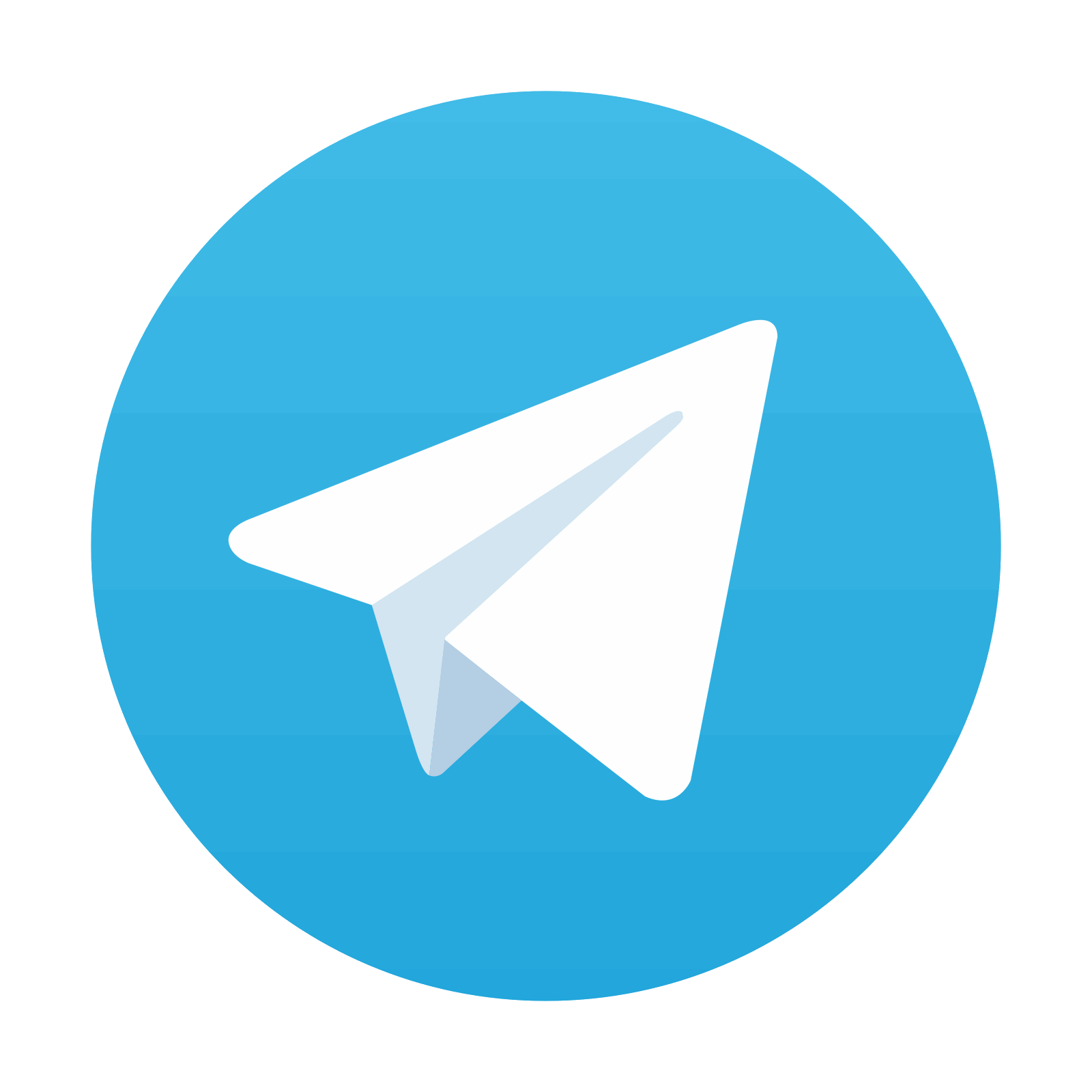
Stay updated, free articles. Join our Telegram channel

Full access? Get Clinical Tree
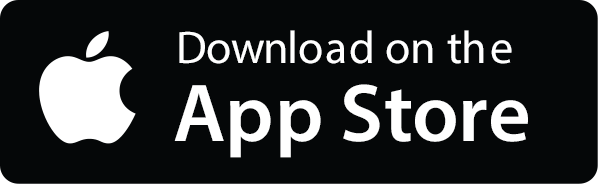
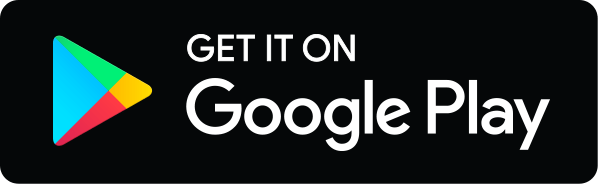
