Introduction
Hyperglycemia is common in acutely ill patients. In the past, stress hyperglycemia was considered to be a beneficial adaptive response. However, prospective observational and clinical trial data show a consistent and clear association between hyperglycemia and increased morbidity and mortality in all critically ill populations and in particular those with acute brain injury, stroke, and subarachnoid hemorrhage. Glycemic control therefore has become an important aspect of critical care. However, the optimal level of glycemic control in critical illness remains in debate since Van den Berghe et al. published their study conclusions in 2001 showing that strict glucose control was associated with reduced mortality. In these randomized trials intensive insulin therapy (IIT) was used to target a blood glucose of 80 to 110 mg/dL. Studies since then have not been able to duplicate these results (Normoglycemia in Intensive Care Evaluation-Survival Using Glucose Algorithm Regulation [NICE-SUGAR]), and so a more liberal glucose target of 140 to 180 mg/dL is favored. In addition, an association between hypoglycemia that can be caused by IIT, and high glycemic variability and poor outcome also is apparent, further emphasizing the importance of glucose control. The safety and efficacy of IIT may be influenced by patient-related and intensive care unit–related variables. In particular, nutrition is closely associated with glycemic control. Furthermore, guidelines recommend use of early nutritional support, preferably enteral nutrition. However, optimal energy requirements and the timetable of nutritional support still are not fully resolved, in part because energy expenditure needs may not be met with enteral nutrition only, and there are different recommendations for parental nutrition in Europe and North America. This chapter addresses the importance of glucose control: how to monitor glucose levels in ICU patients, practical aspects of glucose control in ICUs, nutrition therapy, and methods to calculate nutritional requirements in critical care.
Glucose
Plasma glucose usually is between 60 and 100 mg/dL before meals and up to 150 mg/dL after a meal. Hypoglycemia is defined as a blood glucose less than 50 mg/dL. Several factors such as malnutrition, cirrhosis, renal failure, and alcoholic ketoacidosis or drugs such as pentamidine, haloperidol, bactrim, or salicylates increase the risk of developing hypoglycemia. Traditionally, acute hyperglycemia was defined as a random glucose concentration greater than 200 mg/dL. In 2010, the American Diabetes Association (ADA) proposed a threshold of 140 mg/dL (7.8 mmol/L; ADA Standards of Medical Care in Diabetes–2010 http://care.diabetesjournals.org/content/33/Supplement_1/S11.full ). Hyperglycemia may be considered as mild (110-180 mg/dL), moderate (180-400 mg/dL) or severe (>400 mg/dL). Table 14.1 lists the diagnostic criteria for diabetic ketoacidosis or hyperosmolar hyperglycemic state. In the hospitalized patient stress and medication (e.g., steroids) are factors associated with elevation of blood glucose. In addition, there can be relative insulin resistance after administration of catecholamines or glucocorticoids.
Criteria | Diabetic Ketoacidosis | Hyperosmolar Hyperglycemic State | ||
---|---|---|---|---|
Mild | Moderate | Severe | ||
Plasma glucose (mg/dL) | >250 | >250 | >250 | >600 |
Arterial pH | 7.25-7.30 | 7.00-7.24 | <7.00 | >7.30 |
Serum bicarbonate (mEq/L) | 15-18 | 10 to <15 | <10 | >15 |
Urine ketones | Positive | Positive | Positive | Small |
Serum ketones | Positive | Positive | Positive | Small |
Effective serum osmolality * | Variable | Variable | Variable | >320 |
Anion gap † | >10 | >12 | >12 | Variable |
Level of consciousness | Alert | Alert/drowsy | Stupor/coma | Stupor/coma |
* Effective serum osmolality = 2[measured Na+] + glucose/18.
Assessment of Glucose in the Intensive Care Unit
Blood to measure glucose can be obtained from a variety of sites (e.g., a fingerstick device, venipuncture, or through an intra-arterial or venous catheter). Fingersticks may be inaccurate when there is edema, hypoperfusion, or anemia, whereas capillary blood glucose monitoring may not be accurate in shock. In addition, care to prevent contamination by intravenous solutions is needed when using samples from arterial or venous catheters because pseudohyperglycemia may be present if a blood sample is drawn from a line where the patient is receiving dextrose 5% in water (D5W) or total parenteral nutrition (TPN). Laboratory analysis of plasma is the best means to analyze blood glucose levels. However, this technique may be too slow for care of critically ill patients. Many hospitals now have laboratories operated by licensed medical technologists in, or close to, the ICU that allow rapid assessment of blood gases, electrolytes, hemoglobin, prothrombin time (PT), and glucose through a blood gas analyzer or “stat” platform (e.g., i-STAT [Abbott Point of Care, Princeton, NJ], Radiometer ABL 825, or RapidLab 865). These devices generally are very accurate and can be used interchangeably with core laboratory devices, but turnaround time can still be a limiting factor.
Point-of-Care Testing
Glucose testing using point-of-care (POC) devices has become increasingly common in hospital and ICU settings as well as in the operating room and in the outpatient environment. In addition, there are many different self-monitoring blood glucose (SMBG) devices available for patients to use, for example, Accu-Chek Active and Accu-Chek Performa (Roche Diagnostics, Indianapolis, IN), OneTouch Ultra2 (LifeScan Inc., Milpitas, CA), MediSense Optium or Xceed (Abbott Diabetes Care Inc., Alameda, CA), and others. These devices all are subject to strict regulatory standards (i.e., International Organization for Standardization. In vitro diagnostic test systems: requirements for blood glucose monitoring systems for self-testing in managing diabetes mellitus. [ISO 15197:2003 http://www.iso.org/iso/home/store/catalogue_tc/catalogue_detail.htm?csnumber=26309 ; accessed Oct. 19, 2012.)
Although POC devices are used at bedside, they are licensed under laboratory medical directors and governed by several regulatory bodies such as Health Care Financing Administration (HCFA), The Joint Commission, Clinical Laboratory Improvements Amendments of 1988 (CLIA 88), the College of American Pathologists (CAP), and individual state departments of health, hospital policies, and procedure manuals for each device. This means use of the devices must follow regulation, and there needs to be proficiency testing for accuracy and testing of unknown samples provided by CAP or commercial sources for comparison to reference range values to calibrate and verify the device. Staff who use the devices also are expected to receive annual competency testing. POC monitors are reasonably accurate, particularly when blood from a catheter is used. However, POC glucometers can be inaccurate (20%) when patients have low blood glucose levels and can differ from laboratory values ; not all achieve ISO 15197 accuracy criteria. This can limit their use when tight glucose control is targeted. Consequently it may be better to examine blood glucose levels using central lab or blood gas analyzers rather than POC devices and when POC devices are used careful clinical evaluation of each system for glucose measurement is critical.
i-STAT Analyzer
This device can measure blood gases, electrolytes, glucose, and certain coagulation parameters through single-use sensors constructed using thin-film technology. Because the sensor film is thin, it can be used immediately and unlike thick film sensors does not require a calibration period. Waste, however, is a potential disadvantage of use.
GEM 3000
The GEM 3000 (Instrumentation Laboratories, Bedford, MA) has thick film sensors or electrodes in strips that can measure glucose, lactate, and blood gases. The sensors contain reagents and calibrators packed into small cartridges that are placed in the body of the analyzer. The cartridges can test multiple samples and the sensors are reusable. Samples with increased osmolalities, high protein, or high lipid volumes adversely affect the function. In addition, gallamine triethiodide, ethanol, sodium fluoride, potassium oxalate, acetaminophen, isoniazide, or thiocyanate may affect glucose or lactate levels.
Minimally Invasive Devices
Continuous monitoring sensors (e.g., CDI 500 Parameter Monitoring System, Terumo Cardiovascular Systems, Ann Arbor, MI) that are inserted into the bloodstream are available but their benefit still needs to be elucidated. These monitors based on optical technology are primarily used to measure blood gases and most commonly have been used in cardiac surgery. However, electrochemical sensors that can monitor glucose are available.
Microdialysis
In 2009 the Eirus system (Dipylon, Solna, Sweden) was CE-marked for continuous glucose and lactate monitoring in blood, and in 2011, the Eirus TLC catheter received its CE mark. This technology is based on microdialysis so no blood is removed from the patient, and it does not require a separate line because the device includes a central venous catheter. It allows second-by-second monitoring of glucose and lactate. The glucose value is presented on a monitor as a numeric value and a trend curve is provided. Studies have verified its technical feasibility, accuracy, and performance in the ICU. However, as of 2012 the device has not yet become commercially available.
Why Is Glycemic Control Important in the Neurocritical Care Unit?
In critical illness, release of stress hormones (e.g., epinephrine and cortisol), medications such as exogenous glucocorticoids or catecholamines, and release of inflammatory mediators (e.g., in sepsis or trauma) all inhibit insulin release and action. This results in stress hyperglycemia, defined as an increase in blood glucose in the presence of acute illness, through enhanced gluconeogenesis, inhibition of glycogen synthesis, and impaired insulin-mediated glucose uptake by tissues. In addition, intravenous dextrose (e.g., in parenteral nutrition or in antibiotic solutions), can exacerbate or contribute to hyperglycemia. Overall, hyperglycemia may be present in 40% of critically ill patients; 80% of ICU patients with hyperglycemia have no history of diabetes before admission.
Hyperglycemia during critical illness is associated with adverse outcome and an increased risk for infection, myocardial infarction, and neurologic and renal damage, particularly in patients who are not diabetic before admission. The effect of hyperglycemia also depends in part on patient pathology (i.e., ICU admission diagnosis). However, the impact of hyperglycemia on patients with preexisting diabetes is less clear even though diabetic patients are at greater risk for complications. In fact, in diabetic patients with elevated hemoglobin A 1c (HbA 1c ) levels (>7%), glucose levels that are desirable in other patients appear to be “unsafe” in diabetic patients with chronic hyperglycemia. Although the likelihood of hyperglycemia is greater in sicker patients, it appears that hyperglycemia itself is an independent factor associated with outcome particularly in stroke, both hemorrhagic and ischemic, and acute coronary syndromes. In addition, survival is inversely associated with the duration of hyperglycemia. However, the exact threshold at which elevated blood glucose is deleterious remains uncertain. The relationship between hyperglycemia and increased risk of worse outcome has driven research into glucose control in the ICU, the value and structure of which remain debated, in part because tight glucose control is associated with an increased risk for hypoglycemia that in turn is associated with adverse outcome and mortality. Furthermore, glucose variability also is associated with an increased risk of poor outcome. For example, Cueni-Villoz et al. observed that increased blood glucose variability during therapeutic hypothermia was associated with in-hospital mortality after cardiac arrest, independent of injury severity and mean blood glucose levels.
Consistent evidence from experimental and clinical studies demonstrates that hyperglycemia in the setting of acute neurologic injury is associated with worse outcomes; this concept is widely accepted in the management of critical neurologic illness in adults as well as children. Hyperglycemia at admission is present in almost half of acute stroke patients, and is an independent risk factor for mortality at 30 days, 1 year, and 6 years after stroke. Baird et al. found that serum glucose greater than 130 mg/dL during the first 72 hours after a stroke was associated with significant increase in infarct size as measured by magnetic resonance imaging (MRI) 3 to 6 days after the ictus. Likewise, increased blood glucose is a risk factor for death and disability after aneurysmal subarachnoid hemorrhage (SAH). For example, McGirt et al. observed that patients with blood glucose greater than 200 mg/dL for 2 or more days were seven times more likely to have a poor outcome at 10 months after SAH. Others have found that mean glucose concentrations greater than 140 mg/dL are associated with the development of symptomatic vasospasm after SAH. In traumatic brain injury (TBI) hyperglycemia also is associated with decreased survival, higher intracranial hypertension, and increased length of hospital stay.
Whether hyperglycemia in the setting of acute neurologic injury is simply an epiphenomenon or actually serves a protective purpose remains controversial. The stress response associated with neurologic injury can lead to hyperglycemia through liberation of catecholamines that induce gluconeogenesis and insulin resistance. This may be an adaptive and protective response because glucose is the primary source of energy in the brain. However, some have postulated that in the setting of anaerobic metabolism, hyperglycemia will result in the accumulation of lactate and an intracellular acidosis, a potential mediator of secondary injury. Conversely, if the supply of glucose, the main energy substrate of the brain, is inadequate, there could be insufficient fuel for glycolysis in already ischemic tissue. Termed the glucose paradox of cerebral ischemia , this conundrum makes determination of an exact target for plasma glucose concentrations in patients with severe neurologic injury difficult. The conundrum is further complicated by accumulating evidence that in some circumstances after acute brain injury lactate is used as a fuel in the brain and may even be protective.
Insulin Therapy in Critical Care
In 2001 and 2006 two single center randomized clinical trials demonstrated the association between IIT and improved outcomes in critically ill patients in surgical ICUs (SICU) and medical ICUs (MICU). In these trials patients were randomly assigned to IIT (target glucose 80-110 mg/dL [4.4-6.1 mmol/L]) or standard care (target glucose 180-200 mg/dL [9.9-11 mmol/L]). In SICU patients ( n = 1548), Van den Berghe et al. observed that an IIT protocol that treated hyperglycemia by administration of intravenous infusion insulin adjusted to maintain blood glucose between 80 and110 mg/dL was associated with reduced mortality during intensive care (8% for conventional therapy vs. 4.6% for IIT) especially among patients who were in the ICU more than 5 days. Moreover, IIT reduced in-hospital mortality (10.9% for conventional care vs. 7.2% for IIT), bloodstream infection, renal failure, critical-illness polyneuropathy, and transfusions. The greatest reduction in mortality was observed for deaths associated with multiple-organ failure with a proven septic focus. A follow-up MICU study ( n = 1200 patients) showed IIT was associated with prevention of newly acquired kidney injury, accelerated weaning from mechanical ventilation, and quicker discharge from the ICU and the hospital. In patients who received IIT, mortality was greater among those who were in the ICU less than 3 days but significantly decreased in those patients with MICU stays longer than 3 days. The mechanism by which IIT lowered mortality remains poorly understood and probably involves both glycemic and nonglycemic pathways. The potential nonglycemic benefits of insulin include myocardial protection, partial correction of abnormal serum lipid profiles, and the prevention of excessive inflammation.
However, other studies have not been able to duplicate the results of Van den Berghe et al. and in a meta-analysis that included 29 randomized trials with 8432 patients Wiener et al. found that tight glucose control (IIT) increased the risk of critical hypoglycemia in critically ill adult patients but did not reduce hospital mortality. In turn, hypoglycemia increases the risk of mortality. Although IIT was popular in the years immediately before and after 2000, recommendations ( Table 14.2 ) now target a blood glucose concentration between 140 and 180 mg/dL (8-10 mmol/L) according to NICE-SUGAR, published in 2009. In this randomized clinical trial 6104 patients were randomized to IIT (target blood glucose 81-108 mg/dL (4.5-6 mmol/L), or conventional glucose control (target <180 mg/dL [<10 mmol/L]). Ninety-day mortality was significantly less in the conventional glucose control group. In addition, severe hypoglycemia (<40 mg/dL) was rare when using conventional glucose control (0.5% vs. 6.8% in the IIT group). Other investigators also have observed that hypoglycemia is rare using the current American Association of Clinical Endocrinologists and American Diabetes Association (AACE/ADA) consensus recommendations that use a blood glucose target of 140 to 180 mg/dL in the ICU. However, there are differences, in particular glycemic control superimposed on different nutritional strategies, between the trials in Leuven and NICE-SUGAR that may limit direct comparison. Indeed it is conceivable that the safety and efficacy of IIT may be influenced by patient-related and ICU setting–related variables.
Year | Organization | Patient Population | Treatment Threshold | Target Glucose mg/dL | Definition of Hypoglycemia | Updated Since NICE-SUGAR Trial |
---|---|---|---|---|---|---|
2009 | American Association of Clinical Endocrinologists and American Diabetes Association | ICU patients | 180 | 140-180 | <70 | Yes |
2009 | Surviving Sepsis Campaign | ICU patients | 180 | 150 | Not stated | Yes |
2009 | Institute for Healthcare Improvement | ICU patients | 180 | <180 | <40 | Yes |
2008 | American Heart Association | Acute coronary syndromes | 180 | 90-140 | Not stated | No |
2007 | European Society of Cardiology and European Association for the Study of Diabetes | ICU patients with cardiac disorders | Not stated | Strict (refers to 80-110 mg/dL) | Not stated | No |
Insulin Therapy in Neurocritical Care
The potential mechanisms by which IIT is theorized to benefit the critically ill—attenuation of the cortisol stress response, improved immune cell function, reduced endothelial dysfunction, correction of abnormal lipids, prevention of toxicity to mitochondria, and reduced hyperglycemic axonal damage —may be relevant in patients suffering from acute neurologic illness. In a preplanned subanalysis of SICU patients, 63 of whom had isolated brain injury, Van den Berge et al. also observed that IIT reduced both the incidence of critical illness polyneuropathy and time on the ventilator. In the patients with isolated brain injury, 1-year functional outcomes were significantly improved in those patients in the IIT group, despite the admission Glasgow Coma Scale (GCS) score being higher in the conventional insulin therapy group. For MICU patients who needed intensive care for at least 7 days, IIT reduced the incidence of critical illness myopathy and neuropathy and was protective against prolonged mechanical ventilation. Subsequent studies suggest glycemic control can help reduce critical illness polyneuropathy or myopathy, although this may depend on the diagnostic criteria used.
Several studies have focused on glucose control specifically in patients with primary neurologic diseases (e.g., TBI and stroke); overall it does not appear that IIT improves outcome, whereas its use is associated with more frequent episodes of hypoglycemia that in some patients aggravates outcome. The UK Glucose Insulin in Stroke Trial (GIST) randomized patients with acute ischemic stroke to either conventional insulin therapy or IIT (72 and 126 mg/dL); 90-day mortality was similar in the two groups. Bilotta et al. randomized 78 SAH patients to conventional or IIT. The patients randomized to the IIT group had a significant decrease in infection but no difference in mortality or neurologic outcome. The same researchers randomized 97 patients with severe TBI to either conventional treatment for glucose greater than 220 mg/dL or IIT for (80-120 mg/dL). The patients treated with IIT had more episodes of hypoglycemia but rates of infection, poor outcome, and mortality were similar in the two groups.
Two randomized phase II trials of IIT for acute ischemic stroke were completed in the United States. The Treatment of Hyperglycemia in Ischemic Stroke trial aimed for glucose concentrations less than 130 mg/dL in the tight control group, and less than 200 mg/L in the loose control group. In the Glucose Regulation in Acute Stroke trial, the goals were 80 to 120 mg/dL and 80 to 180 mg/dL, respectively. In both, the investigators were able to achieve their targets for tight glucose control without significant hypoglycemia or other major adverse events.
Other data in primary neurologic injury raise concerns about the effects of IIT on cerebral metabolism. Microdialysis studies in patients with severe TBI or SAH suggest that tight systemic glucose control is associated with reduced cerebral extracellular glucose and increased risk of brain energy crisis. In addition, Vespa et al. reported that low extracellular cerebral glucose after TBI was associated with poor outcomes, even in the absence of increased microdialysate lactate. Similarly in patients with severe SAH, Oddo et al. observed that increased insulin dose independently predicted brain energy crisis after adjustment for intracranial pressure (ICP) and cerebral perfusion pressure (CPP) and that this in turn was associated with increased mortality. In addition, the relationship between brain interstitial and systemic glucose concentrations is complex and may change when the systemic glucose concentration is greater than 180 mg/dL. Other microdialysis studies suggest administration of insulin could impede the glucose supply of the brain and that brain energy metabolic crisis (elevated lactate-to-pyruvate ratio) may occur with acute reduction in serum glucose even when those levels are normal. Together these various data suggest that IIT in patients with already marginal serum glucose levels may be detrimental, and the optimal goal for a glucose level in the setting of acute neurologic injury remains to be elucidated. The available evidence suggests a less restrictive target for systemic glucose control (8-10 mmol/L; 144-180 mg/dL) may be reasonable. Furthermore the data emphasize the importance of monitoring blood glucose and the effects of therapy and that an approach that is perhaps more individualized to avoid both hypoglycemia and hyperglycemia is warranted until specific glycemic control guidelines for patients with severe brain injury in the neurocritical car unit (NCCU) are better defined. An example of a continuous insulin protocol is provided in Table 14.3 .
|
Blood Glucose Level (mg/dL) | Initial Insulin Infusion (U/hr) |
---|---|
>351 | 14 (plus 0.1 U/kg IV bolus) |
280-350 | 10 |
240-279 | 8 |
200-239 | 6 |
170-199 | 4 |
140-169 | 3 |
111-139 | 2 |
Beyond a Glucose Threshold
There are several areas of research beyond just the threshold at which to treat elevated blood glucose that are relevant to glycemic control: (1) treatment algorithms and patient safety mechanisms (see also Chapter 6 ), (2) use of computerized decision support systems to deliver insulin or development of continuous monitors with automated closed-loop systems, (3) glucose variability, (4) whether target levels should differ according to the indication for ICU admission or the stage of acute illness (i.e., a population-specific glycemic control strategy), and (5) the relationship between glucose, glycemic control, and nutrition. In addition, attention to ICU processes is important. For example, the risk of hypoglycemia associated with IIT is associated with nursing schedules and fatigue, whereas when nurses override clinical decision support systems for IIT they tend to use lower-than-recommended doses to avoid hypoglycemia. Finally bioinformatics systems can be used to facilitate integration of glucose control and nutrition.
Glucose variability, that is, changes in the measured level of blood glucose, may be of particular importance because it appears to be an independent or even casual factor associated with adverse outcome. This in part may explain the limited effect of IIT because IIT seems to increase glucose variability. On the other hand IIT also is associated with hypoglycemia, the severity of which is independently associated with increased mortality. However, there are no prospective studies that examine whether reduced glucose variability is associated with better outcome, and so insulin therapy is for the moment targeted to a glucose threshold rather than reduced glucose variability.
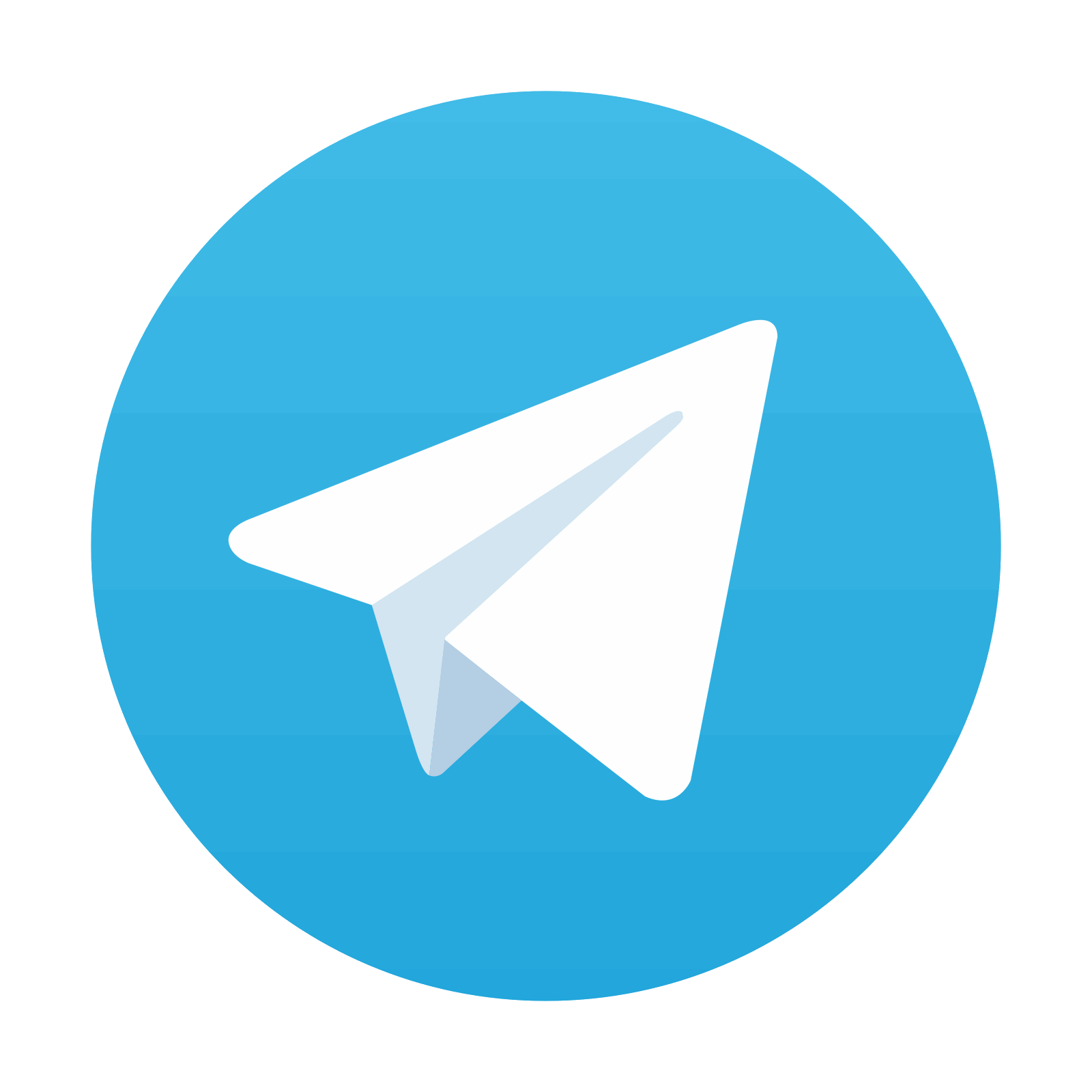
Stay updated, free articles. Join our Telegram channel

Full access? Get Clinical Tree
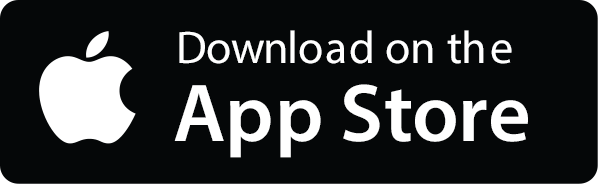
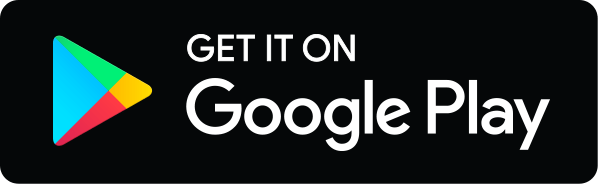