Fig. 1
Molecular, cellular and pathological as well as neuroregeneration events that follow the initial interaction of M. leprae with Schwann cell-axon units within the adult peripheral nerves. These events together with a cascade of complex inflammatory responses over a long incubation period subsequently cause neurodegeneration that manifests clinically in leprosy as the loss of sensory and motor neuronal functions
3.2 Why Do Leprosy Bacteria Target the Glial Cells of the PNS?
Schwann cells, the glial cells of PNS, which derive from neural crest precursors, comprise myelin-forming and non-myelin-forming phenotypes (Le Douarin and Dupin 2003; Jessen and Mirsky 2005). Although they can be considered as an example of sophistication in cell differentiation they show remarkable plasticity, which contributes to the regeneration capacity of adult PNS even after severe injury (Radtke and Vogt 2009). By selecting Schwann cells ML have acquired several advantages (Cole et al. 2001; Rambukkana 2010). Recent studies have suggested that ML use the regenerative properties of PNS for the expansion of their bacterial niche (Rambukkana et al. 2002; Tapinos et al. 2006; Rambukkana 2010). Regeneration of damaged peripheral nerves has been documented in patients with advanced leprosy and this may also be associated with a part of ML efforts to maintain an intracellular niche during human infection (Miko et al. 1993). These and accumulating new findings led to the proposition that the high plasticity of non-myelinating Schwann cells that could easily be manipulated could be one of the reasons why ML have chosen Schwann cells as the primary residence for colonization and subsequent dissemination (Masaki et al. 2013) (Fig. 1). Indeed, high plasticity generally increases the susceptibility of somatic cells for manipulations when exposed to appropriate stimuli or microenvironment (Theise and Wilmut 2003). Likewise, during infection, bacterial efforts needed to manipulate host cells with high plasticity are relatively less demanding as compared to highly differentiated cells like myelin-forming Schwann cells. Indeed, myelin-forming Schwann cells have been shown to be much less preferred by ML in vitro and in vivo models as well as in leprosy patients (Rambukkana et al. 2002). Additionally, Schwann cells also serve as safe haven for ML, since they protect the organism from host immune assault (Stoner 1979; Job 1989). Thus, initial ML propagation in Schwann cells is likely to occur without hindrance from immune cells. Such favorable conditions, which are assisted with the non-toxic, non-cytopathic, and non-apoptotic nature of ML, permit bacterial residence within Schwann cells for a long period of time, a favorable condition that fits to extremely passive obligate ML life style (Lahiri et al. 2010; Rambukkana 2010).
3.3 Schwann Cell Infection, Demyelination and Regeneration
Under physiological and pathological conditions Schwann cells take decision to remain quiescent or to differentiate, to undergo programmed cell death or to proliferate and de-differentiate. ML appear to have evolved mechanisms to induce cell proliferation and de-differentiation after having caused demyelination of myelin-forming Schwann cells and invaded non-myelin-forming Schwann cells (Rambukkana 2010). Both demyelination and the invaded ML in non-myelin-forming Schwann cells in large numbers have been documented in lepromatous patients (Shetty et al. 1980, 1988). These findings suggest that ML multiply as long as Schwann cells, which lack anti-microbicidal machinery, can tolerate the bacterial load and then release bacteria and infect more Schwann cells (Rambukkana et al. 2002; Rambukkana 2004) (Fig. 1). These conditions generated by ML infection are somewhat similar to peripheral nerve injury in vivo where Schwann cells rapidly proliferate, an important event for the promotion of nerve regeneration (Fawcett and Keynes 1990). Once colonized, intracellular ML repress Schwann cell differentiation program and instead maintain infected Schwann cells in a de-differentiated stage that is favorable for intracellular bacterial propagation (Masaki et al. 2013). Although the latter conditions have significant advantage for the establishment of infection within the PNS, maintaining Schwann cells in de-differentiated stage will cause adverse effects on the functions of peripheral nerves. This could be an alternative mechanism for nerve dysfunctions after long incubation periods during human infections (Fawcett and Keynes 1990) (Fig. 1).
It is interesting to note that, in addition to neurodegeneration, regeneration of damaged axons has also been documented in patients with advanced leprosy, and this may also partly be associated with bacterial efforts to maintain an intracellular niche during human infections (Miko et al. 1993). Such studies on patients provide evidence that ML may assist the nerve regeneration process in order to maintain Schwann cell niche in active stage so that bacterial survival can be secured despite the immune responses. Using mouse models Masaki et al. (2013) have recently demonstrated that ML use the plasticity of adult Schwann cells to reprogram infected cells and thereby convert the infected cells to progenitor/stem cell-like cells which favour bacterial dissemination following colonization in Schwann cells. The capacity of these reprogrammed cells to secrete chemokines that recruit macrophages and transfer infection further assists the development of a secondary bacterial niche but also ML dissemination by granuloma formation, a pathogenic hallmark of human leprosy (Cosma et al. 2003). This new knowledge suggests that PNS not only serves as a primary niche for bacterial propagation but also promotes infection to other parts of the body particularly to skeletal muscles, bones and smooth muscles, which are known to harbour ML in leprosy patients. These accumulating evidences led us to the proposition that plasticity of non-myelinating Schwann cells could be one of the reasons why ML have chosen them as the primary non-immune residence for colonization, which subsequently lead to the peripheral nerve damage.
3.4 Contribution of Schwann Cell Infection to Early Neurodegeneration
A growing body of evidence now suggests that the lack of interaction between glial cells and neurons could be the cause for disease pathogenesis in the nervous system. Recent studies have shown that when glial-neuronal interaction is perturbed, neuronal functions are also abrogated. For example, knockdown of neuregulin 1, a major axonal ligand that communicates with Schwann cells via glial receptors ErbB3/2 complex, causes several abnormalities in neuronal functions (Nave and Salzer 2006). Interestingly, ML bind to neuregulin receptors and induce signaling that eventually causes demyelination (Tapinos et al. 2006). These findings suggest that ML interference with glial-neuronal communication could initiate neuronal dysfunctions. Furthermore, such non-immune mediated myelin damage could rapidly be repaired, as remyelination is known to occur spontaneously in adult peripheral nerve in response to injury (Fawcett and Keynes 1990). Nevertheless, ML-induced nerve injury conditions could be progressive during the course of an extremely slow infection. This continues to develop with Schwann cell propagation and bacterial replication that subsequently release immune factors and recruit inflammatory cells to the site of infected peripheral nerves (Masaki et al. 2013).
3.5 Activation of Signaling Pathways in Infected Schwann Cells
As noted above, homeostasis of glial cells and neurons and their reciprocal interactions play a significant role in maintaining a healthy PNS. Leprosy bacteria appear to take advantage of this intimate cell-cell communication system by infecting Schwann cells and altering or subverting critical Schwann cell functions (Tapinos et al. 2006). Induction of signaling in response to ML is one of the key events that occur very early after infection, when ML binds to ErbB2 receptor tyrosine kinase on Schwann cells (Tapinos et al. 2006). This leads to the sequential activation of Raf, Mek1/2 and Erk 1/2/MAPK signaling pathways in myelinated Schwann cells and is sufficient to induce demyelination (Tapinos et al. 2006). Such receptor-mediated MAP kinase signaling was the first to give evidence that the activation of Erk1/2 alone is sufficient to induce demyelination without immune responses or other lesions. Along with demyelinating changes, intracellular ML also can activate Erk1/2 signaling via PKC and LCK signaling without using canonical receptor-mediated activation, and induce Schwann cell proliferation (Rambukkana 2010). Thus, infection of Schwann cells can start not only demyelination but also cell proliferation (Fig. 1).
Interestingly, the lesson learned from leprosy bacteria infections on the activation of Schwann cell Erk1/2 signaling in demyelination has recently been reiterated in inducible transgenic mouse model. In this model, sustained activation of inducible Raf-kinase transgene that activates downstream Erk1/2 in myelinated Schwann cells is sufficient to cause demyelination (Napoli et al. 2012). Like in ML infection, transgene-induced Erk1/2 signal activation and subsequent demyelination also recruit inflammatory cells, which are usually required for regeneration following demyelination and injury. Thus, it is likely that initial ML infection in leprosy patients may also undergo similar series of events following ML interaction with Schwann cell-axon units—activation of Erk1/2 signaling, demyelination and subsequent inflammatory response. This recruits macrophages which in turn aggravate inflammatory conditions that eventually abolish the nerve functions. Nerve dysfunction due to inflammation at this stage seems to manifest clinically as sensory or sensorimotor loss (Job 1989).
3.6 Association of Inflammation with Neurodegeneration in Human Leprosy
Nerve involvement in leprosy patients can be identified following nerve function loss due to strong inflammatory responses, in the form of sensory or motor neuron damage (Minauchi and Igata 1987; Sabin et al. 1993). From this stage, the disease process is progressive and could lead to deformities and disabilities unless the patients are treated to reduce the bacterial load and inflammatory conditions. Although bacterial cure can be achieved by successful multidrug antibiotic therapy, neurological injury may continue to occur in these patients due to strong inflammation. This includes the delayed type hypersensitivity reactions producing acute inflammation known as type-1 reactions, which are episodes with enhanced T cell and macrophage responses in peripheral nerves leading to their destruction. However, we know little about the immune mechanisms involved in nerve damage in human leprosy.
4 Clinical Manifestations
4.1 Phenotypes, Classification
In humans, the estimated incubation period from initial infection to clinical manifestation of nerve injury is likely to be 5–7 years, although it could go up to 30–40 years, but no proper documented studies are available on this. The onset of nerve damage is insidious. However, if untreated, the neurological damage could be progressive, as in other neurodegenerative diseases, and result in permanent damage to the nerves and subsequent impairment in limbs and eyes. The bacilli favor the cooler areas of the body such as chin, malar areas of face, earlobes, buttocks, knees, and distal extremities. Disease phenotype of leprosy is thought to depend on host immune reactions to the bacteria, especially the T cell immunity (Ooi and Srinivasan 2004). When the host responds to the bacterial infection with little or no cellular immunity as in the lepromatous form of leprosy with high bacterial load, numerous skin lesions appear, and peripheral nerves are diffusely affected slowly and progressively. When the host responds with strong cellular immunity, as in the tuberculoid form of leprosy with low or no bacteria. The nerve is more markedly damaged due to aggressive immune attack. Thus, leprosy is classified according to the number of skin lesions and the number of bacilli found on slit-skin smear examination (Brown et al. 1996a, b; Chandi and Chacko 1986; Cole et al. 2001; Coruh and McDougall 1979). Paucibacillary disease indeterminate [tuberculoid tuberculoid (TT) and borderline tuberculoid (BT)] is defined as fewer than six skin lesions with no bacilli on slit-skin smear testing. Multibacillary disease [borderline borderline (BB), borderline lepromatous (BL) and lepromatous leprosy (LL)] is characterized by six or more lesions with or without positive skin smear results, mostly with high bacterial content.
4.2 Neuropathies and Skin Lesions
4.2.1 Diverse Clinical Signs and Symptoms of Neuropathies and Skin Lesions Across the Leprosy Spectrum
Regardless of host immune response, variability of nerve lesions occurs across the leprosy spectrum. Tuberculoid leprosy, particularly the TT type, is characterized by a well-defined uniformly circular or oval erythematous/hypo-pigmented skin plaque with maximal induration of the margins sloping towards the center. The surface is bald, dry and scaly, and completely anesthetic. In the vicinity of the skin lesions, thickened nerve is sometimes palpable. The involved dermal nerves are infiltrated with granulomas composed of epithelioid cells and lymphocytes. In peripheral neuropathy, a single nerve is enlarged, which results in a mononeuropathy such as wrist drop, foot drop or claw hand (Minauchi and Igata 1987; Ooi and Srinivasan 2004; Agrawal et al. 2005). Mononeuropathy multiplex is also seen. Commonly involved are greater auricular, radial cutaneous, ulnar, median, common peroneal, posterior tibial, and facial nerves (Minauchi and Igata 1987; Ooi and Srinivasan 2004; Agrawal et al. 2005).
Patients with the lepromatous form with high bacterial load, particularly LL patients, present with generalized disease with multi-system involvement, sparing only the central nervous system. Most strikingly involved tissues in addition to nerves are the skin, mucous membrane and reticuloendothelial systems (Sehgal 1994). Skin lesions are multiple, bilateral, and symmetric. Typically, the lesions are hypopigmented, shiny with ill-defined margins and merges imperceptibly with surrounding skin. Frequently involved are eye-brows, nose and lips, along with flattening of the bridge of the nose resulting in classical “leonine facies”. Nerve involvement results in progressive bilateral symmetrical cutaneous sensory loss, and distal weakness, together with patch anesthesia and mononeuropathies (Minauchi and Igata 1987). The nerve trunks are bilaterally and symmetrically thickened and tender (Ridley and Jopling 1966; Sehgal 1994). Enlarged nerve may be functioning well, suggesting that bacilli invasion into nerves itself does not cause acute nerve damage, but it is the inflammatory reactions that are responsible for the nerve damage.
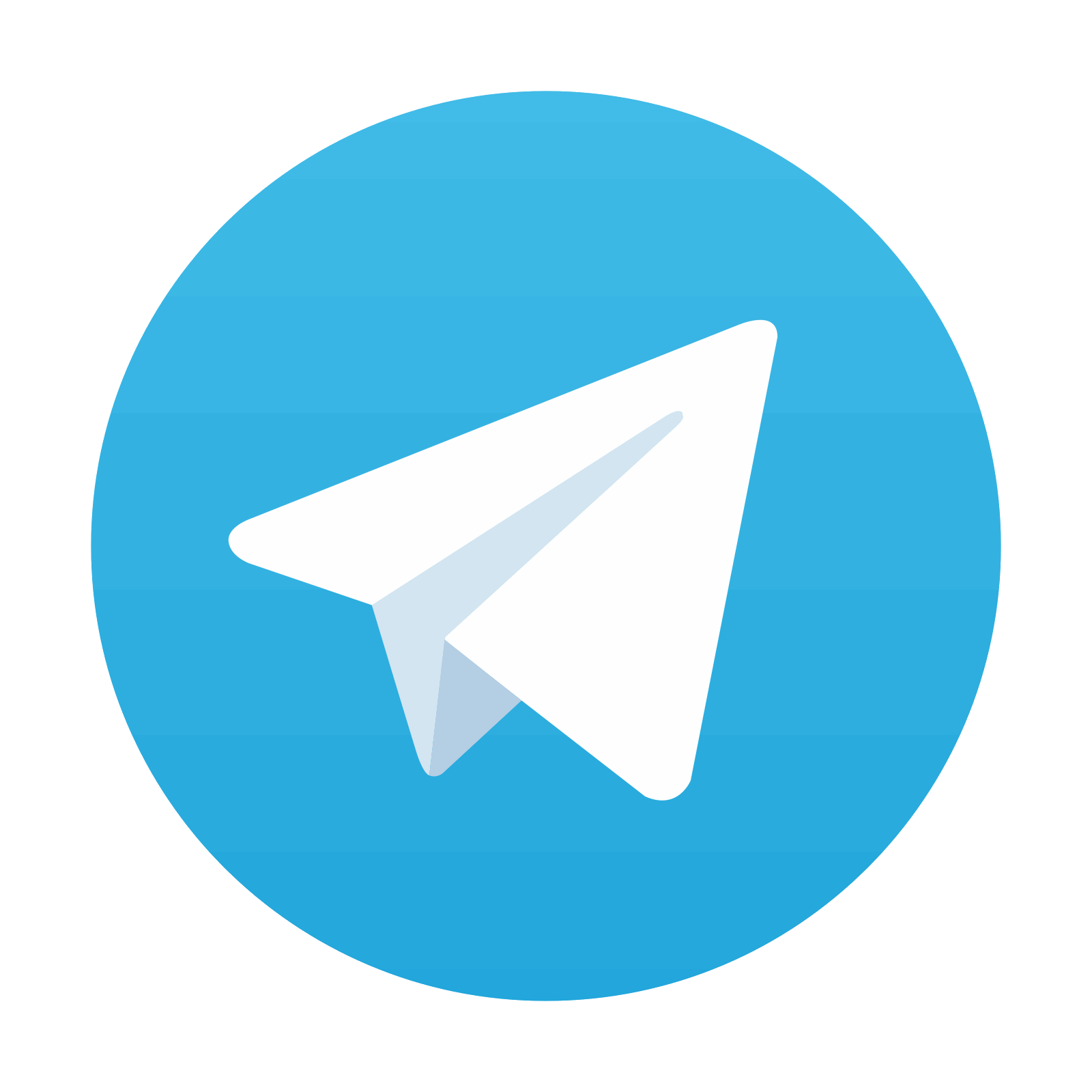
Stay updated, free articles. Join our Telegram channel

Full access? Get Clinical Tree
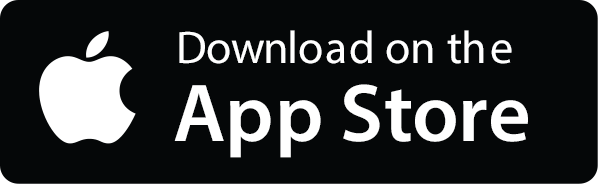
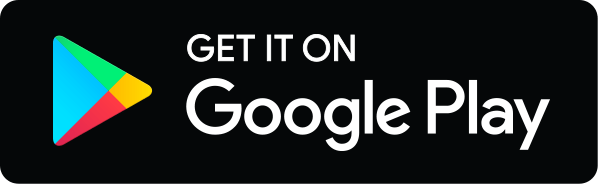