Genetics of Juvenile Myoclonic Epilepsy: Faulty Components and Faulty Wiring?
Martina Durner*
Deb Pal*,†
David Greenberg‡
*Division of Statistical Genetics, Department of Biostatistics, Mailman School of Public Health, Columbia University, New York, New York
†Clinical and Genetic Epidemiology Unit, New York State Psychiatric Institute, New York, New York
‡Mailman School of Public Health and New York State Psychiatric Institute, Columbia Genome Center, Columbia–Presbyterian Medical Center, New York, New York
Introduction
Several years ago, juvenile myoclonic epilepsy (JME) was seen as the ideal model form of epilepsy to unravel the presumed genetic origins of generalized epilepsies with unknown causes, the epilepsies we called idiopathic. JME showed a strong genetic component that was similar to the genetic contribution seen in other age-dependent idiopathic generalized epilepsies (IGE). The argument, however, was that JME is the most homogenous epilepsy syndrome, easily and unambiguously identifiable through its unique clinical manifestation of myoclonic jerks experienced shortly after awakening. Nongenetic contributing factors could not be identified, and JME was therefore called the “prototype of idiopathic generalized epilepsy” (1a, Janz 1969).
Rapid progress in genetic molecular methods promised a quick success in finding “the” JME gene. In hindsight, this expectation can be seen as having been overly optimistic, underestimating the complexity of what was assumed to be a “simple” epilepsy syndrome. Subsequent research brought some successes, but also raised even more questions.
In this paper, we try to point out some of these questions. We also advocate taking a new look on how to approach the enormous challenge of not only finding the genetic basis of JME but also other epilepsy genes, since the problems encountered in the JME work are not unique to JME and occur in other IGE syndromes as well.
Are we Looking for “the” Juvenile Myoclonic Epilepsy Gene?
When gene searches for epilepsy first began, grant applications generally had as one of the specific aims “to identify the gene for (choose the form of epilepsy).” Almost always, a positive family history for seizures was found in many patients, otherwise there would be little justification for a genetic study. However, the fact that the overall pattern of seizures in families was not usually not consistent with a simple gene inheritance (either dominant or recessive) was largely ignored and the concept that a single gene that did not manifest itself in all gene carriers was introduced, that is, reduced penetrance. This view prevailed despite the fact that the data indicated that this concept is too simplistic and that more than one gene was likely to be involved in the explanation of the patterns seen in common JME (see below).
Another argument for questioning the concept of a single gene causing JME is the evidence for genetic heterogeneity in JME. Even though JME was chosen because it was thought to be a homogeneous, clearly defined disease, it became obvious, early on, that many patients, although they had similar clinical characteristics, did not always share the same genetic basis. Evidence for linkage was reported for several chromosomal locations and genetic heterogeneity was evident from the findings on chromosome 6q21 (1,2,3,4,5), 6p12 (6,7), and 15p (8,9,10). Cossette et al. (11) were the first to show that a mutation in a gene, GABRA1, encoding the alpha1 subunit of the gamma-aminobutyric acid receptor subtype A (GABAA cosegregated in a large French–Canadian family with many family members affected with JME. Sander et al. (12) found negative evidence for linkage to the location of GABRA1 on chromosome 5q in all their JME families and we have sequenced 45 JME patients for GABRA1 without finding any sequence variations.
To summarize, it has become clear that we have to revise our expectations of finding the JME gene. Most likely there are several genes that contribute to JME and many are most likely neither necessary nor sufficient to cause JME on their own. Some of these genes will be quite common in the general population, but as long as other (genetic, epigenetic or environmental) factors are not present, the disease will not manifest itself. To make matters even worse, these genes could act in several different combinations making it hard to detect their effect in different datasets due to random variation in the collection of pedigree data (13).
What Genetic Models Might Explain the Current Observations in Common Epilepsies?
Single-Gene Model
As mentioned above, the notion that we should expect to find one gene for JME persist despite the fact that the observed patterns of inheritance do not support a single-gene model for JME or other IGE. The single-gene model predicts that either one-half or one-quarter of offspring of at-risk matings will be affected with the trait. (Below, we discuss penetrance.) This is clearly not the case in IGE. Families showing clear dominant inheritance or clear recessive inheritance are quite rare. The common epilepsy families show segregation ratios (which, crudely stated, are sibling risk ratios corrected for ascertainment bias) on the order of 0.08 to 0.12, far lower than predicted by single gene models of 0.25 or 0.50.
Would a Mixture of Cases, in Which Some Families have a Genetic form and Some Nongenetic (sporadic) form, Explain the Data?
Such a model, by itself, would explain the observed segregation ratio, but little else. First, simple clustering analysis would reveal clusters of families, some with multiple affected members and some with only one affected member, if the disease showed simple inheritance. Second, under such a model, almost any linkage study would quickly reveal the cosegregation of markers and disease, even if there were heterogeneity in the data. Third, the MZ twin concordance rate would be quite low, likely 50% or less because the majority of MZ twins would have the “sporadic” form of the disease. In fact, the MZ twin rate in IGE is higher than 80%, strongly suggesting that IGE is an almost purely genetic disease in almost all of the JME cases. Thus, a single-gene model, together with some families having an environmental form, will not explain the observed data.
Would Not Reduced Penetrance Explain the Results?
For most common diseases, the simple Mendelian models can be rejected because the observed segregation ratio is far too low. Thus, the concept of reduced penetrance was invented to explain this observation. In thinking of reduced penetrance, it is tacitly assumed that there is a single gene responsible for the disease but that “other factors” play a role in the gene’s expression. Usually, these factors are thought of as environmental. If the cause of reduced penetrance is environmental, then the MZ twin concordance rate should be a direct reflection of the penetrance, e.g., if the penetrance is 50%, as measured by the segregation ratio, then the MZ twin concordance rate should be 50%. However, the “other factors” could also be purely genetic; that is, other genes could play a role in the expression of the disease. If that were the case, then the segregation ratio would not be the 25% or 50% predicted by the single-gene model but would be a function of the combined segregation ratios of multiple genes and highly dependent on the gene frequencies of the genes. However, when there is no environmental influence, the model also predicts that the MZ twin concordance rate will be 100%, because MZ twins share 100% of their genes in common. This is, in fact, what is seen in IGE. The high concordance rate in MZ twins points to little environmental influence and an almost exclusive genetic basis for a “reduced penetrance.” This is also confirmed by the fact that no studies have identified any environmental agents that are correlated with IGE.
Oligogenic Model
JME is one of the few examples of an epilepsy in which a formal segregation analysis was reported (14). In that case, an oligogenic model in which two specific genes must be present for disease expression (an epistatic model) was compared to the segregation ratio observed in JME families, with excellent fit. These results do not exclude the possibility of more than two genes being involved, but it is difficult to distinguish epistatic models involving two loci from those involving more loci.
Thus, the existing data strongly suggests that there is little environmental influence in IGE but that there is “reduced penetrance.” These results can be best explained by an oligogenic model in which at least two genes must be present to manifest IGE.
Other Multigenic Models
One of the other models, that require multiple genes and is commonly cited, is the polygenic threshold model (PTM). The PTM assumes that many genes, each with an equal, but small, effect, contribute to disease expression. When the number of genes, out of the many possible genes, reaches a certain count, then the disease will be expressed. When this model was tested on early data on JME, it came quite close to being rejected (15). Finding any of these genes in a PTM model is extremely difficult because the influence of each gene is small and their combination will vary among families and patients. It means, ultimately, that everyone with the disease can have a different disease genotype.
If we modify the model so that we say that one gene predominates or provides major susceptibility but that an accumulation of other genes allows that one to act, we are back to a single-gene model, but with a so-called “polygenic background,” essentially a single-gene model with reduced penetrance, but reduced penetrance that is still entirely genetic.
A pure polygenic model however, and to a lesser degree a “polygenic background” model, have become untenable through the localization of several genes via linkage analysis or, even more compellingly, the identification of specific genes. Thus, the model that appears to explain most of the currently known data is an oligogenic model in which two or more genes must be present in order for epilepsy to manifest.
Should we use Single Large vs. Many Small Families?
The answer to this question is—it depends on what we are looking for. Heterogeneity and reduced penetrance are major problems in finding genes for common diseases. Large families were perceived to offer a way to eliminate those problems. The fact that it is one pedigree reduces the chance of heterogeneity and the fact that many members are affected means that reduced penetrance is less of a problem. This strategy has been quite successful so far in the apparent identification of ion-channel genes and neurotransmitter-related genes in epileptogenesis. However, contrary to commonly held belief, these single, large, high-density families with many affecteds that have a phenotype similar to those in families with more sparse “genetic loading” (i.e., families with low familial occurrence of seizures) did not provide us with answers for common forms of JME. These results are in line with findings in other common diseases. For example, the relevance of presenillins in common forms of Alzheimer’s Disease, or parkin in common forms of Parkinson’s Disease, etc., has not been established. While each of these findings provide a major step in elucidating potential pathways in these diseases and may lead to new treatment options, they have, thus far, not yet contributed to our understanding of the more common forms of disease. It appears that if we want to understand the underlying pathology of common forms of epilepsy, we have to study common families.
Even when studying large, high-density families, there are several difficulties. First, the variable phenotype in some of these families, especially the families with generalized epilepsy with febrile seizures (GEFS), point to the involvement of more genes, that together with the already discovered mutations in ion channel or GABA genes lead to the different phenotypes. Since we are limited to single rare families, each family does not have enough statistical power to detect genes that are involved in some, but not all, affected family members. Second, by requiring families to have many affected members, one is selecting for families that are more likely to have several independent disease genes segregating within the family, that is, families with intrafamilial heterogeneity (16). For example, in the GEFS family reported by Wallace et al. (17), several affected family members did not have a mutation in the SCN1B gene and were therefore labeled as “phenocopies.” Fortunately, the effect of those “phenocopies” was not strong enough to mask the detection of the major contribution in this family of a SCN1B mutation, a biological meaningful candidate gene. It might be more difficult to dissect the genetic contributions in families with increased intrafamilial heterogeneity and disturbances in less obvious candidate genes.
What Phenotype are we Studying?
Usually, genetic studies start out with a patient that is seen by a physician. A family history is then taken and family members are screened for the presence or absence of the trait under study. In families of JME patients however, a significant proportion of the family members that also have epilepsy do not have JME, but some other kind of IGE. Depending on the study, only 15% to 30% of affected family members have myoclonic jerks, 15% to 30% have absence seizures and 50% to 85% of affected family members have GTCS (18,19). On the other hand, most of the JME patients themselves also have GTCS, in addition to the myoclonic jerks; about one-third have absence seizures. This raises the question of what phenotype exactly are we studying? Are we indeed looking for a “JME” gene? Or are we looking for a more general gene that nonspecifically lowers seizure threshold? Does the same genetic disturbance underlie JME, absence epilepsy or “pure” grand-mal epilepsy and just shows variable expressivity?
This question was largely ignored until we started to question the construct of clinical epileptic syndromes that are based on a leading symptom, but also allow for additional symptoms that may or may not occur. We thought for genetic purposes, it might be better to focus on the individual symptoms, in our case, the different seizure types. We already knew that IGE is most likely caused by several genes. We postulated that there are genes common to all IGE that merely lower seizure threshold and there are specific genes that are necessary for both the manifestation of seizures and determination of specific seizure types. We analyzed our genetic data of adolescent-onset IGE, comprising of JME, juvenile absence epilepsy (JAE), and epilepsy with pure GTCS (EGTCS) according to seizure types (i.e., myoclonic seizures, absence seizures, and GTCS) and found evidence for a common gene for all these seizure types on chromosome 18, but also locations of “individual” genes for myoclonic jerks on chromosome 6 and for absence seizures on chromosome 5 (13). These findings provided support for an intriguing hypothesis and unified the observation of different seizure types in families and individual patients. Epidemiologic studies further support this notion that it is not the epilepsy syndrome, but the seizure type of myoclonic and absence seizures that have distinct genetic effects (20).
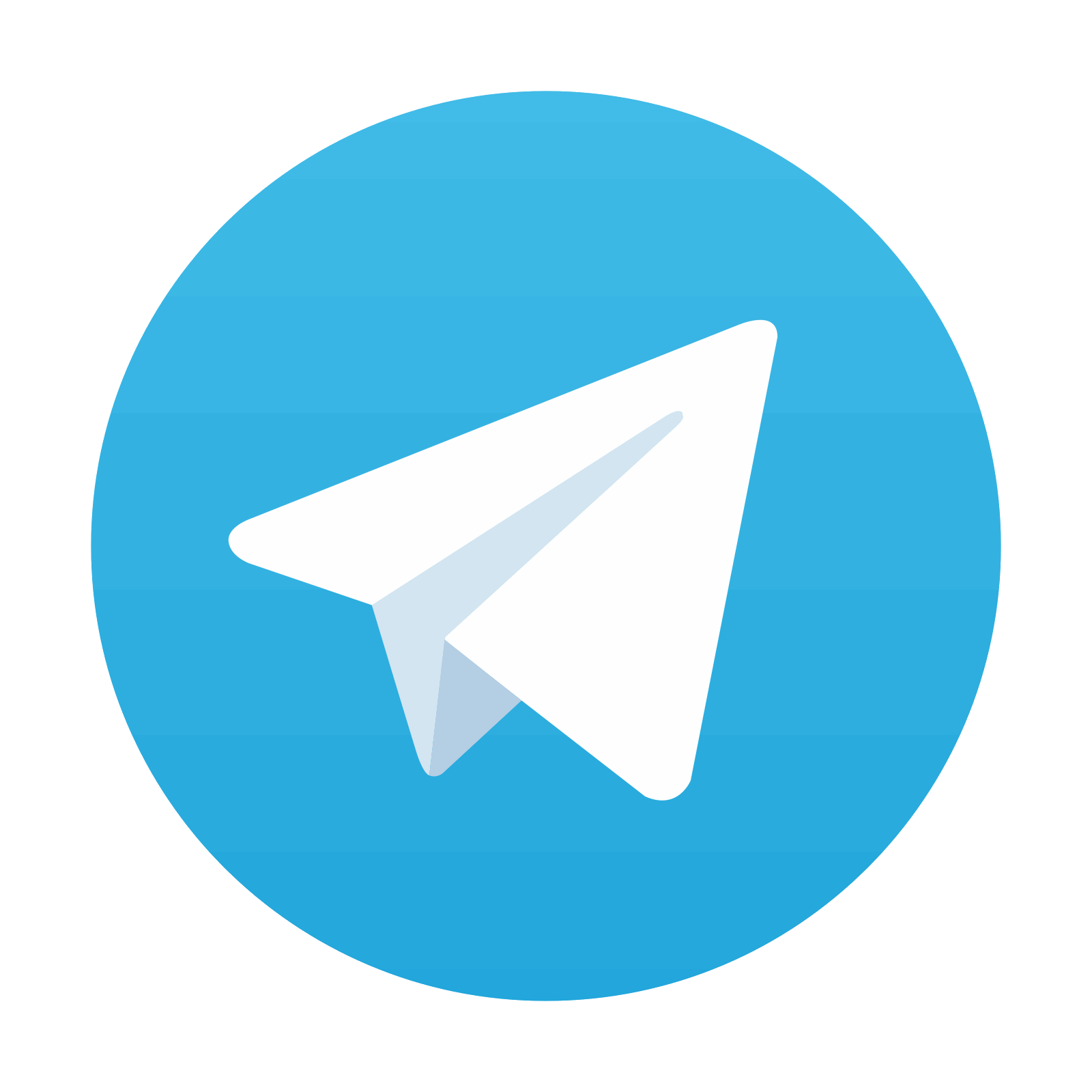
Stay updated, free articles. Join our Telegram channel

Full access? Get Clinical Tree
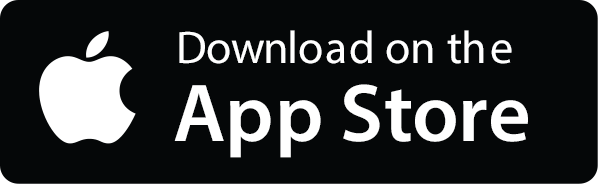
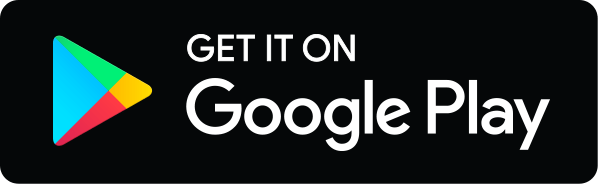