Introduction
Bleeding and thrombosis are common complications in the intensive care unit (ICU); their impact on neurologically ill patients is of particular concern because these complications may result in permanent neurologic disability. Sequelae from unrecognized bleeding can lead to cerebral ischemia, organ hypoperfusion, multiorgan failure, and death. Thrombotic complications, such as deep vein thrombosis (DVT), are common in neurologically injured patients given the associated limb weakness and complicated by the altered mental state. Multimodality monitoring in the neurologic ICU may preempt and thus mitigate the consequences of these common conditions.
The first part of this chapter briefly reviews the physiology of erythropoises and the consequences of anemia and thrombocytopenia, explores the complications of blood transfusion, and discusses alternatives to blood product administration in neurologically critically ill patients. The clinical utility and application of new monitoring techniques available to evaluate anemia and thrombocytopenia in the ICU are discussed. The latter portion of this chapter is devoted to the diagnosis, monitoring, and management of venous thromboembolism.
Hematology
Erythropoiesis and Blood Counts
Erythropoiesis is catalyzed by the hormone erythropoietin and leads to production of mature erythrocytes. Reticulocytes are immature erythrocytes that are released into the circulation. Within 1 day, reticulocytes transform into mature red blood cells that circulate for 100 to 120 days. Ultimately, senescent red blood cells (RBCs) are removed from circulation by macrophages in the spleen and other reticuloendothelial tissues. On average, the number of erythrocytes formed is equivalent to the number destroyed.
The complete blood count (CBC) provides accurate information about hemoglobin (Hgb) concentration and cell counts as well as calculated indices of erythrocyte size and Hgb content. The Hgb concentration varies by both age and gender ( Table 15-1 ). In the average adult male, the Hgb concentration varies between 13 and 17.5 g/dL; in women it ranges from 12 to 16 g/dL. A high white blood cell (WBC) count, lipemia, or a precipitating monoclonal protein can result in a spuriously high Hgb. High altitude will result in an increased Hgb concentration; the rise in Hgb is proportional to the magnitude of elevation. Women of childbearing age have an average Hgb 10% lower than men of the same age. Because of the wide range of normal Hgb and hematocrit (Hct) values, it often is difficult to diagnose mild anemia.
Age | Hemoglobin (g/dL) | Hematocrit (%) | Red Blood Cell Count (1012/L) | MCV (fL) | MCH (pg) | MCHC (g/dL) | ||||||
---|---|---|---|---|---|---|---|---|---|---|---|---|
Mean | −2 SD | Mean | −2 SD | Mean | −2 SD | Mean | −2 SD | Mean | −2 SD | Mean | −2 SD | |
Birth (cord blood) | 16.5 | 13.5 | 51 | 42 | 4.7 | 3.9 | 108 | 98 | 34 | 31 | 33 | 30 |
1-3 days (capillary) | 18.5 | 14.5 | 56 | 45 | 5.3 | 4.0 | 108 | 95 | 34 | 31 | 33 | 29 |
1 week | 17.5 | 13.5 | 54 | 42 | 5.1 | 3.9 | 107 | 88 | 34 | 28 | 33 | 28 |
2 weeks | 16.5 | 12.5 | 51 | 39 | 4.9 | 3.6 | 105 | 86 | 34 | 28 | 33 | 28 |
1 month | 14.0 | 10.0 | 43 | 31 | 4.2 | 3.0 | 104 | 85 | 34 | 28 | 33 | 29 |
2 months | 11.5 | 9.0 | 35 | 28 | 3.8 | 2.7 | 96 | 77 | 30 | 26 | 33 | 29 |
3-6 months | 11.5 | 9.5 | 35 | 29 | 3.8 | 3.1 | 91 | 74 | 30 | 25 | 33 | 30 |
0.5-2 years | 12.0 | 10.5 | 36 | 33 | 4.5 | 3.7 | 78 | 70 | 27 | 23 | 33 | 30 |
2-6 years | 12.5 | 11.5 | 37 | 34 | 4.6 | 3.9 | 81 | 75 | 27 | 24 | 34 | 31 |
6-12 years | 13.5 | 11.5 | 40 | 35 | 4.6 | 4.0 | 86 | 77 | 29 | 25 | 34 | 31 |
12-18 YEARS | ||||||||||||
Female | 14.0 | 12.0 | 41 | 36 | 4.6 | 4.1 | 90 | 78 | 30 | 25 | 34 | 31 |
Male | 14.5 | 13.0 | 43 | 37 | 4.9 | 4.5 | 88 | 78 | 30 | 25 | 34 | 31 |
18-49 YEARS | ||||||||||||
Female | 14.0 | 12.0 | 41 | 36 | 4.6 | 4.0 | 90 | 80 | 30 | 26 | 34 | 31 |
Male | 15.5 | 13.5 | 47 | 41 | 5.2 | 4.5 | 90 | 80 | 30 | 26 | 34 | 31 |
* These data have been compiled from several sources. Emphasis is given to studies employing electronic counters and to the selection of populations that are likely to exclude individuals with iron deficiency. The mean −2 SD can be expected to include 95% of the observations in a normal population.
The RBC count is the number of RBCs per unit volume. It generally ranges between 4.2 and 5.9 million cells per microliter. RBCs are the most common cell type present in blood. They are smaller than white blood cells but larger than platelets. A normal platelet count is between 150,000 and 400,000/µL. Thrombocytopenia is defined as a platelet count less than 150,000/µL.
The Hct is the ratio of RBCs to blood volume. With modern laboratory equipment, the Hct is calculated and not measured directly. The mean concentration of Hgb within the red cell population (mean corpuscular hemoglobin concentration [MCHC] is the quotient of the Hgb divided by the Hct. The red cell distribution width (RDW), another parameter of red cell size, measures the variability in red cell size of circulating erythrocytes. For example, the etiology of anemia with a low MCV and a high RDW is most likely iron deficiency; even though the anemia is microcytic, the variability of RBC size is high. In contrast, a macrocytic anemia would yield both a high MCV and RDW. Together with the reticulocyte count, the MCV and RDW can narrow the differential diagnosis of anemia.
The reticulocyte count (RC) measures the number of immature RBCs in circulation and is a marker of RBC production. This count may be inaccurate in the presence of nucleated RBCs or nuclear debris in the peripheral blood. When the RC is reported as a percentage, it should be adjusted for the total number of RBCs present. This correction can be made by multiplying the reticulocyte count by the Hct and divided by age and gender specific normative Hct values. Alternatively, the percent of reticulocytes can be multipied by the red cell count to determine the absolute RC. The normal absolute reticulocyte count (ARC) is between 25,000 and 75,000/µL. In the presence of anemia, an ARC less than 75,000/µL indicates a hypoproliferative process, whereas an ARC greater than 100,000/µL indicates hemolysis or an appropriate erythropoietin response. RCs between 75,000 and 100,000/µL must be interpreted for the degree of anemia present.
Review of the peripheral blood smear remains an informative diagnostic tool. Not only can it confirm findings of an automated CBC, but it also may suggest a marrow disease or hemolytic process. Microcytic, hypochromic cells may suggest iron deficiency or thalassemia, whereas macrocytosis with ovalocytes may suggest a megaloblastic anemia. The peripheral smear may demonstrate echinocytes, seen in uremia; acanthocytes, observed in liver disease; or schistocytes, found in disseminated intravascular coagulation (DIC). Special stains of the peripheral smear may assist in the diagnosis of anemia, particularly when there is rouleaux formation and automated counters may be inaccurate.
Early after acute hemorrhage, red cell mass and plasma volume contract at the same rate; therefore, there may not be any observed reduction in Hgb concentration or Hct. The platelet count often increases after acute blood loss and there often is an increase in reticulocyte count. Because young erythrocytes are larger than mature ones, the MCV is usually slightly elevated. Bleeding is usually accompanied by an increase in indirect bilirubin, which reflects the catabolism of heme from extravasated RBCs.
Anemia
Anemia in the Intensive Care Unit
Anemia is a common problem among critically ill patients. According to the World Health Organization, anemia is clinically defined as an Hgb concentration less than or equal to 12 g/dL for women and 13 g/dL for men. Nearly two thirds of patients are anemic on admission to the ICU and between 70% and 95% of patients become anemic by day 3. Anemia is potentially deleterious because lower Hgb levels decrease oxygen carrying capacity of the blood and may reduce tissue oxygenation. This is detrimental for patients with critical illness who often have increased metabolic demand for oxygen from infection, sepsis, fever, and mechanical ventilation and may be particularly detrimental for patients in the neurocritical care unit (NCCU) with reduced cerebrovascular reserve.
Anemia is associated with increased ICU length of stay and increased mortality in critically ill patients. In surgical ICU patients with cardiac disease, anemia is associated with increased risk of death. Anemia also is associated with the development of left ventricular hypertrophy in patients with end-stage renal disease, and its correction is associated with fewer cardiovascular events. Preoperative anemia is associated with worse surgical outcomes and preprocedural anemia among patients undergoing percutaneous coronary intervention is associated with increased adverse in-hospital outcomes.
Causes of Anemia in the Intensive Care Unit
The causes of anemia in the ICU are varied and may occur concurrently. These include sepsis, trauma, burns, infections, hemolysis, immune-associated iron deficiency, decreased endogenous erythropoietin production, renal failure, liver disease, malignancy, collagen vascular disease, nutritional deficiency, gastrointestinal bleeding, drug-related causes, and phlebotomy. Phlebotomy is the most significant modifiable factor associated with anemia in the ICU and is a predictor of blood transfusion ; it accounts for 40 to 70 mL per day of blood loss early in the ICU period. Smoller observed that the amount of blood drawn per day varied from 12 mL per day on the ward, to 33 mL a day in the ICU in patients without an arterial line, to 74 mL a day in ICU patients with an arterial line. For every 100 mL of blood removed by phlebotomy, Hgb and Hct levels fall by 0.7 g/dL and 1.9%, respectively.
ICU patients with evidence of inflammation and multiorgan failure develop anemia despite a lack of active bleeding. This “anemia of critical illness” results from underproduction of erythrocytes and is similar to anemia of chronic disease, which is thought to be due to inflammation. Inflammatory cytokines, such as tumor necrosis factor-α (TNFα), interferon-α (IFNα), and interleukin-1 (IL-1), suppress erythropoiesis and are central in the pathogenesis of anemia in these patients. These inflammatory mediators inhibit hypoxia-induced (as opposed to anemia-induced) erythropoietin (EPO) production by up to 89%.
Proinflammatory cytokines not only induce bone marrow suppression of erythropoiesis but also aggravate bleeding and disrupt iron metabolism. These cytokines exacerbate intestinal bleeding through increased permeability of the intestinal wall. They alter iron metabolism by stimulating phagocytosis of RBCs. More than 90% of ICU patients have low levels of serum iron (Fe), total iron binding capacity (TIBC), and Fe-to-TIBC ratio, but have normal or elevated serum ferritin. Despite low levels of circulating iron, EPO levels remain only modestly increased and are not markedly elevated. This blunted EPO response results from the inhibition of EPO transcription by inflammatory mediators. Rogiers et al. found that at the same serum level of Hgb, ambulatory anemic patients had an eight times higher level of EPO than did septic ICU patients.
The etiology of anemia in the ICU is multifactorial and can be difficult to determine. The diagnosis of anemia in the ICU is complicated because serial monitoring of hematologic profiles (i.e., phlebotomy) can exacerbate the condition.
Monitoring Anemia in the Intensive Care Unit
Modern laboratory testing and the availability of automated cell counters provide a wealth of diagnostic information for interpretation.
The extended differential count (EDC) quantifies other cell types in the peripheral blood including nucleated RBCs (NRBCs). Although NRBCs typically are only seen in neonates, their presence has been identified in adults, notably in patients with septicemia, massive hemorrhage, and severe hypoxia. When identified in adults, the presence and burden of NRBC may correlate with mortality and poor outcome. For example, Stachon observed a mortality rate of 44% in surgical patients with NRBCs compared with 4.2% for patients without.
Other parameters of cellular analysis available through automated analyzers include the immature reticulocyte fraction (IRF), mean reticulocyte hemoglobin content (CHr), mean reticulocyte volume (MCVr), fragmented RBC (FRBC) count, and the immature platelet fraction (IPF). IRF is an early and sensitive index of erythropoiesis. When characterized with total reticulocyte count, it can discriminate between anemias with increased erythropoiesis (increased total reticulocyte count and IRF) such as hemolytic anemias; decreased marrow function (decreased total reticulocyte count and IRF); and conditions such as acute infections and myelodysplastic syndrome with dissociation of total reticulocyte count and IRF (total reticulocyte count low, IRF high). It also is useful to identify resumption of marrow activity after bone marrow transplantation or chemotherapy.
The CHr and the MCVr are reticulocyte indices that reflect the adequacy of iron stores available for erythropoiesis. The CHr is important because it can help identify iron-deficiency anemia in the face of the acute phase response when total iron stores, as evidenced by an elevated ferritin and transferrin, are adequate. This may be seen in anemia of chronic disease. The MCVr has not been well studied, but has been used to evaluate possible EPO abuse in sports. The main limitations of these new indices include limited availability of the machines and variable sensitivity of the analyses.
FRBCs can be found in a variety of diseases from microangiopathies to cardiovascular disorders (valve prostheses and endocarditis). Microangiopathies need immediate diagnosis and treatment, and expedient identification and quantification is paramount. The sensitivity of the new analyzers to diagnose microangiopathy is very high (>90%), but the specificity is low (20%-50%). Therefore in certain populations, the FRBC count may be a good screening test for microangiopathies, but further study is warranted.
In addition to new laboratory indices of red cell size and function, new monitoring devices permit measurements of total Hgb concentration. In contrast to the standard cyanomethemoglobin method of Hgb determination, co-oximeters use spectrophotometry to determine Hgb concentration. The methodology of Hgb measurement is similar to that of oxyhemoglobin measurement by conventional pulse oximetry, except that instead of two wavelengths of light, multiple wavelengths are transmitted through the finger to measure the light absorbance characteristics of Hgb. Point-of-care co-oximeters, such as the HemoCue (Angelholm, Sweden), allow for capillary measurement of Hgb concentration at the bedside, whereas noninvasive co-oximeters, such as the Radical-7 (Masimo Corp, Irvine, CA) device, provide continuous Hgb measurements. The accuracy of these devices compare well against standard laboratory assays, although the Radical-7 may give lower readings than the HemoCue during surgery. Hgb measurements with these devices have the additional benefit of providing rapid results that may facilitate monitoring in the operating room, ICU, and emergency department.
Anemia in the Neurocritical Care Unit
Anemia is common in the NCCU and, depending on the definition, occurs in nearly 40% of patients. It has been identified as an independent factor associated with death or severe disability in several disorders including subarachnoid hemorrhage (SAH), particularly in patients who develop delayed cerebral ischemia, traumatic brain injury (TBI), or intracerebral hemorrhage (ICH). However, there remains variation in how anemia is managed.
In the uninjured brain, vasodilation of the cerebral arteries compensates for anemia-related reduction in oxygen carrying capacity; therefore, frank brain hypoxia does not typically occur at Hgb concentrations greater than 6 g/dL. The ability of cerebral vessels to vary their caliber to maintain constant perfusion is termed cerebral autoregulation . With impairment of cerebral autoregulation, such as may occur after SAH or TBI, tissue hypoxia may occur at higher Hgb concentrations. Consistent with this, fewer ischemic events are observed in SAH patients with higher Hgb concentrations. However, the Hgb threshold below which cerebral metabolic dysfunction occurs is only beginning to be elucidated.
A fundamental tenet of neurocritical care is that ischemia is an important cause of brain injury. Anemia and compromised oxygen delivery may exacerbate ischemia; therefore anemia may represent a therapeutic target in the NCCU. However, the ramifications of anemia may vary by the type of brain injury. The consequences of anemia after SAH, particularly among patients with severe vasospasm or delayed cerebral ischemia (DCI), may be dire. In contrast, the significance of cerebral ischemia in other neorocritically ill subpopulations is less clear; therefore, anemia may be of less concern.
Optimal Hemoglobin Threshold in the Neurocritical Care Unit Population
Several physiology studies in neurocritically ill patients have sought to define an Hgb threshold by measuring markers of cerebral metabolism. Brain tissue oxygen tension (P bt O 2 ) monitoring and lactate-to-pyruvate ratio (LPR) measurements from cerebral microdialysis demonstrated increased brain hypoxia and cell energy dysfunction when Hgb levels were less than 9 g/dL in SAH patients. However, the physiologic data in TBI are conflicting. Although TBI patients frequently have pathologic evidence of cerebral infarction, premortem evidence of ischemia is debatable. Early evidence demonstrated compromised cerebral blood flow suggestive of ischemia; however, it was later demonstrated that the altered blood flow correlated with decreased cerebral metabolism. Regardless, anemia appears to be a predictor of poor outcome, although transfusion of PRBCs does not always appear to mitigate its effect.
The treatment of anemia in the NCCU is unclear in part because of the heterogeneous pathophysiology of diseases treated therein. Moreover, transfusion trials in general ICUs included very few or excluded neurologically critically ill patients and therefore results cannot be easily extrapolated from them. Transfusion studies in patients with active cardiac ischemia have not demonstrated consistent benefit from a liberal transfusion approach. It is unclear whether this is analogous to patients with cerebral ischemia.
Thrombocytopenia
Thrombocytopenia in the Critically Ill
The importance of platelets and their role in thrombus formation is clear, but their significance in sepsis is being elucidated. Thrombocytopenia occurs in 13% to 58% of ICU patients ; 23% have at least one platelet count less than 100,000/mm 3 and 10% had counts less than 50,000 mm 3 . Sepsis is considered the major independent risk factor for thrombocytopenia in the ICU. Other factors associated with thrombocytopenia are episodes of bleeding or transfusion, longer ICU stays, and Acute Physiology and Chronic Health Evaluation II (APACHE II) scores greater than 15. The development of thrombocytopenia during the ICU stay is associated with decreased ICU survival and may have a greater implication for prognosis than admission thrombocytopenia. For example, Cawley et al. observed that a drop in platelet count to less than 50% of admission levels was associated with greater mortality than admission APACHE II, Simplified Acute Physiology Score (SAPS), or multiple organ dysfunction syndrome (MODS) scores.
ICU-related thrombocytopenia is primarily caused by peripheral loss, destruction, and margination of platelets rather than bone marrow hypoplasia or nutritional deficiency. Sepsis accounts for 48% of cases. Other causes include liver disease, hypersplenism, consumption, platelet destruction, DIC, medications, immune phenomena, and intravascular devices. The cause of a low platelet count in the ICU can be difficult to determine and in more than 25% of critically ill patients the cause is multifactorial. Pseudothrombocytopenia occurs when platelets clump in association with an ethylenediamine tetra-acetic acid (EDTA)–dependent platelet agglutinin. An accurate platelet count can be determined by collecting the sample in a citrated collection tube or by using a heparinized blood sample. Postresuscitative hemodilution can result in thrombocytopenia (i.e., after blood transfusion or with crystalloid or colloid replacement therapy for blood loss). The post-transfusion reduction in platelet count is associated with both splenic sequestration of platelets and a reduction in viable platelets in stored blood.
Platelets play a complex role in sepsis and multiorgan dysfunction. Platelets of septic patients may display increased adhesion and aggregation. Bacteria and bacterial products may affect platelet function. Lipopolysaccharide increases platelet aggregation in some animal models, but has the opposite effect on human platelets in vitro. Lipoteichoic acid, a cellular membrane component of gram-positive bacteria, inhibits platelet aggregation in human platelets. Escherichia coli endotoxin may indirectly reduce platelet responsiveness.
Drug-Induced Thrombocytopenia
Drug-induced thrombocytopenia (DIT) is a common nonimmune-mediated disorder, associated with bone marrow suppression and accelerated platelet destruction and defined as a platelet count between 50 and 150,000 × 10 6 /L with any degree of spontaneous bleeding. It is observed in 19% to 25% of ICU patients and associated with medications such as cytotoxic chemotherapeutic agents, thiazide diuretics, ethanol, tolbutamide, and bleomycin. DIT typically develops 2 to 3 days after a previously administered medication, and 7 days after a virgin drug. Discontinuation of the medication normalizes the platelet count by 5 to 8 days. A major bleeding event can occur in 4% of patients, and these patients may benefit from intravenous immunoglobulin (IVIg), plasmapheresis, or platelet transfusion but not corticosteroids.
Drug-Induced Immune Thrombocytopenia
Drug-induced immune thrombocytopenia (DITP) is a disorder characterized by the development of drug-dependent antibodies that bind to glycoproteins on the surface of platelets and accelerate their destruction in the presence of the specific drug. This type of disorder may be more severe than DIT. Hundreds of drugs have been associated with this type of thrombocytopenia; the most common are heparin, quinine (cinchona alkaloids), penicillin, sulfonamides, nonsteroidal anti-inflammatory drugs (NSAIDs), anticonvulsants, antirheumatics, oral hypoglycemics, gold salts, diuretics, rifampicin, ranitidine, and the glycoprotein (GP) IIb/IIIa inhibitors (e.g., abciximab, tirofiban, or eptifibatide). The precise mechanisms by which various drugs incite the immune response are beyond the scope of this chapter. Some patients can make both drug-independent and drug-dependent antibodies during the course of medication exposure. The drug-independent antibodies (autoantibodies) usually are transient but if they persist, can result in a chronic autoimmune thrombocytopenic purpura. When agents such as GPIIb/IIIa inhibitors are used, platelet counts should be frequently monitored. If the platelet count is less than 50,000/µL, the drug should be discontinued. If the platelet count is less than 10,000/µL and there is severe bleeding or if an invasive procedure is required, then platelet transfusion should be considered. Readministration of GPIIb/IIIa at a later date is not recommended because the rechallenge might prove more severe than the initial event.
Heparin-Induced Thrombocytopenia
Heparin-induced thrombocytopenia (HIT) is an anticoagulant-induced prothrombotic immune disorder caused by heparin-dependent platelet-activating IgG antibodies that recognize complexes of platelet factor 4 (PF4) bound to heparin. HIT should be suspected when the platelet count falls to less than 50% of the baseline level or an absolute number of less than 150,000/µL between days 5 and 14 of heparin exposure. Several factors are associated with the development of HIT: (1) the dose or duration of heparin use; (2) the type of heparin administered; (3) the patient population; and (4) patient gender ( Table 15.2 ). A greater risk of HIT is observed in patients exposed to a high dose of heparin or a long duration of treatment, with unfractionated heparin rather than low molecular weight heparin (LMWH), with surgical rather than medical patients, and in females.
Risk Factor | Odds Ratio | Comment |
---|---|---|
Duration of heparin use (>1 week vs. <1 day) | ∼20-100 | Odds ratio (OR) estimated as follows: risk for HIT postcardiac surgery (with UFH prophylaxis for >1 week) ∼2% versus risk for “delayed-onset HIT” (without prophylaxis) ∼0.02%-0.1% |
Type of Heparin UFH > LMWH > fondaparinux | ∼10-15 | Difference in risk for HIT between heparin types is established for postsurgical thromboprophylaxis (UFH vs. LMWH) and is more pronounced in women. ORs shown are for UFH versus LMWH (woman, postsurgical prophylaxis) [18] |
Type of patient (surgery > medical > pregnancy) | ∼3-4 | Highest reported frequencies of HIT are in postsurgical thromboprophylaxis (OR shown is for surgery vs. medical) [18] |
Gender (female > male) | ∼1.5-2.0 | Difference in risk for HIT between women and men has only been established for UFH thromboprophylaxis |
PF4 is a tetrameric protein, involved with hemostasis, immunoregulation, and angiogenesis. Heparin binding induces a conformational change in PF4. HIT differs from most other drug-induced immune thrombocytopenias in that the induced antibodies engage the Fc receptor (not Fab region) and thereby activate platelets (i.e., they promote thrombosis and a hypercoagulable state, which is characteristic of HIT). PF4 also neutralizes the anticoagulant effect of heparin, which exacerbates the prothrombotic milieu.
Clinical complications of HIT include both venous and arterial thromboses. Arterial thrombosis is more common in those with known cardiac disease, whereas DVT is seen in postoperative patients. Cerebral sinus thrombosis, venous limb gangrene, and hemorrhagic adrenal infarction also are reported. Concomitant prothrombotic risk factors include diabetes mellitus, malignancy, systemic lupus erythematosus (SLE), antiphospholipid antibody syndrome, indwelling catheters, and trauma. The incidence of stroke is 3.1% among patients with HIT and is more common in females, in patients with more severe thrombocytopenia, and early in the course of HIT. Myocardial infarction can occur in 3% to 5% of patients and amputation in as many as 20% of patients with HIT and limb thrombosis. In SAH, HIT may occur in up to 15% of patients, and its risk is increased in Fisher grade 3 patients, females, and perhaps endovascular treatments. The incidence of hypodensity on computed tomography (CT) scans and worse outcome is increased.
PF4 antibodies are transient and are undetectable a median of 50 to 85 days after an episode of HIT. In some patients antibodies remain detectable at low levels for several months. If heparin is readministered to a patient with high levels of antibodies, development of thrombocytopenia can be rapid. This is unlikely more than 100 days from exposure. The “4 T’s”—Thrombocytopenia, Timing, Thrombosis, and oTher plausible explanations for the clinical scenario—is an eight-point clinical scoring system that evaluates the likelihood of developing HIT ( Table 15.3 ). It has a high negative predictive value and can help rule out HIT. If HIT is suspected all heparin sources must be stopped, preferably before the demonstration of HIT antibodies. The direct thrombin inhibitor family of anticoagulants should replace heparin because treatment with LMWH does not fully eradicate the risk of HIT.
Date : | Points (0, 1, or 2 for each of 4 categories: maximum possible score = 8) | ||
---|---|---|---|
2 | 1 | 0 | |
T hrombocytopenia * Score = _____________ | >50% platelet decrease to nadir ≥20 × 109/L | 30%-50% platelet count decrease (or >50% directly resulting from surgery) or nadir 10 − 19 × 109/L | <30% platelet decrease or nadir <10 × 10 9 /L |
T iming † of platelet count decrease, thrombosis, or other sequelae (first day of heparin course = day 0) Score = ________ | Days 5-10 onset † or ≤1 day (with recent heparin exposure within past 5-30 days) | Consistent with days 5-10 decrease, but not clear (e.g. , missing platelet counts), or ≤1 day (heparin exposure within past 31-100 days), or platelet decrease after day 10 | Platelet count decrease ≥4 days without recent heparin exposure |
T hrombosis (including adrenal infarction) or other sequelae (e.g., skin lesions) Score = ____________ | Proven new thrombosis, or skin necrois (at injection site), or post-IV heparin bolus anaphylactoid reaction | Progressive or recurrent thrombosis or erythematous skin lesions (at injection sites), or suspected thrombosis (not proven) | None |
O T her cause for thrombocytopenia Score = ____________ | No explantion for platelet count decrease is evident | Possible other cause is evident | Definite other cause is present |
Total score = _______________ Pretest probability score: 6-8 = high, 4-5 = intermediate, 0-3 = low |
* Changes to score can occur, based on new information (e.g., further decrease in platelets, new thrombosis, other causes for platlet decrease).
† First day of immunizing heparin exposure considered day 0; the day the platelet count begins to decrease is considered the day of onset of thrombocytopenia (it generally takes 1 to 3 more days until an arbitrary threshold that defines thrombocytopenia is passed. Usually, heparin administered at or near surgery is the most immunizing situation).
Thrombotic Microangiopathies: Thrombotic Thrombocytopenic Purpura
Thrombotic thrombocytopenic purpura (TTP) is a rare but potentially fatal disorder that mostly affects adult women. It is characterized by red cell fragmentation (microangiopathy), hemolytic anemia, and thrombosis (consumptive thrombocytopenia) that lead to ischemic organ damage. The predilection for thrombosis is a consequence of abnormal numbers of von Willebrand multimers (µL-vWF) in the circulation due to altered vWF homeostasis. vWF is normally released in response to prothrombotic stimuli, and persistence of these multimers leads to platelet activation and clumping with subsequent microvascular thrombosis. The protease, ADAMTS13, a disintegrin and metalloproteinase with a thrombospondin type 1 motif, member 13 normally cleaves the µL-vWF into smaller peptides. When the protease activity is decreased or absent there is accumulation of the large multimers. The activity of ADAMTS13 can be decreased by many different factors but most cases of acquired adult TTP result from autoantibodies to ADAMTS13. Congenital TTP results from complete absence of this protease.
TTP often is seen with infection, pregnancy, or estrogen-containing compounds. General clinical features include fever, anemia, thrombocytopenia, renal failure, and neurologic symptoms from multiorgan damage due to microvascular thrombi. Patients present with complaints of weakness, malaise, nausea, vomiting, abdominal pain, hallucinations, and seizures. Diagnostic laboratory tests for TTP include hemoglobin, platelet count, peripheral smear (to document fragmentation), lactate dehydrogenase (LDH), bilirubin, and reticulocyte count. ADAMTS13 activity level and antibody tests may be helpful. The differential diagnosis for TTP includes DIC, the HELLP syndrome (hemolytic anemia, elevated liver enzymes, low platelets), hemolytic uremic syndrome (HUS), malignant hypertension, preeclampsia, and some infections. Treatment is aimed at prompt removal of circulating antibodies (by plasma exchange), replacement of ADAMTS13 (by administration of FFP, cryoprecipitate) and immunosuppression (with steroids, cyclosporine, vincristine, cyclophosphamide, or rituximab).
Disseminated Intravascular Coagulation
Disseminated intravascular coagulation (DIC) is a syndrome of thrombosis and systemic hemorrhage caused by an abnormally activated extrinsic clotting cascade or tissue factor system. Tissue factor (TF) is a tissue membrane glycoprotein, which binds to factor VII. Normally, the TF/factor VIIa complex activates factors X and XI that initiate clotting. In the face of systemic inflammation, circulating cytokines, IL-1, IL-6, and TNFα cause the constitutive release of tissue factor and down-regulate thrombomodulin, an endothelial cell surface receptor that activates anticoagulants and inhibits factors Va and VIIa. The down-regulation of thrombomodulin converts the antithrombotic blood vessel endothelial surface to a prothrombotic one. The early thrombotic phase causes widespread fibrin deposition, which is followed by consumption of platelets and clotting factors that culminate in fibrinolysis and hemorrhage.
The diagnosis of DIC should always be suspected if the patient has acrocyanosis, purpura, dusky cyanosis of the nose, ears, and genitalia, or is oozing blood from venipuncture or arterial catheter sites. Neurologic complications of DIC include coma, encephalopathy, SAH, small and large vessel stroke, embolic infarction from nonbacterial thrombotic emboli, and intracerebral hemorrhage. DIC is a major cause of stroke in medical ICUs, is a frequent complication in terminally ill patients, and may aggravate neurologic deficit after TBI. Several scoring systems help diagnose DIC. One such system developed by the International Society of Thrombosis and Haemostasis combines platelet count, the ratio of fibrin monomers to fibrin degradation products, a prolonged prothrombin time (PT), and fibrinogen level to determine a DIC score. The sensitivity and specificity of this scoring system is greater than 90%. Other scoring systems includes a D-dimer test and can help predict mortality.
Monitors of Platelet Function
Circulating platelets vary in size, metabolism, and activity. Newly released platelets contain RNA and owing to the similarity to reticulocytes, are called reticulated platelets . The largest are more reactive and secrete a greater quantity of thrombogenic factors. Automated counters can quantify reticulated platelets and determine platelet size variability and mean platelet volume. Fluorescent dyes and cytometers have allowed quantification of reticulated platelets. Increased presence of reticulated platelets may be seen in peripheral platelet destruction and acute blood loss, whereas a decreased number may be seen in marrow hypoplasia or cytotoxic chemotherapy. The increase in immature platelet fraction (IPF) may precede the rise in platelet count by days, and may serve to limit prophylactic platelet transfusion after chemotherapy. Moreover, an increase in reticulated platelets may be associated with an increased risk of thrombosis in thrombocytosis and myeloproliferative disorders.
New automated counters can provide the mean platelet volume (MPV) and platelet distribution width (PDW), which are analogous to the erythrocyte indices. There is a nonlinear inverse relationship between MPV and platelet concentration; MPV decreases with higher platelet counts. Therefore the MPV should be assessed relative to platelet number. MPV is also a known marker of platelet activation. MPV elevation is associated with increased risk of myocardial infarction (MI) in patients with coronary artery disease (CAD) and increased mortality after acute MI. Studies have demonstrated MPV elevations in acute ischemic stroke ; moreover, some studies suggest that increased MPV is related to poor functional outcome, although this remains controversial. Although these parameters provide novel information, there are many limitations to the use of platelet indices. The anticoagulant used for CBC determination incites nonuniform structural changes in platelets. As a consequence, the MPV may increase or decrease depending on the method of measurement. There is no mathematical correction for this phenomenon, and therefore platelet indices are still considered experimental.
Platelet Function Assays
Platelet aggregation with a platelet aggregometer is the gold standard to test platelet function. However, this method is labor intensive and requires technologic expertise; therefore, it is unsuitable for bedside monitoring. Since 2000, devices that can be used in the laboratory or at the point of care (POC) have become available; however, the results do not always correlate with the gold standard, aggregometry, and more studies need to define the role of these new assays.
A variety of POC testing (POCT) devices are available for platelet function. These devices have been developed primarily to measure the effect of antiplatelet medications and not necessarily to diagnose platelet function defects. The Platelet Function Analyzer 100 (PFA-100, Siemans Corporation, NY) is an optical detection device that measures closure time, or time to the cessation of the blood flow through a channel coated with collagen and a platelet activator, either adenosine diphosphate (ADP) or epinephrine. Eight hundred microliters of citrated whole blood is required for the analysis. Although PFA-100 results are abnormal in both congenital platelet abnormalities and moderate-severe von Willebrand’s disease (vWD), it is not sensitive enough to serve as an adequate screening test to exclude all congenital abnormalities or mild vWD. A normal ADP closure time has been found to be a good negative predictor of bleeding ; however, an elevated closure time has a poor positive predictive value. Other POCT is intended primarily to monitor antiplatelet medications such as aspirin, clopidogrel, or glycoprotein IIb/IIIa inhibitors. Correlation with platelet aggregometry has been poor; one study found the sensitivity of the PFA-100 and the Verify-Now (Accumetrics, San Diego, CA) to be 62% and 39%, respectively. Another study of 100 patients treated with 75 to 100 mg of aspirin for CAD demonstrated variable levels of aspirin resistance (12%-22%) when measured by four tests of platelet aggregability. Only 2% of patients were aspirin resistant by all tests, and correlation between tests was poor.
Transfusion in the Critically Ill
Packed Red Blood Cell Transfusion
Blood transfusion in the ICU is common due in large part to the increased severity of illness, the burden of chronic diseases, improved ICU management, and blood-intensive surgeries. Annually, approximately 15 millions units of blood are donated, and 13 million are transfused. Up to 85% of patients remaining in the ICU more than 1 week are transfused, with an average total transfusion of 9.5 units. Overall packed red blood cell (PRBC) transfusion rate among ICU patients is between 37% and 50%.
Since 2000, concern about the safety of PRBC transfusion, including immunosuppressive and microcirculatory complications, has led to a reappraisal of transfusion practices. In 1999, the Transfusion Requirements in Critical Care (TRICC) trial, a randomized study, examined whether a restrictive (Hgb threshold of 7 g/dL) and liberal (Hgb threshold of 10 g/dL) transfusion strategy in the ICU was associated with mortality. The primary endpoint of 30-day mortality was similar between two groups and in subgroups, including in patients with cardiac disease, severe infections or septic shock, or trauma. However, in patients with an APACHE II score less than 20, or those younger than 55 years of age, a restrictive approach was associated with less mortality. The authors recommended that critically ill patients with isovolemic anemia receive PRBC transfusion when the Hgb concentration is less than 7 g/dL and that Hgb levels be maintained between 7 and 9 g/dL. Many observational studies are consistent with this observation. The most appropriate transfusion strategies remain unclear for patients in the NCCU; although guidelines regarding transfusion practice have been published for general critical care, neurocritical care, TBI, and SAH.
Efficacy of Transfusion
RBC transfusions are intended to augment oxygen delivery and improve tissue oxygenation. It is presumed that increased Hgb concentration increases oxygen carrying capacity and provides more oxygen to delivery-dependent tissue. However, whether blood transfusion successfully achieves improved tissue oxygenation remains unclear. In the NCCU population several studies have examined the effect of transfusion on brain oxygen. In summary, transfusion increases brain oxygen in about 75% of patients. However, even when transfusion increases brain oxygen it may not always correct other markers of cellular distress (e.g., the LPR).
Sequelae of blood storage, termed the storage lesion, may be responsible for the observed lack of efficacy of RBC transfusion (RBCT). First, during storage, RBCs undergo a predictable change from biconcave disks to deformed spheroechinocytes, resulting in loss of deformability and decreased ability to navigate the microcirculation (vessels of 3-8 µm). Second, increased osmotic fragility may lead to decreased RBC survival. Third, adenosine triphosphate (ATP) is depleted. Fourth, the p50, a measure of Hgb affinity for oxygen, is lower in stored blood, which results in less oxygen unloading to tissues. Fifth, after 7 to 14 days, stored blood is depleted of 2,3-diphosphoglycerate (2,3-DPG) ; this shifts the oxygen dissociation curve to the left and reduces the amount of oxygen available for tissue consumption. Sixth, prolonged storage results in adhesion of RBCs to endothelial cells, which can compromise microvascular circulation. Finally, storage for more than 42 days may cause vasoconstriction upon transfusion due to free Hgb and lysophosphatidyl choline species released from the cellular membrane of senescent RBCs.
Potential Side Effects of Transfusion
A variety of deleterious side effects are associated with PRBC transfusion. First, although rare, risks are associated with crossmatching and the transmission of viral and prion diseases. The risk of transmission of human immunodeficiency virus (HIV), hepatitis C virus, and hepatitis B virus are 1 in 1,900,000, 1 in 600,000, and 1 in 220,000 respectively. Cytomegalovirus (CMV) is present in 4% of transfusions from healthy donors due to the reactivation of latent CMV in leukocytes. Other transfusion-transmitted infections are thought to be due to contaminated leukocytes. Contaminated leukocytes may also contribute to infection or febrile nonhemolytic transfusion reactions (FNHTR) and hemolytic transfusion reactions. In addition, leukocyte activity on RBC membranes during storage and degradation during storage that releases oxygen free radicals and proteases, may incite inflammation in the transfusion recipient.
Transfusion may have detrimental effects on the immune system. Transfusion-related immunomodulation may lead to alloimmunization, a heightened immune response as in transfusion reactions, or tolerance induction, a suppression of the immune response that predisposes to hospital-acquired infections. Alloimmunization and consequent induction of human leukocyte antigen (HLA) antibodies and T cell activation, results in several clinical syndromes, including transfusion reactions, transfusion-associated graft-versus-host disease, transfusion-related acute lung injury (TRALI), and perhaps various autoimmune diseases. TRALI is defined as a new episode of respiratory distress not explained by an alternate etiology that occurs during, or within 6 hours of, a completed transfusion. The most common clinical features include bilateral pulmonary edema, hypoxemia, fever, dyspnea, and hypotension in the presence of normal cardiac function. Plasma-rich blood components (FFP and platelets) and high-volume transfusion may predispose to TRALI, although it has been associated with all blood product components including IVIg and cryoprecipitate.
Tolerance induction after transfusion is associated with a decrease in natural killer cell function, defective antigen presentation, and a reduction in helper/suppressor T-lymphocyte ratio. Consequently transfusion is linked to increased predisposition to hospital-acquired and postoperative infections in variety of disorders and ICU patients, multiorgan dysfunction, acute respiratory distress syndrome (ARDS), systemic inflammatory response system (SIRS), and even to cancer recurrence. A recent meta-analysis of transfusion in the ICU suggests that the pooled odds ratio for developing an infectious complication was 1.8 (95% CI, 1.5-2.2).
The deleterious effects of transfusion are thought to occur in large part from inflammatory cytokines (e.g., IL-1, IL-6, IL-8), inflammatory mediators (e.g., bactericidal permeability-increasing protein [BPI] and TNFα) ; neutrophil activation and the accumulation of histamine, lipids, cytokines, cellular membranes, and HLA class antigens. Some of these compounds are derived from WBCs, which are present in the cellular components of standard-preparation blood products. Consequently although it seems reasonable to expect that leukoreduction of stored blood would mitigate the immunomodulatory effects of transfused RBCs, its clinical impact and cost effectiveness have not been fully elucidated. There also may be non–leukocyte mediated mechanisms of immunosuppression (i.e., RBC lysis leads to release of arginase, an enzyme that degrades arginine, an amino acid that normally stimulates lymphatic function).
Strategies to Prevent Anemia
Anemia can be detrimental to ICU patients, but so is its correction with blood transfusion. One way to reduce the incidence of anemia in the ICU is to decrease the amount of phlebotomized blood. Strategies to decrease phlebotomy include the use of pediatric collection tubes in adults, recycling of discard volumes, POC technology, and new paradigms in laboratory-ordering practices. The use of pediatric-size laboratory tubes can reduce blood loss by almost 50%. Discard volumes required for “clearing the line” amount to almost a third of daily blood loss in the ICU. Recycling of discard volumes through the use of blood conservatory devices can minimize iatrogenic blood loss. POC technologies employ rapid bedside analyzers of whole blood that provide accurate analyses of multiple analytes with minimal blood sampling. One milliliter of whole blood is sufficient for bedside analysis of pH measurements, arterial blood gases, selected electrolytes, glucose, and hematocrit. Use of another POC device, namely thromboelastography, has been shown to reduce not only administration of blood components but also bleeding. Education of physicians and nurses to promote judicious laboratory ordering and testing is paramount. Documentation of phlebotomy volume on ICU flow sheets has been shown to effectively decrease iatrogenic blood loss. Discontinuation of laboratory panels and standing laboratory orders may further reduce unnecessary blood draws. Batching of blood draws can also minimize the wastage associated with each phlebotomy attempt.
Alternatives to Transfusion
Because RBCT can worsen outcome, alternative strategies have been tried, including stimulation of red cell production by recombinant human EPO (rhuEPO), Hgb-based oxygen carriers, and perfluorocarbons. A detailed discussion of Hgb-based oxygen carriers and perfluorocarbons is beyond the scope of this chapter but is available elsewhere. The use of EPO is of particular interest to the NCCU patient population because both EPO and its receptor (EPOR) are present in neural and vascular tissue. Furthermore, several lines of evidence suggest that EPO may be neuroprotective. Whether rhuEPO is useful in the ICU remains unclear, because some studies suggest that although transfusion needs may be reduced there is a significant increase in the incidence of thrombotic vascular events. In addition, a variety of side effects including seizures, hypertension, headache, tachycardia, nausea, and clotted vascular access are described. Enthusiasm for the use of rhuEPO therefore has waned and many studies of erythropoiesis-stimulating agents (ESAs) have been halted. In 2008, the U.S. Food and Drug Administration (FDA) published a black box warning on all ESAs for increased risk of mortality, cardiovascular and thrombotic events, and tumor progression or recurrence. Because of its neuroprotective effects, EPO studies in ischemic stroke, SAH, and TBI continue (clinicaltrials.gov/sho/NCT00313716 and www.controlled-trials.com/mrct/trial/661629/bellomo ). There are promising results from preliminary human randomized controlled trials (RCTs) in SAH. However, mortality was actually greater with EPO in a recent multicenter RCT of acute ischemic stroke patients.
Coagulation
Hemostasis
The coagulation system is normally quiescent. Endothelial cells that line the intimal surface of blood vessels promote smooth blood flow by inhibiting platelet function and fibrin accumulation. Upon vascular injury, the antithrombotic properties of the endothelial monolayer are lost, and prothrombotic substances contained within the subendothelium are exposed, inciting platelet adhesion. This is mediated by vWF, which binds platelets at its receptor in the platelet membrane and attaches to the damaged vessel wall. Adherent platelets release the contents of the storage granules, including ADP and thromboxane A2, thus activating circulating platelets to bind the damaged vessel wall. These activated platelets expose binding sites for fibrinogen and lead to fibrin deposition, the final step in the formation of the hemostatic plug. Fibrin is converted from plasma fibrinogen by thrombin. Thrombin is formed from prothrombin, its zymogen precursor, and activated factors X and V (the common pathway). Factor X is most often activated by the tissue factor (extrinsic) pathway, but may be activated by the contact activation (intrinsic) pathway. Fibrin is ultimately digested by the fibrinolytic system, which is mediated by plasmin. Plasmin is formed from endothelial-derived plasminogen activators.
Four bleeding tests have traditionally been used to evaluate the bleeding patient: the PT, activated partial thromboplastin time (aPTT), bleeding time, and platelet count. The PT measures the integrity of the extrinsic and common pathways, including factors VII, X, and V; prothrombin; and fibrinogen; whereas the aPTT measures the integrity of the intrinsic and common pathways, including high molecular weight kallikrein (HMWK); prekallikrein; factors XII, XI, IX, VIII, X, and V; prothrombin; and fibrinogen. Bleeding time is a screening test of platelet-endothelial interactions. It measures the time to cessation of bleeding after a standard incision in the volar aspect of the forearm. It is prolonged in thrombocytopenia, vWD, platelet abnormalities, and primary vascular disorders; it is usually not affected by coagulation factor deficiencies. This test, however, lacks reproducibility and has poor sensitivity and specificity.
Normal results of these screening tests essentially exclude clinically significant bleeding. Notable exceptions include factor XIII deficiency and vWD, which may have normal results to these screening tests. If the bleeding time is prolonged and thrombocytopenia is not present, evaluation for medications (e.g., aspirin) or diseases (e.g., end-stage renal disease [ESRD]) that may affect platelet function is warranted. Prolongation of the PT or partial thromboplastin time (PTT) usually indicates a factor deficiency or presence of an inhibitor. A mixing study can discriminate between these etioloiges because a deficiency should correct with addition of normal plasma, whereas the presence of an inhibitor would not correct the prolonged time.
Venous Thromboembolism
Venous Thromboembolism in the Intensive Care Unit
Venous thromboembolism (VTE) is composed of DVT and pulmonary embolism (PE) and occurs in 117 to 145 per 100,000 individuals each year. In the United States, the frequency of DVT approaches 2 million people per year, with fatal PE in 50,000 to 200,000 patients. The 1-year mortality rate is as high as 21% for DVT and 39% for PE, and PE accounts for 5% to 10% of potentially preventable hospital deaths. Risk factors for VTE include: (1) age greater than 40 years; (2) prolonged immobility; (3) prior VTE; (4) malignancy; (5) myeloproliferative disorders; (6) cardiac dysfunction; (7) stroke; (8) respiratory failure; (9) pregnancy; (10) obesity; (11) varicose veins; (12) estrogen use; (13) inflammatory bowel disease; (14) nephritic syndrome; (15) femoral vein catheterization; (16) sepsis; (17) major surgery; (18) trauma; and (19) inherited or acquired thrombophilias. Among hospitalized patients the risk is much greater in ICU than other hospitalized patients ; in particular, patients who remain in the ICU more than 72 hours and who have end-stage renal failure, personal or family history of VTE, platelet transfusion, and vasopressor therapy are at increased risk. Despite routine thromboprophylaxis, 10% of ICU patients develop lower extremity DVT, and VTE remains one of the most common unsuspected autopsy findings in critically ill patients.
Venous Thromboembolism in Neurosurgical Patients
Neurosurgical patients have several unique risks for VTE including: (1) treatment-related immobilization, (2) disease-related or postoperative paralysis, (3) prolonged surgical procedures (>4 hours), (4) delayed initiation of activity, (5) corticosteroid use, and (6) pharmacologic dehydration. In particular patients with TBI, malignant tumors, advanced age and those who undergo craniotomy (in contrast to spinal surgery) are at greater risk. Kaolin-activated thromboelastography has identified a transient hypercoagulable state during and after neurosurgical operations; indices of hypercoagulability were more pronounced with longer operative times and cranial rather than spinal procedures. Brain tissue is rich in tissue factor (factor VII), which may incite a prothrombotic state. After surgical procedures there is increased inhibition of tissue plasminogen activator (tPA), a potent clot-dissolving enzyme that converts plasminogen to plasmin. Diminished tPA results in a reduced capacity to clear thrombi. Local hypoxemia in venous channels that may occur with lengthy neurosurgical procedures or with prolonged inactivity can promote clot formation in as little as 2 hours.
Among neurosurgical patients after craniotomy, the reported rates of VTE vary widely from zero to 60%. Although the prevalence of PE is between 1.5% and 8.4% among neurosurgical patients, the risk of mortality from PEs can be as high as 50% in this population. The greatest risk for DVT is in patients with brain tumors (28%-43%), followed by those undergoing craniotomy (25%), and those with TBI (20%).
The overall risk of developing DVT in patients with brain tumors is 3% to 60%. The incidence of DVT is generally considered highest with malignant gliomas, although some meningiomas, especially those that adhere to the sinuses, can be associated with higher DVT rates. The pathogenesis of VTE in brain tumor patients is thought to be associated with the high concentration of TF, the cell surface receptor of factor VII/VIIa, which plays a pivotal role in the initiation of the coagulation cascade. High-grade tumors may express higher concentrations of TF, procoagulants, and fibrinolytic inhibitors than low-grade tumors. Elevated levels of D-dimer, homocysteine, lipoprotein (a) (lp[a]), vascular endothelial growth factor (VEGF), tPA and plasminogen activator inhibitor (PAI) are found in patients with malignant glioma and likely contribute to the observed hypercoagulable state. Chemotherapeutic agents used to treat malignancy, including nitrosurea and cisplatin, may also increase the risk of DVT in these patients.
Thromboprophylaxis in Neurosurgical Patients
There is a general reluctance to use pharmacologic prophylaxis for VTE in neurosurgical patients because the consequences of bleeding may be devastating. Several studies have attempted to determine whether the risk of pharmacoprophylaxis outweighs the risk of VTE. Danish et al. sought to determine the best method of thromboprophylaxis in neurosurgical patients by use of a decision analysis model that incorporated the incidence, prevalence, and likelihood ratios of VTE and ICH. The addition of heparin lowered the incidence of VTE but increased the incidence of ICH. The impact of intracranial bleeding was greater than the benefits of heparinization, so they concluded that overall outcomes were best with mechanical prophylaxis alone. In a single center study the combination of low-dose unfractionated heparin and twice-weekly surveillance Doppler ultrasound in neurosurgical patients was associated with an incidence of PE of 0.09% and of DVT of 2.6%, a rate of VTE lower than that in the other ICUs within the same institution. In a randomized trial of 307 patients undergoing elective neurosurgical procedures, 17% of patients with compression stocking therapy and enoxaparin developed DVT, whereas 32% who received only compression devices had DVTs. The incidence of major bleeding was similar. The ninth American College of Chest Physicians (ACCP) conference on antithrombotic and thrombolytic therapy recommends mechanical prophylaxis, preferably with intermittent pneumatic compression (IPC) or pharmacolagic prophylaxis be used over no prophylaxis for patients undergoing craniotomy. The risk of a DVT in patients who undergo elective spinal surgery is low. The 9th edition ACCP guidelines recommend mechanical prophylaxis, preferably with IPC, over no prophylaxis (Grade 2c), unfractionated heparin (Grade 2c), or LMWH (Grade 2c) unless they have additional risk factors such as advanced age, known malignancy, presence of a neurologic deficit, previous VTE, or an anterior surgical approach. There also is debate on what to do after TBI or spinal cord injury (SCI). The 9th edition ACCP guidelines suggest that in the absence of a contraindication, all TBI patients receive LDUH (Grade 2c), LMWH (Grade 2c) or mechanical prophylaxis, preferably with IPC (Grade 2c). If LMWH is contraindicated, then IPC is recommended. Inferior vena cava (IVC) filters are not recommended as primary prophylaxis in trauma patients. In patients with acute SCI, which carries the highest risk of VTE, the ACCP guidelines recommend VTE prophylaxis with the use of IPC and either low-dose unfractionated heparin (LDUH) or LWMH, once primary hemostasis has been achieved.
Venous Thromboembolism Prophylaxis in Stroke Patients
VTE is a common complication of stroke, similar to that for surgical patients, and is associated with increased mortality and morbidity. A meta-analysis of more than 23,000 ischemic stroke patients suggested that low-dose LMWH therapy rather than unfractionated heparin (UFH) or placebo had the best risk-benefit profile, decreasing the risk of both DVT and PE without an increased risk of spontaneous intracerebral hemorrhage (sICH). The PREVAIL study, a randomized trial of 1762 ischemic stroke patients, found that 40 mg of once-daily enoxaparin reduced the risk of VTE by 43% compared with UFH (5000 units subcutaneously twice daily) including inpatients with a National Institutes of Health Stroke Scale (NIHSS) score greater than or less than 14. The rate of symptomatic ICH was similar but there was a small increase in the rate of extracranial bleeding in the enoxaparin group (1% versus 0%).
There is less evidence about optimal VTE prophylaxis in patients with hemorrhagic strokes, although the risk of DVT may be up to four times greater in ICH than in patients with ischemic stroke. The increased risk is likely related to reduced rates of pharmacoprophylaxis and a higher degree of neurologic impairment. Abrupt cessation and reversal of oral anticoagulation also may increase this risk, although one study found that patients with oral anticoagulation–related hemorrhages were not at increased risk of thromboembolic events. Review of data from the National Hospital Discharge Survey demonstrates that of 14 million patients with ischemic stroke and 1.6 million patients with ICH the incidence of PE and DVT were 0.51% and 0.74% and 0.68% and 1.1%, respectively.
The incidence of VTE after ICH may be reduced with the addition of intermittent pneumatic compression to elastic stockings and may be further reduced with administration of low-dose UFH administered three times a day when started 2 days after ICH. Pharmacoprophylaxis with either UFH or LMWH may safely be initiated 2 days after ictus. The American Heart Association and American Stroke Association (AHA-ASA) guidelines for ICH management recommend intermittent pneumatic compression in addition to elastic stockings for prevention of VTE (class I, level B evidence) and consideration of low-dose subcutaneous LMWH or UFH after 1 to 4 days from onset in patients with immobility and documented cessation of bleeding.
Diagnosis and Surveillance for Venous Thromboembolism
The presence of clinical symptoms is not a reliable marker of DVT, and hospital-acquired VTE is usually clinically silent. Traditional clinical features such as a tender, warm, and swollen calf; a positive Homans’ sign; and venous dilation are unreliable. The vast majority of patients with PE exhibit no stigmata of DVT. The highest risk of pulmonary embolism is associated with thromboses of the proximal veins above the popliteal fossa. In less than 1% of patients, calf vein thrombi cause PE. DVTs of the proximal leg contribute to 90% of pulmonary emboli, whereas the remainder is thought to arise from the pelvis or the upper extremities.
The gold standard diagnostic test to identify a DVT remains contrast venography; however, it is being rapidly replaced with Duplex ultrasonography (DUS) given the invasive nature, technical demands, costs, and potential risks of venography. For proximal DVT, ultrasonography has diagnostic sensitivities of 89% to 96% and specificities of 94% to 99%. The sensitivity and specificity of DUS is lower for symptomatic calf DVT, 73% and 93%, respectively. It should be noted that for asymptomatic patients DUS has a sensitivity of only 50%; therefore DUS should only be performed when there is a clinical suspicion of DVT. Radiologic imaging is necessary for the diagnosis of PE in patients with at least an intermediate pretest probability of PE.
D-dimer is protein produced when cross-linked fibrin is degraded by plasmin, the principal enzyme involved in fibrinolysis. D-dimer has been studied extensively. Although D-dimer levels coupled with a low pretest probability of DVT may reliably exclude DVT in some populations, its utility in hospitalized or critically ill patients is limited. In some patients with confirmed DVT, the D-dimer level may be normal.
Routine ultrasound surveillance of lower extremities has been proposed to decrease the PE rate, especially in neurologically injured patients often with altered mental states and limb immobility. A decision and cost effectiveness analysis demonstrated that adherence to pharmacoprophylaxis resulted in fewer DVTs and was eight times less costly per quality adjusted life-year. The authors concluded that optimizing thromboprophylaxis was a better value in terms of cost and health gains when compared with routine ultrasound screening.
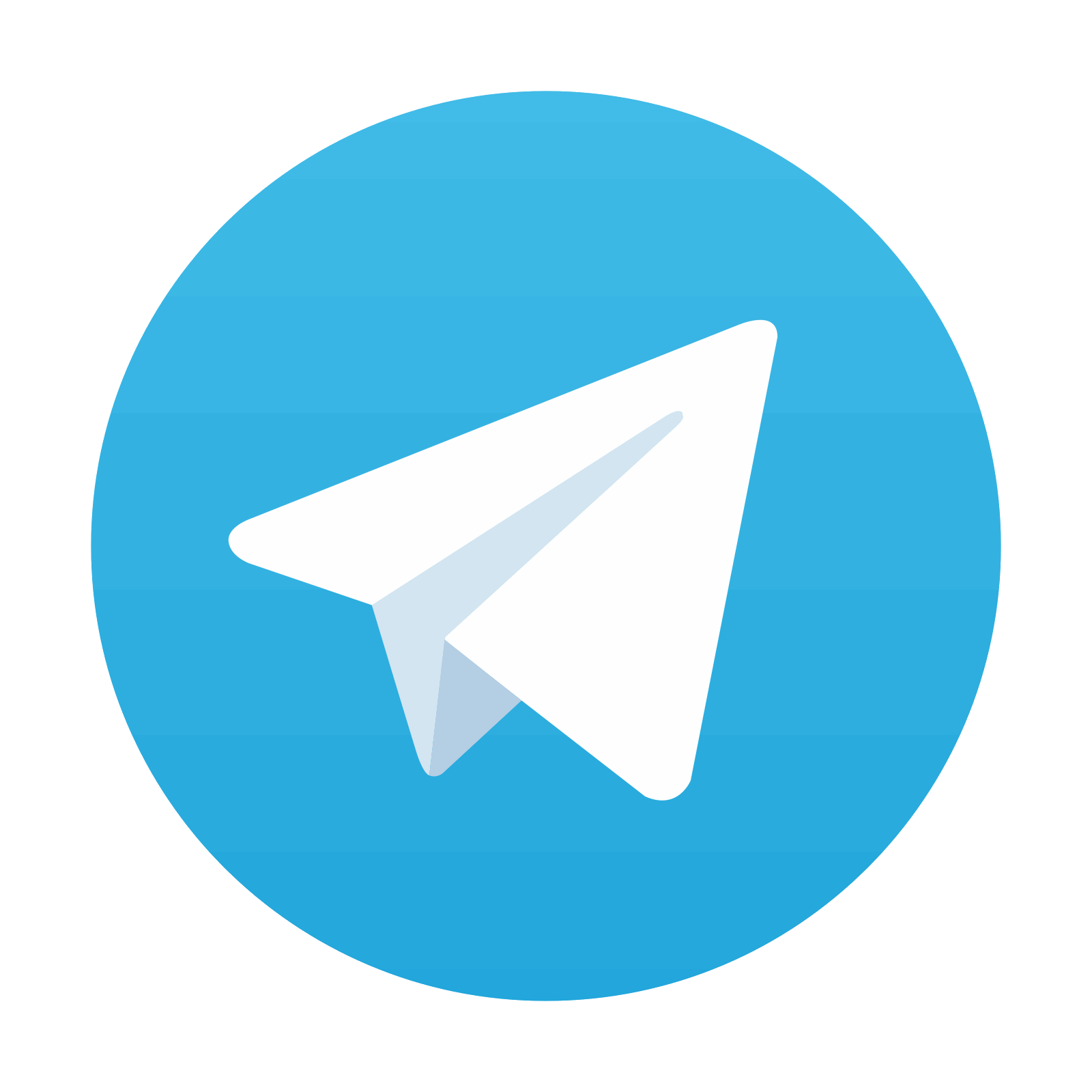
Stay updated, free articles. Join our Telegram channel

Full access? Get Clinical Tree
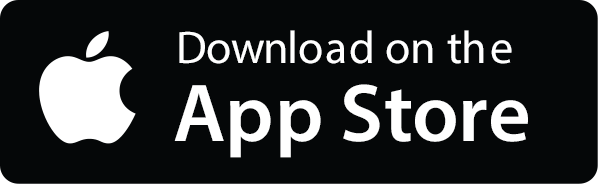
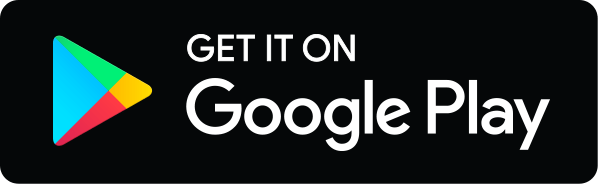