Abstract
The utility of induced hypothermia to mitigate injury to the brain has been long debated in medicine, and it may be the most studied neuroprotectant strategy in traumatic brain injury (TBI). Hypothermia has effects on dozens of injurious processes and animal data demonstrates that its application after TBI leads to outcome improvements. Hypothermia was used as standard practice decades ago and has been subjected to rigorous clinical trials. While hypothermia consistently lowers intracranial pressure, it has failed to alter outcomes in multicentered studies. It is possible that changing study designs to include more homogeneous patient populations, requiring more rigorous clinical protocols for other aspects of clinical care, and developing more sensitive outcome measures may reveal the anticipated benefit of hypothermia. However, it is also possible that the side effects of therapy outweigh benefits in this patient population. Despite the effort, hypothermia’s role in the management of TBI remains uncertain.
Keywords
Craniocerebral trauma, Critically ill, Hypothermia, Traumatic brain injury (TBI)
Introduction
Therapeutic hypothermia—the intentional lowering of temperature in an attempt to mitigate injurious processes—has undergone several life cycles in medical practice. Interest in hypothermia may have first been introduced into medical practice from reports centuries ago suggesting that injured soldiers may survive when kept away from heat sources. More recently, neurological protection has been observed in people who have suffered prolonged cardiac arrests while under ice-cold water ( ). In the 1960s and 1970s, therapeutic hypothermia had become incorporated into many clinical practices for cardiac arrest, traumatic brain injury (TBI), and other conditions and was essential for the development of safe cardiac bypass procedures ( ). Landmark studies have determined that hypothermia improves neurological outcomes in adults after cardiac arrest and in neonates after perinatal asphyxia ( ). However, contemporary research on the utility of therapeutic hypothermia for TBI has been much more mixed. This chapter is intended to summarize the utility of therapeutic hypothermia in TBI by reviewing proposed mechanisms of neurological protection, various management strategies, and the current clinical evidence for its use.
Mechanisms of Protection and Potential Side Effects
Hypothermic neuroprotection has been demonstrated in many preclinical models of injury, with the majority of the studies in TBI being performed in rodents. In these models, hypothermia induced near the time of injury has led to improved behavioral and histologic outcomes ( ). However, the primary mechanisms of hypothermic neuroprotection remain unclear because of the myriad effects hypothermia can have on cell function, organ activity, and metabolic processes. Hypothermia has effects on many secondary injury mechanisms including (1) cerebral metabolism, (2) excitotoxicity, (3) oxidative stress, (4) blood–brain barrier (BBB) permeability, (5) gene expression, (6) neurotrophin levels and function, (7) neuroinflammation, (8) cerebral swelling, and (9) axonal injury. This review will focus on data from TBI models and a few of the most prominent postulated mechanisms: oxidative stress, neuroinflammation, BBB permeability, and neurogenesis.
One of the most compelling examples of bench-to-bedside research of posttraumatic hypothermia has focused on the effects of hypothermia on free radical damage and oxidative stress. Multiple biochemical pathways may lead to free radical production following TBI and the central nervous system may be particularly prone to the deleterious effects of free radicals on neuronal and glial cell survival, vascular function, and neuroinflammation because of the role of lipids in the brain. In children with severe TBI [Glasgow Coma Scale (GCS) score <9], demonstrated a marked and sustained reduction in antioxidant reserve in cerebrospinal fluid over the first several days after injury. This data has been interpreted as evidence for a free radical damage time course and is supported by evidence of lipid peroxidation and protein oxidation. Hypothermia may alter this pathway if it can minimize the damage in a time-dependent manner. In a confirmatory study, found that children with severe TBI who were cooled to 32–33°C for 48 h after severe TBI had preserved total antioxidant reserves and glutathione levels.
The humoral and cellular neuroinflammatory response to TBI has been shown to be temperature dependent. Within the humoral system, two of the primary proinflammatory cytokines responsible for the pathophysiologic response to TBI, tumor necrosis factor-alpha (TNF-α) and IL-1β, are affected by hypothermia. Although hippocampal TNF-α mRNA levels were significantly reduced by posttraumatic hypothermia, TNF-α protein levels were unchanged after 3 h at 33°C. demonstrated that hypothermia decreased expression of the TNF receptor and as well as a shift in TNF signaling from proapoptotic to procell survival pathway. This model has also been used to show hypothermia reduces mRNA and protein levels of IL-1β. It has been postulated that these hypothermia effects may be related to caspase-1 activation, which is the enzyme primarily responsible for processing of pro-IL-1β to the active form and is temperature dependent. The cellular neuroinflammatory response to posttraumatic hypothermia was investigated by using a rat model with a target temperature of 32°C for 4 h; animals treated with hypothermia had a four- to eightfold decrease in acute neutrophil accumulation despite there being no effect on peripheral neutrophil counts.
Compromise of the BBB after trauma can lead to extravasation of bloodborne substances into the brain parenchyma leading to edema, inflammation, and secondary injury and is another target of hypothermia therapies. have shown posttraumatic hypothermia significantly reduced BBB permeability at 3 and 7 days after injury. Matrix-metalloproteinase-9 (MMP-9), a protein that can degrade the basal lamina leading to disruption of the BBB, is significantly increased in the cortex and hippocampus after TBI and is attenuated by therapeutic hypothermia ( ).
examined the effect of posttraumatic hypothermia on neurogenesis. They found that bromodeoxyuridine (BrdU) and doublecortin (DCX) to labeled neuroblasts were increased in the dentate gyrus at 7 days after TBI in hypothermic animals using a fluid percussion model. The methods of this study were insufficient to determine if the effect of hypothermia was based on an increase in actual neurogenesis or simply neuroprotection of existing neuroblasts from TBI-based cell death.
Management Strategies
Depth, Timing, Duration, and Rewarming
The institution of therapeutic hypothermia requires a number of clinical decisions—what is the depth of hypothermia desired, what is the intended duration, what is the timing of the therapy, and how should it be stopped? Hypothermia requires an induction phase, a maintenance phase, and a rewarming phase. The depth of hypothermia is generally defined based on the core body temperature outlined in Table 2.1 . For TBI, clinical studies are limited to studying mild and moderate degrees of hypothermia, because of the cardiovascular complications associated with deeper levels. Interestingly, invasive monitors currently in clinical use can measure the brain temperature (BT) directly. The relationship between the BT with systemic temperature has been described ( ) and may eventually be a more relevant therapeutic target. One study in children compared head cooling to normothermia, with a goal BT of 34.5 ± 0.2°C for 72 h in the experimental group compared to BT of 38.5 ± 0.5°C in the control group. The hypothermic group had significantly lower intracranial pressure (ICP) after 8 h of study inclusion. There were no differences in complications between groups except a higher rate of bradycardia in the hypothermia group ( ). In an adult study by Liu et al., systemic cooling resulted in a similar reduction in ICP as brain cooling; however, the brain cooling group had an improved neurologic outcome at 2 years after injury in comparison to the systemic cooling and normothermia groups. All three groups had a similar complication rate except for thrombocytopenia in hypothermia groups ( ).
Depth | Core Temperature (in °C) |
---|---|
Mild hypothermia | >34 |
Moderate hypothermia | 32–34 |
Severe hypothermia | 20–32 |
Profound hypothermia | <20 |
The timing of hypothermia after TBI has largely been based on preclinical data. Preclinical studies have focused on early hypothermia—within minutes to few hours after injury—as this strategy has demonstrated decreased brain edema and improved functional outcomes ( ). This approach has been adopted for large clinical trials; with the presumption that hypothermia’s benefit will wane as time passes after injury. For clinical studies, this philosophy has resulted in most studies testing hypotheses based on the fact that early hypothermia must have salutary effects on the entire population under study. Given the heterogeneity of TBI as a disease, it is likely that this has led to some of the negative trial results.
The duration of hypothermia has been long debated and no consensus currently exists. Research studies in perinatal asphyxia suggest that 72 h is beneficial, while many cardiac arrest studies have been successful with durations between 12 and 24 h. For TBI, most studies have opted for durations between 24 and 48 h, as will be reviewed in the following; however, some authors have argued that a longer duration may prove effective since edema peaks between 3 and 5 days after injury ( ). compared long-term (4–5 days) versus short-term (1.5–2.5 days) hypothermia started within 4 h of injury; the long-term group had an improved ICP control and neurologic outcome at 6 months after injury with similar complication rates between both groups.
Rewarming strategies are another variable that may have an important impact on outcomes. Most studies in TBI have demonstrated that ICP can be increased during the rewarming phase (rebound intracranial hypertension); therefore, both the method of rewarming and the rate of return to normothermia have been debated. Methodologically, rewarming can be an active process (with the application of heat sources) or a passive process (with the removal of cooling therapies). Early efficacy studies in the field used an active rewarming strategy. The rate of temperature rise to complete the therapy has also varied widely, with rates as rapid as 0.5°C every 2 h to as slow as 0.1°C every hour. Currently, the optimal strategy for rewarming method and rate are unclear.
Methods of Cooling
Hypothermia is induced and maintained by a variety of methods that include surface and intravenous cooling methods, with modalities including intravenous catheter-based systems, intravenous infusion of cold saline, and cooling blankets. The intravenous catheter-based systems, although associated with risks of central venous catheter placement (including deep vein thrombosis and thrombophlebitis) and higher costs ( ), have been shown to be more effective than other systems related to speed of induction of hypothermia as well as maintenance of temperature within desired goals. In a randomized study, compared the efficacy of an intravenous cooling catheter versus antipyretics and cooling blankets in preventing fever in patients admitted to the ICU. They found a significantly lower fever burden in patients with the intravenous cooling catheter without differences in infection rates or sedation requirements. Other methods can also achieve temperature control but often require a more regimented protocol to achieve the desired goals. randomized 50 patients to mild hypothermia (cold saline infusion and cold packs, air circulating blankets, water circulating blankets, gel-coated pads, and an intravenous heat exchanger) or standard temperature management. The water circulating blankets, gel-coated pads, and intravenous heat exchanger were significantly more efficacious, and the intravenous heat exchanger maintained temperature in target range significantly more often. For children with TBI, the use of intravascular devices is not feasible due to technical constraints of the available devices. found that iced-saline infusion in children resulted in temperature decrease of 2°C within 1 h after completion of the infusion. Combined with surface cooling this intravenous method may be the most effective way to rapidly lower temperature during the induction phase for future studies.
As mentioned earlier, targeting BT is now possible—making regional or selective hypothermia of the patient’s head an alternative method to achieving hypothermia after TBI, which may have fewer side effects. Preliminary studies suggest that custom cooling devices of the brain were effective at achieving a BT <34°C within a 2–6 h time frame while commercially available systems were not adequate to achieve this desired goal ( ). Further research into focal cooling systems is needed.
Side Effects and Complications
As with all critical care therapies, hypothermia can lead to serious complications, some of which can be injurious for patients with TBI. The most common side effect of surface cooling is shivering, which can lead to increased stress, sympathetic discharge, and muscular activity, all leading to increased oxygen demand and energy expenditure that could lead to exacerbation of brain injuries. This side effect can be mitigated by sedation and/or neuromuscular blockade and is not observed during hypothermia with intravascular cooling devices.
Hypothermia is associated with alterations in the hematopoietic and immunological systems, leading to concerns regarding hemostasis and infections in patients with TBI ( ). In a Cochrane review, hypothermia to 35°C was associated with increased incidence of pneumonia in adults with TBI, but this risk was not present for trials with good allocation concealment ( ). Hypothermia is also associated with a change in platelet number and function and changes in the clotting cascade. The clinical significance of these is unclear, as coagulopathy and thrombocytopenia appear to resolve with rewarming ( ).
Lastly, hypothermia can have important effects on cardiovascular performance. Hypothermia decreases heart rate, increases systemic vascular resistance, decreases cardiac output, and has variable effects on blood pressure (BP). Given the evidence that decreased BP and cerebral perfusion pressure (CPP) can affect outcomes after TBI, maintaining BP during the hypothermia and rewarming period is essential to patient care. Moreover, arrhythmias are common during the induction and rewarming phases of hypothermia, and their incidence can be exacerbated by hypothermia-induced changes in serum electrolytes including hypo/hyperkalemia, hypomagnesemia, and hypocalcemia. For all of these side effects, clinicians must weigh the potential benefit to risk ratio of hypothermia and systems should be in place to monitor and manage the complications.
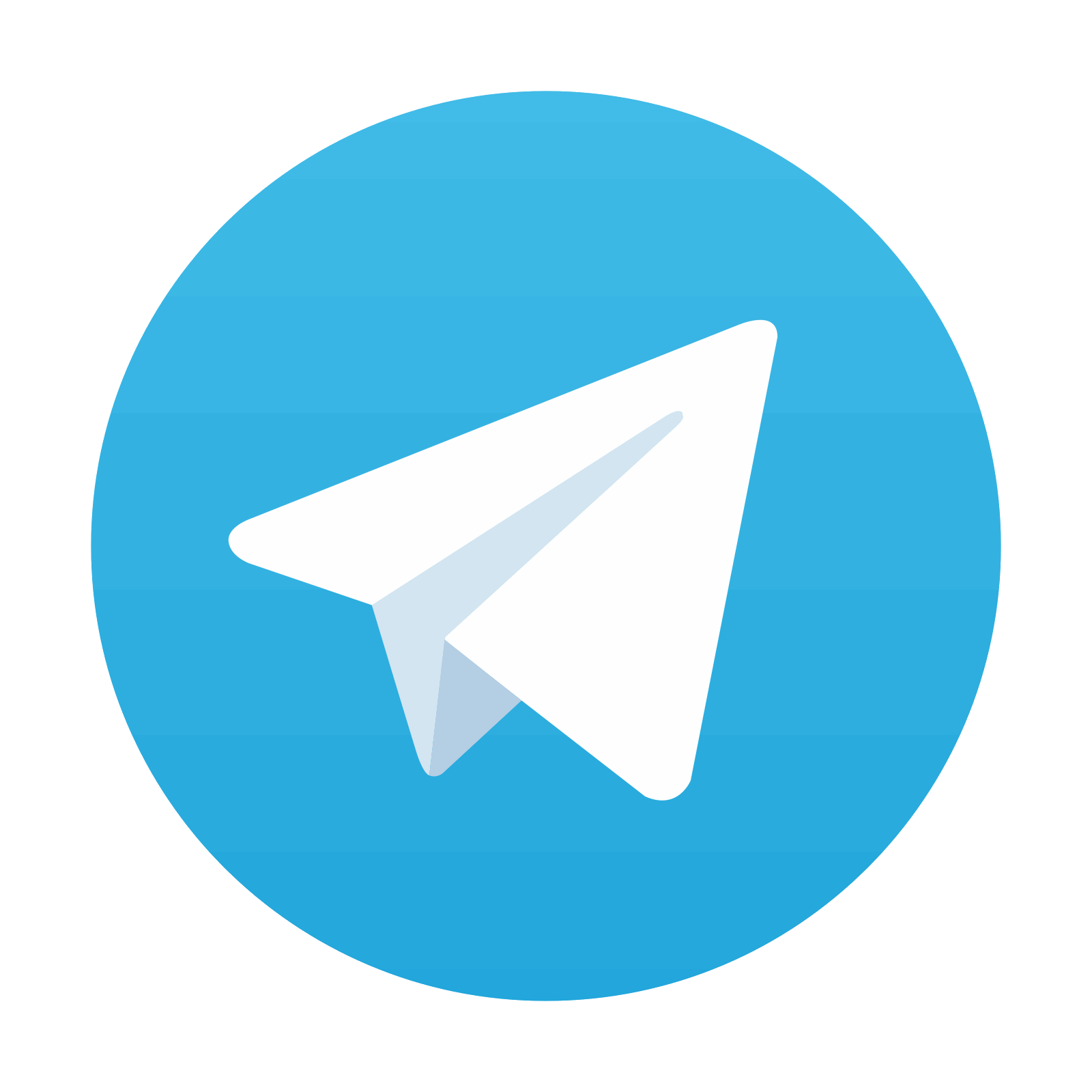
Stay updated, free articles. Join our Telegram channel

Full access? Get Clinical Tree
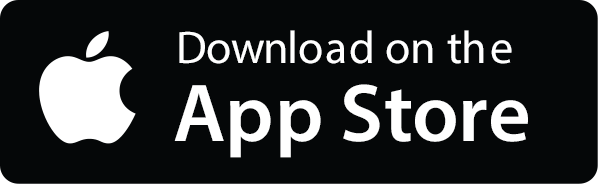
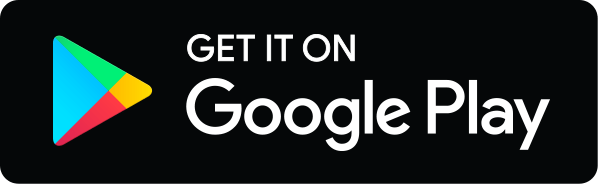