Fig. 21.1
MR flow imaging. (a) T2 sagittal MR image of craniovertebral junction and spinal canal. (b) Corresponding phase contrast MR images demonstrating caudal displacement of CSF (seen as black), during systole. (c) The same image during diastole, with return of CSF in a cephalad direction. The three red, horizontal lines on images “a” and “b” show the levels of the transverse image pairs “d”, “e” and “f”, respectively. The graph “g” shows the flow volume, with time, across the cardiac cycle
Although many studies of the CSF flow in Chiari malformation have been performed, the impact such measurements in clarifying the cause of syringomyelia is still a matter of debate. Martin et al. summarised previous theories and their own results (Martin et al. 2010). We have performed quantitative pulsatile CSF flow measurements in patients with Chiari 0 malformation and Chiari type I malformation, without and with syrinxes of different sizes (Bogdanov et al. 2004). We compared CSF flow patterns in the subarachnoid channels, spinal cord and syrinxes in Chiari type I malformation patients and controls (Haughton et al. 2003). Our measurements cannot decide if CSF is driven into spinal cord or is generated in the spinal cord itself, but we can demonstrate the hydrodynamic steps in the development of the hindbrain hernia and the syrinx. Depending on the degree of flow obstruction at the foramen magnum, we see a significantly increased pulsatile CSF flow volume and velocity in the subarachnoid channels and spinal cord, beginning just below the craniocervical junction. Lower down, enlargement of the spinal cord by the syrinx impedes pulsatile CSF flow in the subarachnoid channels and allows the flow velocity to increase further. The fluid in the spinal cord, however, maintains nearly the same pulsatile flow volume and velocity as in the section above, just below the foramen magnum, due to the low hydrodynamic fluid resistance of the syrinx. The pulsatile hydrodynamic power is thereby transferred from the subarachnoid channels to the spinal cord and from the spinal cord to the syrinx. In the section of spinal canal below the syrinx, pulsatile flow power of the cavity itself is redistributed to the subarachnoid space, which provokes a transient increase in the velocity in the subarachnoid CSF channels. With progression of the disease, this hydrodynamic power decreases continuously in the subarachnoid CSF channels and increases correspondingly in the syrinx.
In our measurements, the subarachnoid space, spinal cord and syrinx appear to be communicating compartments that are in a hydrodynamic equilibrium. The subarachnoid channels have the lowest resistances and therefore allow the highest flow velocities. The diameter of the subarachnoid space decreases at the location of the syrinx. This leads to higher resistance and thus a decrease in the flow volume while the flow velocity increases or is maintained. The spinal cord has a high hydrodynamic resistance, because of the higher tissue density of the extracellular space. Inside the syrinx, depending on the properties of the cavity, the resistance is similar or slightly higher than in the subarachnoid space. The transfer of the pulsatile flow wave from the subarachnoid channels to the spinal cord and syrinx leads to a time lag between the flow peaks of subarachnoid space and syrinx. The pulsatile force of the increased flow volume is transferred to the perivascular and extracellular fluid of the spinal cord and to the syrinx. The pathological CSF flow hydrodynamics appears to become self-reinforcing and transfers more and more hydrodynamic energy from the subarachnoid space to the syrinx.
It is a characteristic for the pathophysiology of syringomyelia in Chiari type I malformation that development of the syrinx does not start immediately below the foramen magnum but mainly somewhat more distal, from where it spreads upward and downward as the disease progresses. The accumulation of extracellular fluid decreases the cross-section volume of the subarachnoid space, which intensifies the already pathologically high subarachnoid flow disturbances. Under these conditions the accumulation of fluid in the spinal cord merges in a pre-syrinx and finally syrinx, which again increases the flow disturbances, by reducing the volume of the subarachnoid space. The subarachnoid channels, spinal cord, pre-syrinx and syrinx behave as communicating compartments, with different hydrodynamic resistances. Thus, the hydrodynamic power is more and more transferred or changed from the subarachnoid space, via the spinal cord, into the syrinx.
References
Almen T (1969) Contrast agent design. Some aspects on the synthesis of water-soluble contrast agents of low osmolality. J Theor Biol 24:216–226PubMedCrossRef
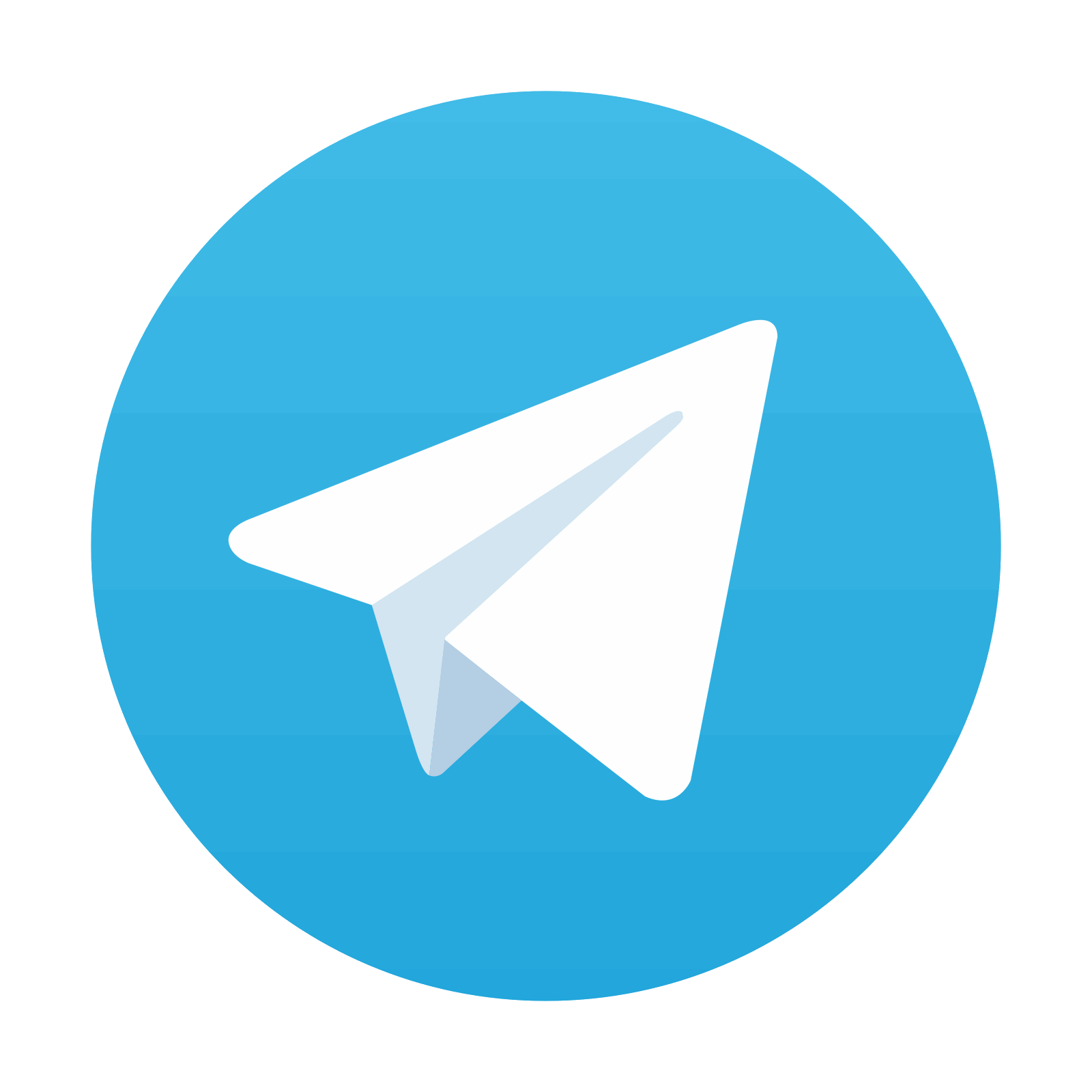
Stay updated, free articles. Join our Telegram channel

Full access? Get Clinical Tree
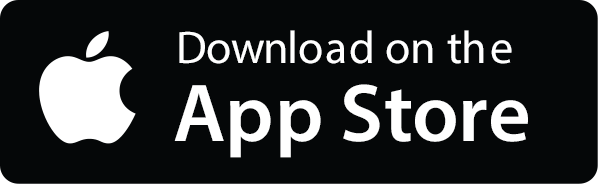
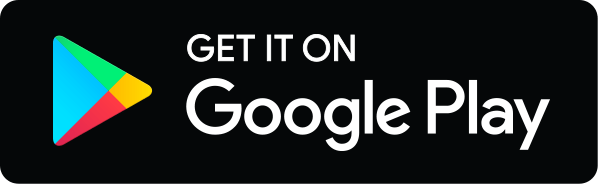