Figure 84.1. Patient’s position for anatomical hemispherectomy.
The head is then shaved and a “T”-shaped incision planned to allow access from the floor of the middle fossa to the midline of the head. Superficial landmarks useful for incisional planning include anatomic midline from nasion to inion, the lateral edge of the anterior fontanelle, the transverse sinus location, the greater wing of the sphenoid bone, and the zygomatic arch (Fig. 84.2).
Figure 84.2. Important superficial landmarks, “T” incision and planned.
The T incision is designed by a line at least 0.5 cm from midline and a perpendicular line from the zygomatic root just anterior to the tragus. The midline incision extends from the hairline to a point 4 to 5 cm above the inion. The incision is made with caution in the younger patient with an open anterior fontanelle to avoid inadvertent sagittal sinus injury. The skin edges are then reflected and periosteum and temporalis muscle fascia visualized. The muscle is mobilized off the underlying bone with a “T” incision, reflecting each muscle cuff inferiorly. Burr holes are done at the keyhole, the floor of the middle fossa just above the zygomatic arch, and lastly along the parasagittal areas just off the midline to avoid sagittal sinus injury (if anterior fontanelle is closed). The optimal craniotomy flap allows exposure to the midline, orbitofrontal base, floor of the middle fossa, and total length of the sylvian fissure. The craniotomy flap is carefully removed with a high-speed air-drill craniotome.
After the dura mater is opened in an H fashion, the sylvian fissure is identified and venous drainage patterns inspected. The distance from the superior craniotomy edge to the interhemispheric fissure is verified. The locations of major draining veins to the sagittal sinus are noted and carefully protected until later in the procedure to avoid early and often devastating blood loss. The orbitofrontal region is inspected and the position of the olfactory tract visualized as an anatomic guide to the gyrus rectus and midline structures (Fig. 84.3).
Figure 84.3. “H” dural opening and hemimegalencephalic brain.
The dissection of the sylvian fissure begins with early exposure and control of the middle cerebral artery trunk in the sylvian fissure just distal to the lenticulostriate branches. The sylvian fissure is split along its entire length using bipolar electrocautery, suction, and sharp microdissection (loupe magnification is preferred for this portion of the procedure). This should be done carefully to minimize bleeding, but cortex can be aspirated as necessary to aid in exposure. Once opened, the insular cortex including the inferior and superior circular sulci should be visualized along the length of the sylvian fissure.
The middle cerebral artery is then ligated with bipolar cautery and surgical hemostatic clips (Fig. 84.4). The inferior circular sulcus is identified and the white matter of the temporal stem is localized just deep to the sulcus. Using suction aspiration, the white matter is removed along the temporal stem, and the temporal horn of the lateral ventricle is entered. A cottonoid patty is placed here to protect the choroid plexus and prevent blood from entering the ventricular system. The pial dissection along the anterior (temporal) aspect of the sylvian fissure is carried below the main sylvian vein to the floor of the anterior aspect of the middle fossa. The anterior temporal pole is then aspirated to expose the edge of the tentorium. The white matter dissection of the temporal stem is then continued posteriorly to achieve exposure of the temporal horn from the anterior aspect to the trigonal region (Fig. 84.5). A long, thin cottonoid is then placed posteriorly into the ventricle passing from the trigone up into the lateral ventricle.
Figure 84.4. Exposure of superior and inferior circular sulcus surrounding insula.
Figure 84.5. Temporal horn access through inferior circular sulcus and identification of important landmarks for mesial structures dissection.
The posterior trigonal area is then plugged with a large cotton ball to prevent blood from entering the lateral ventricle. Exposure of the tentorial edge and basomesial temporal pia is then achieved by dissection of the lateral ventricular sulcus (collateral eminence) from within the temporal horn, just lateral to the hippocampus. This can be done with bipolar coagulation and suction or ultrasonic aspiration. In either case, the amygdala, hippocampus, and choroid plexus are protected from injury with cottonoid patties. Once the mesiobasal pia is identified just lateral to the parahippocampal gyrus, the dissection can be extended anteriorly to meet the prior pial dissection at the floor of the anterior middle fossa. The parahippocampus is then aspirated to identify the tentorial edge. The tentorial edge is then followed from anterior to posterior, curving back behind the mesencephalon. At this point, the posterior cerebral artery branches can be ligated as they pass from the perimesencephalic cistern over the tentorial edge to the temporooccipital cortex. At the conclusion of this phase of the operation, the temporal lobe lateral to the parahippocampal gyrus has been disconnected and the posterior cerebral artery branches divided. The amygdala, hippocampus, and a remnant of the parahippocampal gyrus remain in place.
Suprasylvian dissection through the superior limiting (circular) sulcus of the insula takes place to divide the coronal radiata and expose the lateral ventricle along its length. This can be done by careful dissection from above the insula or by following the previous trigonal ventricular opening around the posterior aspect of the insula to the lateral ventricle (Fig. 84.6). Dissection is facilitated by dividing the posterior branches of the MCA at the end of the sylvian fissure. Once the corona radiata is divided, the entire length of the lateral ventricle is opened and the foramen of Monro plugged with a small cotton ball to prevent blood from entering the dependent ventricular system. Care should be taken to protect the choroid plexus to avoid unnecessary bleeding. Similarly, basal ganglia disruption can be prone to bleed and is best controlled by the application of hemostatic agents to the exposed surfaces.
Figure 84.6. Opening of lateral ventricular system and corpus callosotomy. (Tip of the shunt from the opposite hemisphere is also seen.)
The corpus callosum is identified from within the ventricle at the junction of the septum pellucidum and the roof of the lateral ventricle. Aspiration of the roof of the lateral ventricle just above this area leads to the gray matter of the ipsilateral cingulate gyrus and falx cerebri. This is meticulously aspirated to prevent injury to the contralateral cingulum. Once this area is exposed, identification of the pericallosal arteries and corpus callosum proper is easily achieved. The corpus callosum and ipsilateral cingulate gyrus are then aspirated from the genu to the splenium. Complete sectioning is important to achieve and can be accomplished by following the pericallosal artery as it closely follows the characteristic course of the callosum. Special attention should be given to the genu and splenium to assure complete disruption of the horizontal fibers. Additional assistance is achieved by removal of the cingulate gyrus and identification of the inferior edge of the interhemispheric falx. Finally, the ipsilateral fornix is disrupted by aspiration at a point just anterior to the splenium. Next, the mesial dissection should continue anteriorly coagulating and dividing the pia of the ipsilateral mesial frontal lobe including the arterial branches from the anterior circulation. This mesial frontoparietal disconnection is followed anteriorly to the base of the frontal lobe just above the olfactory nerve (frontal pole). Posteriorly, the edge of the falx is followed as it transitions to the tentorium. This mesial parietooccipital resection should connect with the basal temporal disconnection below the sylvian fissure, which was performed earlier. At this point, the callosum is disconnected and the pia along the mesial aspect of the entire hemisphere is coagulated and divided. The only remaining portion of the hemisphere in place is the basal–frontal lobe below the genu and the draining veins to the venous sinuses.
The last remaining pia to be divided extends from the anterior aspect of the sylvian fissure down along the posterior–basal–frontal lobe. This pia is coagulated and divided along with the MCA branches to the frontal cortex. The posterior–basal–frontal lobe is aspirated, maintaining a plane just anterior to the anterosuperior insula (Fig. 84.7).
Figure 84.7. Important surgical landmarks of right frontobasal disconnection.
The orbitofrontal pia is then coagulated and divided down to the olfactory nerve, and the pia overlying the gyrus rectus is identified and divided. The gyrus rectus is then aspirated to expose the contralateral gyrus rectus and a cottonoid patty placed to mark the midline. The pial dissection along the olfactory nerve is then carried anteriorly to avoid disruption of the nerve. The remaining gyrus rectus is then aspirated with the posterior removal limited by the internal carotid artery. The deep white matter and mesial frontal gyri are removed in subpial fashion by a dissection plane marked by the anterior aspect of the frontal horn starting below the prior dissection of the genu of the corpus callosum. This dissection is carried out through the caudate nucleus along the course of the anterior cerebral artery to where it joins the internal carotid artery. Special care should be taken after the hemisphere is removed to ensure complete removal of the basal–posterior–frontal lobe. Once all the pial surfaces and white matter tracts have been cut, the draining veins to the sinuses are circumferentially coagulated and divided and any bleeding points packed with hemostatic agent. At this point, the entire hemisphere can be removed in one anatomic piece and sent for pathologic study. The remaining amygdala–hippocampus bloc is then removed as the last portion of the procedure.
The insular cortex can be removed if so desired by subpial aspiration using the ultrasonic aspirator or suction coagulation. As the middle cerebral artery has already been controlled, arterial injury is of less concern than in the functional hemispherectomy operation. Care must be taken to limit resection to the insular gyri to avoid injury to deeper subcortical structures. Perhaps stereotactic imaging would be useful at this stage, although a practical approach is to stop the dissection when underlying white matter is reached.
Adams Modification of Anatomic Hemispherectomy
Adams modification was an attempt to avoid the complications of hemosiderosis and hydrocephalus. The classic anatomic hemispherectomy is supplemented by a muscle plug in the foramen of Monro on the resection side and by folding down the stripped dura of the convexity bone onto the falx, central block (composed by basal ganglia and thalamus and middle fossa cavity). The subdural space is occluded and outflow of cerebrospinal fluid from the opposite side is prevented. Using this technique, there seems to be a higher rate of infection, but the rate of hydrocephalus seems to be reduced, compared to the classic anatomic resection (8).
Functional Hemispherectomy and Other Disconnection Techniques
Classic Functional Hemispherectomy
Rasmussen et al. proposed “functional hemispherectomy,” minimizing tissue resection (5–7). The overall goal of the functional hemispherectomy is to disconnect the frontal lobe (through an incision placed just anterior to the genu of the corpus callosum) and to disconnect the parietal and occipital lobe (through a posterior incision) and remove the temporal lobe and central part of the frontal and parietal lobe. The fiber tracts projecting from the remaining parts of the frontal, parietal, and occipital lobes to the brainstem and spinal cord are then transected. The blood supply to the disconnected cortical regions is kept intact.
In the functional hemispherectomy procedure, T-shaped scalp incision (similar to one described under anatomic hemispherectomy) is performed. The craniotomy is smaller than in the anatomical hemispherectomy, especially in the anterior–posterior orientation, and is mainly centered in the topographic location of the insula. The dura is opened in the same manner as it has been described for the anatomical hemispherectomy.
The first cortical incision is made along the upper margin of the sylvian fissure, by coagulating and incising the pia and its blood vessels, dissecting down into the frontal and parietal operculum, down to the plane of the insular cortex. From the anterior and posterior ends of this dissection, a central resection is performed, exposing the entire sulcus limitans of the insula and, consequently, the insula cortex. The incisions are extended to the medial surface to the level of the cingulate gyrus, which is preserved at this stage to protect the pericallosal artery, but removed later. By deepening the dissections in the superior sulcus limitans of the insula, the body of the lateral ventricle is entered and the central bloc of tissue removed.
The temporal lobe is removed by coagulating and dividing the pia and its vessels along the superior temporal gyrus, back to the posterior limb of the upper resection, and anteriorly around the temporal pole, down to the uncus. The roof of the temporal horn is entered and then the lateral portion of the temporal lobe is removed through the collateral sulcus. The hippocampus is dissected and removed through the coagulation of the hippocampus sulcus. The hippocampus is dissected free, and the amygdaloid nucleus is removed.
The deep white matter of the medial and inferior aspects of the frontal lobe is divided in the coronal plane, from the central resection area to the most basal and posterior area of the frontal lobe, just rostral to the anterior perforated substance and medial and lateral olfactory striae. The anterior portion of the corpus callosum is also divided, from its body to the knee and rostrum portions, stopping at the level of lamina terminalis. In the same way, the white matter of the parietal lobe is divided posterior to the splenium from the ventricular ependyma, from the body and atrium of the lateral ventricle to the pia overlying the falx and the floor of the middle fossa. Following subpial dissection, the cingulate gyrus is removed. In the same way as the anterior callosotomy, the posterior corpus callosum is also divided, from the topography of the central resection to the splenium. Just anterior to the splenium, the fimbriae and fornices from both hippocampus formations join, forming the hippocampus commissure, which will need to be completely disconnected. After irrigation, the craniotomy is closed as previously described for anatomical hemispherectomy.
Hemidecortication
It is based on the principle that only the epileptogenic cortex needs to be removed in order to achieve seizure freedom. The concept was first delineated by Ignelzi and Bucy in 1968 (9). The integrity of the lateral ventricle is largely preserved, except at the temporal lobe, where removal of the hippocampus requires opening of the temporal horn. Although the main aim is to avoid opening the ventricular system, removal of the hippocampus makes opening of the temporal horn a necessary step. In this procedure, a large wound surface is created, and, in cases of HME, where dysplastic ectopic gray matter is located in the white matter, orientation can be difficult.
Transsylvian, Transventricular Functional Hemispherectomy
This approach was developed and refined by Schramm and colleagues (12). The key features of this approach are (i) small craniotomy and transsylvian exposure of the insular cortex; (ii) anterior mesial temporal lobe resection, including amygdala and hippocampus; (iii) transcortical access to the ventricular system through the limitans sulcus of the insula, from the tip of the temporal horn to the tip of the frontal horn; (iv) frontal–basal disconnection anterior to the anterior cerebral artery; (v) mesial disconnection following the anterior cerebral artery through the anterior portions of the corpus callosum to the splenium; and (vi) posteromedial disconnection in the ventricular trigone following the outline of the falcotentorial border to the temporomesial resection cavity. This procedure is especially suited for cases with enlarged ventricles, porencephalic cysts, and marked atrophy of the insula–basal ganglia block or for cases with larger ventricle and cisterns.
The size of the craniotomy is chosen guided by the length of the corpus callosum, the anteroposterior diameter of the basal ganglia thalamus–insula block (limen insulae to pulvinar), and the degree of ventricular enlargement. The sylvian fissure is then opened, and the circular sulcus is exposed, taking advantage of the fact that the temporal operculum is overlying the inferior limb of the limitans sulcus only about 0.5 to 1 cm, whereas the frontal operculum can overlie the frontal limb of the circular sulcus up to 3 cm. Access to the temporal horn is gained through the inferior circular sulcus approach. The uncus and the lateral parts of the amygdala are removed, and the hippocampus also is taken out either by suction or en bloc. Sparing the major branches of the middle cerebral artery, the ventricular system is then opened all around the insular cortex. From inside the anterior horn of the lateral ventricle, a dissection line is now created by suction and bipolar coagulation from the frontal horn floor, just anterior from the foramen of Monro, down to the basal arachnoid, just anterior to the middle and anterior cerebral arteries. The mesial disconnection can now be continued around the corpus callosum following the anterior cerebral artery. Callosotomy is then performed within the ventricle, back to the area of the splenium. The fornix and the hippocampus tail are disconnected and resected, until the mesial temporal lobe resection cavity is reached.
According to Schramm and colleagues (12), the transsylvian–transventricular hemispherectomy with only a minimal mesial temporal lobe resection should not be used for HME cases, even if ventricles are enlarged, for two reasons: the insular cistern may be atypically configurated and the transsylvian approach can be more difficult even with enlarged hemisphere. In HME, the transsylvian–transventricular hemispherectomy should be combined with resection of the entire temporal lobe or with resection of the frontal operculum to the level of the insular cortex. This resection facilitates the transcortical access from the limitans sulcus of the insula to the lateral ventricle and creates room for postoperative swelling.
According to Bonn series, possible disadvantages of this procedure include problems identifying anatomical landmarks due to the limited exposure. Hydrocephalus, possibly induced by the large wound surface and the transventricular approach, was not seen in the transsylvian “keyhole” hemispherectomies for all causes so far. No case of incomplete disconnection toward the midline was detected, but too anteriorly placed disconnections were seen.
Peri-Insular Hemispherotomy
Peri-insular hemispherotomy was initially developed by Villemure and Mascott (11). The main features of this approach are (i) medium-sized craniotomy exposing the frontal, parietal, and temporal operculum in the whole length of the sylvian fissure; (ii) resection of the frontal and parietal operculum and underlying white matter, opening the whole lateral ventricle through the anterior and superior limitans sulcus of the insula and disconnection of the frontobasal area through the intraventricular approach; (iii) resection of the temporal operculum (T1 gyrus and underlying white matter) and exposure of the temporal horn through the inferior limitans sulcus of the insula; (iv) mesial disconnection through the corpus callosum, from the rostrum and knee to the splenium; and (v) temporomesial disconnection with only anterior resection of the amygdala, anterior aspect of the hippocampus and uncus.
Peri-insular hemispherotomy is best indicated in patients with enlarged ventricle and certain degree of atrophy, and because of the more extensive resection of the operculum and underlying white matter, it can be also applied for HME cases. Kestle et al. used this technique in 11 of their 16 cases. Estimated blood loss was 462 mL, compared to 1.3 L for decortication, 73% of their patients needed a transfusion, and there was no need for shunts (29).
Vertical Parasagittal Hemispherotomy
This approach was first described by Delalande et al. in 1992 (10). It includes initially a small parasagittal craniotomy, followed by complete callosotomy with opening of the roof of the lateral ventricle. Once the entire lateral ventricle is unroofed, posterior disconnection of the hippocampus is achieved by cutting the columns of the fornix at the level of the ventricular trigone. The vertical incision is performed lateral to the thalamus, choroid plexus, and choroidal fissure of the temporal horn, then following the temporal horn from the trigone to the most anterior part of the ventricle, keeping the incision in the white matter. The callosotomy is then completed by resecting the genu and the rostrum of the corpus callosum to the anterior commissure. The next step is the resection of the posterior part of the gyrus rectus, which will allow the visualization of the anterior cerebral artery and optic nerve and provide enough space for the last disconnection step, which is a straight incision anterolaterally through the caudate nucleus from the rectus gyrus to the anterior temporal horn.
ANATOMICAL HEMISPHERECTOMY VERSUS FUNCTIONAL HEMISPHERECTOMY AND OTHER DISCONNECTION TECHNIQUES
The discussion about what would be the appropriate surgical technique for treatment of intractable hemispheric epilepsy is controversial. The literature is full of personal series, specifically reporting seizure outcome and complications related to one specific technique. In addition, most of the studies are retrospective in nature, reporting results in populations that differ in age, severity of seizure, and pathologic substrate. There are no studies that directly compare functional versus anatomical hemispherectomy. In the largest series of patients treated with anatomical hemispherectomy, the surgical outcome is similar to that for functional disconnection (25,29–34). The group at Johns Hopkins reviewed their experience with anatomical hemispherectomy in infants and children (18,22). Of 21 patients with cortical dysplasia, 8 (38%) were seizure free and an additional six (29%) had mild seizures after surgery. In contrast, surgical series after functional hemispherectomy for CD report 50% to 67% of seizure-free rate with an additional 11% to 33% having only rare seizures. Although number are small in all these studies and the radiologic involvement of CD is not well outlined in some of these reports, these results suggest that functional hemispherectomy is at least as effective as anatomical hemispherectomy. The frequency of complications may also be lower after functional hemispherectomy. In one series, of five patients with HME who continue to have seizures after functional hemispherectomy, three had seizures that arose from the operated hemisphere and two had seizures arising from the contralateral hemisphere. These results suggest that anatomic hemispherectomy may be more effective in patients with HME and that functional hemispherectomy may be better suited for patients with more restricted hemispheric cortical dysplasia. At the Cleveland Clinic, we believe that anatomical hemispherectomy provides a better seizure outcome in patients with cortical dysplasia and HME. In a series of patients with catastrophic epilepsy in young ages, incomplete disconnection was the only variable statistically associated with persistent seizures after surgery (14). In a total of 18 patients, 6 patients had persistent seizures after surgery. Two patients had the diagnosis of HME and four had cortical dysplasia. From this group, four patients had incomplete disconnection, always located in the posterior basal–frontal areas. All four patients underwent reoperation, converting the procedure to anatomical hemispherectomy. All four patients achieved seizure freedom. In our studied group, we found that for patients with HME, anatomical hemispherectomy is a better option. In general, the lateral ventricle from patients with HME is characterized by an irregular shape, a relative hypoplasia of the temporal horn. Such anatomical peculiarities, together with irregular and abnormal thickness of the cerebral mantle, deep heterotopic gray matter, distorted trajectory of the anterior cerebral arteries, abnormal large veins at the level of the malformed sylvian fissure, and the possible interdigitation of the mesial aspect of the hemispheres, make the functional hemispherectomy a technically difficult procedure in this group of patients. In our surgical series, all patients with HME who underwent functional hemispherectomy resulted in uncontrolled seizures after surgery; the conversion to anatomical hemispherectomy resulted in seizure freedom in all patients (14).
Although several authors reported higher complications rates in anatomical hemispherectomy (5,35–37), particularly hemosiderosis and secondary hydrocephalus, we did not observe such findings in our patients. With specific regard to hemosiderosis associated with anatomic hemispherectomy, one could speculate whether late mortality from hemispherectomy was caused by the effects of chronic deposition of Fe2+ on the cerebral parenchyma, from repeated intracranial hemorrhages, or it was simply the outcome of hydrocephalus, which escaped detection before the introduction of computerized tomography (CT). It is worth noting that reports concerning hemosiderosis have been practically absent in the literature since the 1970s; nevertheless, hemosiderosis is still cited as a common reason for avoiding anatomic hemispherectomy.
OUTCOME AFTER HEMISPHERECTOMY
There are different perspectives in assessing outcome after hemispherectomy. Seizure outcome is the primary concern, but the long-term functional outcome is equally important in improving the quality of life. Additionally, morbidity associated with the different procedures has to be considered.
Seizure Outcome After Hemispherectomy
In various cross-sectional studies on outcome after hemispherectomy, the seizure-free rates range from 52% to 80% (38–43). We recently examined longitudinal outcome of 170 children who underwent hemispherectomy over a 10-year period at the Cleveland Clinic (38). At a mean follow-up of 5.4 years, 66% of children were seizure free (Engel 1a). Of 58 patients with seizure recurrence, 8 had late remission (seizure free for 1 year or more at last follow-up) and another 16 patients had >90% reduction in seizures (38). Overall, 80% of children were either seizure free or had major improvement after hemispherectomy. Similar seizure outcome has been reported after hemispherectomy in adults with refractory epilepsy due to large middle cerebral artery infarct or cortical malformation (44).
In the Cleveland Clinic hemispherectomy series, age at seizure onset, age at surgery, epilepsy duration, etiology, presence of generalized epileptiform abnormalities, bilateral abnormalities on brain MRI, and type or side of hemispherectomy had no effect on seizure outcome. Prior hemispheric surgery, nonlateralized ictal EEG, acute postoperative seizures, and bilateral abnormalities on PET were predictive of poor seizure outcome on univariate analysis. On multivariate analysis, only the latter two emerged as independent predictors of poor outcome. In one of the earlier reports, children with malformation tended to have poor seizure outcome; we did not find etiology as a predictor in our study. At our center, we favor more tissue resection, not infrequently anatomic hemispherectomy, in children with HME for reasons cited in the earlier section. Head-to-head comparison between different techniques of hemispherectomy is not feasible as most centers tend to do favor one particular technique. Overall, the reports from various centers do not suggest a major difference in seizure outcome with different techniques.
Seizure recurrence after hemispheric disconnection procedure indicates either continued seizures from operated side due to incomplete disconnection or independent epilepsy arising from the opposite hemisphere. Seizure semiology, interictal and ictal EEG findings, and MRI may assist in differentiating in some patients. However, in our experience, EEG findings after disconnective hemispherectomy are complicated and may be unhelpful. It is difficult to reliably confirm the completeness of resection by MRI as well. At our center, in many such cases with failed functional hemispherectomy, we proceed to perform removal of residual tissue on the operated side (thereby converting to anatomic hemispherectomy) to ensure effective hemispherectomy. This strategy has helped in achieving seizure freedom in a third of patients and major improvement with >90% seizure reduction in as high as two-thirds of patients (38,45).
Functional Outcome After Hemispherectomy
Several studies in the past addressed the functional outcome after hemispherectomy. Although most studies do not have a comparative group to study the effects of the surgery per se, most clinicians firmly believe that successful hemispherectomy improves functional outcome as well (46,47). From a practical standpoint, the alternative course of persistent daily seizures and continued worsening of neurologic status is unacceptable to most families.
We recently reported the functional status of 115 children who underwent hemispherectomy at the Cleveland Clinic (47). In our cohort of 115 children, 92% were able to walk independently or with assistance (Fig. 84.8). Children with bilateral motor deficits (worse on the side concordant with surgery) or MRI abnormalities in the unoperated hemisphere or seizure recurrence were more likely to have poor motor outcome. Over two-thirds of children in this series had spoken language skills sufficient for regular conversation; nearly half of these children had age-appropriate language abilities as perceived by their families. Preoperative language delay, bilateral MRI abnormalities, and seizure recurrence were associated with poor language outcome. Age at seizure onset and left-sided surgery had no significant impact on the language outcome in this cohort. Reading abilities were poor in 59% of children and only 18% of children had age-appropriate reading ability. Per parental report, 73% of patients had minimal to no behavioral problems; the rest had significant problems in home and school environment. Children with postoperative seizure recurrence were more likely to have behavioral problems. Although visual field defect is expected to be present in every patient, families did not perceive it as a major handicap. Patients were accustomed to the defect and were able to take precautions to avoid major mishaps (47).
Figure 84.8. Functional outcome after hemispherectomy in 115 children. Mean age at follow-up was 12.7 (±6) years. Reading status was assessed in children older than 6 years (n = 105).
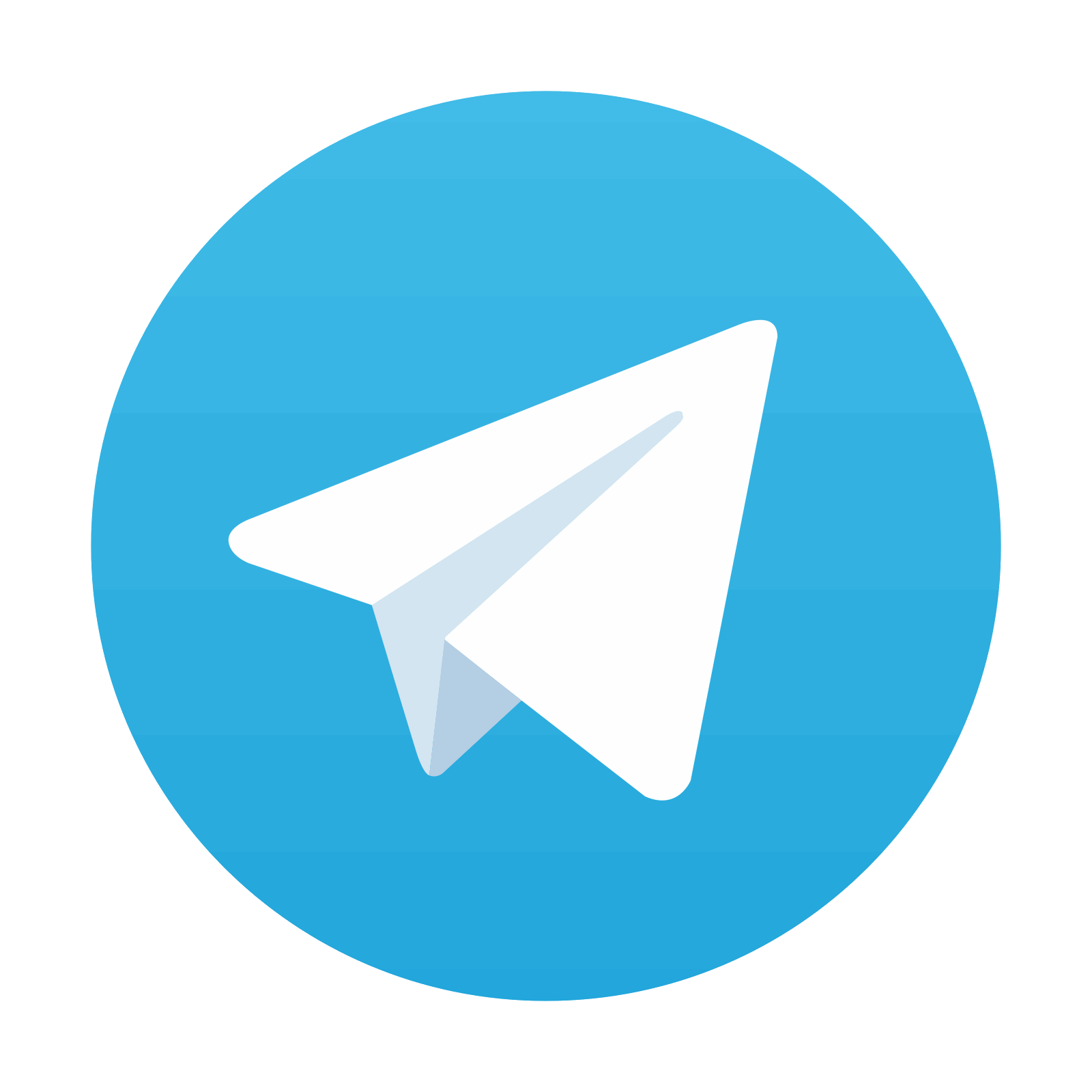
Stay updated, free articles. Join our Telegram channel

Full access? Get Clinical Tree
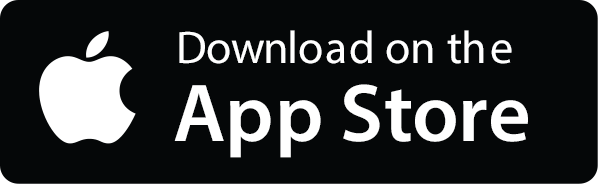
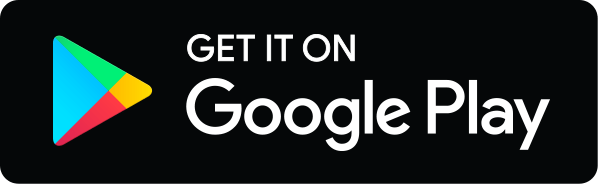