15 Intracavitary Radiation for Cystic Craniopharyngiomas Despite the major advances of skull base microsurgical techniques, as well as expanded endoscopic techniques, craniopharyngiomas continue to be difficult to remove totally with acceptable morbidity. Ideally, surgical techniques result in total removal, maintenance of visual function, sparing of endocrinologic loss, and absence of delayed recurrence. Even when feasible, radical resection continues to be associated with unsatisfactory long-term outcomes, often because of residual neurobehavioral disorders, cognitive impairment, the long-term need for replacement hormone therapy, and hypothalamic obesity. Realizing the morbidity of surgical techniques for craniopharyngioma in the early 1950s, both Leksell et al and Wycis et al proposed the implantation of a β-emitting isotope into cystic craniopharyngiomas.1,2 Isotope implantation was designed to lead to slow involution of the cyst wall by delivery of a tumoricidal dose to the thin epithelial layer of the cyst. Since that time, selected pioneers in Europe have continued to apply the instillation of radioactive isotopes into craniopharyngioma cysts. Efforts in Europe, especially in Stockholm under the direction of Erik-Olof Backlund, demonstrated that intracavitary irradiation provides an effective and safe technique in the management of cystic craniopharyngiomas.3–5 Leksell and Backlund used both radioactive phosphorus 32 (in colloidal chromic solution) and yttrium 90.1,3 In the United States, the unavailability of 90Y has led to the primary use of 32P. Stereotactic insertion of the isotope with fine-needle technique is critical. The treatment of cystic brain tumors using radioactive isotopes is based on the concept that continued cyst enlargement is the result of secretion from the thin epithelial cyst layer. Most craniopharyngiomas in fact have solid components, and subsequently develop cystic changes over the course of time. The cyst gradually enlarges like a bubble blown off a piece of bubblegum. By the time of clinical presentation, the cyst may range in volumes as small as 1 mL to as large as 126 mL. Depending upon its location and volume, the enlarging cyst results in endocrine loss, progressive visual dysfunction, and neurocognitive and intellectual deficits. Intracavitary irradiation uses stereotactic precision to puncture the cyst and instill the isotope volume designed to deliver 180 to 250 Gy of radiation over five half-lives of the isotope.6–8 Because the half-life of 32P is 14 days, it takes ~70 days to deliver the full isotope effect. As a pure β emitter, the falloff of radiation from the decay of the isotope is very steep. The radiation dose falls off in accordance with the inverse square law, which refers to the fact that reduction in activity is proportional to 1 divided by the square of the distance from the source. The half-value tissue penetrance of 32P is 0.9 mm, indicating that most of the radiation effect by far is delivered within several millimeters of the cyst wall. Implantation of the isotope leads to a “coating out” of the internal cyst wall and slow delivery of the radiobiological effect of the 32P decay. This dose range appears to be sufficient to lead to cyst involution in the vast majority of cases. Long-term outcome studies from multiple centers are now available to demonstrate the benefit of intracavitary management of cystic craniopharyngiomas. Because current magnetic resonance imaging (MRI) confirms that most patients have solid and cystic tumors, many will require multimodality management. Successful strategies require cyst control, solid tumor management by microsurgery or endoscopic surgery, and stereotactic radiosurgery.9,10 A combination of such techniques may be best to achieve the best outcomes for patients with these strategically located tumors capable of producing visual, endocrinologic, and cognitive dysfunction. After the primary author returned from his Van Wagenen–sponsored fellowship at the Karolinska Institute in 1981, an initial, cautious trial of the role of intracavitary radiosurgery was pursued at the University of Pittsburgh Center for Image-Guided Neurosurgery. In the 28-year interval from 1980 to 2003, 61 patients with craniopharyngioma (33 males and 28 females) underwent intracavitary radiation after the initial diagnosis or recognition of a recurrent craniopharyngioma.6 All patients had their tumor confirmed initially (before 1991) with computed tomography (CT) and with magnetic resonance imaging (MRI) afterward. The ages of the patients in this initial experience ranged from 4 to 74 years (mean, 28 years), and the calculated cyst volumes ranged from 1.8 to 126 mL. All patients underwent preoperative endocrinologic assessment, visual acuity and formal visual field examinations, and overall neurologic assessment. The majority of the patients had undergone multiple prior surgical procedures, including one or more craniotomies and transsphenoidal resection, and seven patients had had ventriculoperitoneal shunts placed for hydrocephalus management. Fractionated external beam radiation therapy, the role of which has declined over the last 10 years, had been previously administered to 11 patients. We select patients with monocystic or multicystic craniopharyngiomas. After review of the preoperative CT scan or MRI, we can estimate the cyst volume for the nuclear pharmacist. Using a simple calculation, π/6× (X × Y × Z), we determine the volume of an oblate spheroid. X, Y, and Z are the diameters of the lesion in the three cardinal planes; × denotes multiplication. The nuclear pharmacist orders colloidal chromic phosphate 32P in a stock solution dose. This is assayed on the day of delivery and reassayed on the day of the operation. If the cyst volume is less than 20 cm3, the suspension is usually diluted with a 30% glucose solution to facilitate delivery of the isotope. All patients are brought to the operating room for the surgical procedure, which is done under mild conscious sedation. The Leksell Model G stereotactic head frame (Elekta Instruments, Stockholm, Sweden) is attached to the patient’s head with the use of local anesthesia. Highresolution axial CT (2.5-mm configured axial slices, 512 × 512 matrix) is performed with a dedicated intraoperative CT scanner in our image-guided operating room suite. The cyst area in square centimeters is calculated on each slice by tracing the cyst volume after intravenous iodinated contrast is administered. The sum of each slice is then multiplied by the slice thickness (2.5 mm) to calculate the cystic tumor volume in cubic centimeters. The calculated cyst volume is called to the nuclear pharmacist, who prepares the isotope and calculates the volume of P32 necessary to provide a dose of 180 to 250 Gy over five half-lives (~70 days). The patient is advanced through the opening of the CT scanner, and the head is prepared with alcohol and draped. A frontal coronal suture twist drill craniostomy is performed with the arc attached at the chosen X, Y, and Z coordinates for a central puncture of the cyst. The trajectory is plotted in advance to reduce the number of pial transgressions and, when feasible, the ventricular entry. The target point is usually 7 to 10 mm inferior to the dorsal margin of the cyst, as it is sometimes necessary to puncture the cyst sharply and depress the cyst briefly before the 0.9-mmouter-diameter sharp-tipped needle is inserted into the center of the cyst (Fig. 15.1). The 0.9-mm sharp-tipped needle is passed through a 15-cm stabilizing cannula after closed-skull percutaneous twist drill craniostomy and puncture of the dura. Once the target is reached, the stylet is removed and a three-way stopcock is attached (Fig. 15.2). Using a tuberculin syringe, we generally withdraw 1 mL of cyst fluid. A neuropathologist examines the fluid with polarized light microscopy to detect cholesterol crystals. Two tuberculin syringes are attached to the three-way stopcock (Fig. 15.3). The nuclear pharmacist and the radiation safety officer deliver the calculated volume of 32P, usually in the range of 0.6 to 0.8 mL. The isotope contained in the syringe is injected through the stopcock into the cyst, and a barbotage method is used to ensure mixture. After this, the residual isotope is flushed out of the needle with 0.2 mL of sterile saline. The goal is to restore the cyst volume to that of the original calculation. Collapse of the cyst induces a potential for internal wrinkling, which may suboptimally cause areas of the wrinkled cyst wall to receive an insufficient therapeutic dose of the β-emitting isotope. After the final isotope injection, the residual activity of 32P (as well as all instruments and supplies used) is assayed by the nuclear pharmacist. Any activity is subtracted from the dose administered to the patient. Using our intraoperative CT, we do an immediate postoperative head scan and usually observe a small air bubble at the anterior margin of the cyst (the patients are supine on the CT scanner table). All patients are kept in the hospital for a one-night stay. In our experience, the mean radiation dose delivered is 224 Gy (range, 189–250 Gy to the cyst wall). The median cyst volume is 7.0 cm3 (range, 2.0–20 cm3). Clinical follow-up and imaging are obtained from each patient and the referring physicians. When possible, patients are contacted by telephone to determine their long-term outcomes. CT or MRI studies are normally performed at 3 months after the procedure and at quarterly intervals during the first year. In the next 5 years, they are requested annually. Formal neuro-ophthalmologic evaluations, as well as periodic endocrinologic assessments, are also performed. In this review of 61 patients treated before 2008, 12 of the 61 patients eventually died as a result of tumor progression and eight were lost to follow-up (Table 15.1). The mean follow-up interval after diagnosis was 82 ± 14 months (47 ± 8 months after intracavitary irradiation). Actuarial survival rates after diagnosis were 90% at 5 years and 80% at 10 years. We noted a trend toward increased survival in children younger than 16 years of age. Among 53 patients who had long-term follow-up, treatment with 32P intracavitary irradiation was considered to have failed in eight. The results of cyst response after intracavitary irradiation are shown in Table 15.2. In 15 patients with predominantly mono- or multicystic tumors, 80% of the tumors became smaller after treatment. In 37 patients with mixed solid and cystic tumors, 65% of the tumors became smaller after treatment. Cystic control was not associated with the patient’s sex or age, tumor type (ie, mono- or multicystic tumor, mixed tumor with solid components), primary versus adjuvant treatment, cyst volume, radiation dose, preoperative visual acuity or field, or preoperative pituitary function. During follow-up, six patients developed new cystic tumors. Illustrative cases of tumor reduction are shown (Figs. 15.4 and 15.5). Among the 53 patients with long-term follow-up, treatment with intracavitary radiation failed in eight. Seventeen patients (32%) required additional cyst aspiration after intracavitary irradiation because of persistent symptoms related to larger cyst volume (Fig. 15.6). The median interval between intracavitary irradiation and additional cyst aspiration was 0.5 months (range, 0.2–18 months). Early cyst decompression is especially important in patients with progressive optic neuropathy. Although spontaneous cyst regression is the rule, it may take several months. Early cyst aspiration at 2 to 6 weeks after implantation may be necessary to achieve the best early response. In such patients, the procedure is repeated with stereotactic technique. After cyst puncture with fine-needle technique, we gently aspirate approximately one-half of the calculated volume. We assay the fluid to make sure that no significant isotope has been removed. Even if planned reaspiration is performed within 2 weeks of the 32P instillation, a relatively small percentage of isotope can be recovered as it presumably adheres to the internal cyst wall. Among our patients who underwent primary management with intracavitary irradiation for a monocystic craniopharyngioma, 48% had improved visual acuity and 52% had improved visual fields. Among patients who were treated as part of a multimodality strategy (intracavitary irradiation was an adjuvant strategy), 28% had improved visual acuity and 25% had improved visual fields (Tables 15.3 and 15.4). Three patients developed the new onset of visual abnormalities despite documented tumor regression. One of these patients underwent stereotactic radiosurgery to the solid component of the residual tumor. In these patients, visual dysfunction was thought to be related to a potentially adverse effect of 32P irradiation. Among patients treated with primary monocystic intracavitary irradiation, 52% continue to have normal pituitary function, but 48% have had one or more axes of pituitary function loss. In the adjuvant treatment group, only 19% of patients had preserved endocrine function before the procedure, and 81% had documented loss of pituitary function in one or more axes. Three patients developed pituitary dysfunction as a consequence of cystic recurrence. Four patients developed diabetes insipidus after treatment with 32P intracavitary radiation. In our total 28-year experience, only one patient developed an early postoperative complication. A patient with a mixed multicystic and solid craniopharyngioma underwent multiple endoscopic skull base procedures but developed a tumor cyst of the third ventricle. Before the planned procedure, the cyst spontaneously ruptured into the ventricle and decompressed. However, within a matter of months, the cyst reformed, and intracavitary stereotactic irradiation was performed. Several days after this procedure, the patient was readmitted with headache, low-grade fever, stiff neck, and cerebrospinal fluid (CSF) pleocytosis. No radioactivity was confirmed in the CSF. The patient had an aseptic meningitic reaction that resolved with corticosteroids. This patient has undergone additional surgical procedures followed by gamma knife stereotactic radiosurgery.9,10 Patients who are eligible for radical gross total resection of a craniopharyngioma may have the best long-term response in terms of tumor control.11–19 However, such patients often pay a significant price that may include endocrinologic, cognitive, behavioral, and visual difficulties.20,21 Craniopharyngiomas remain one of the most difficult tumors for neurosurgeons to manage. For patients, the side effects of surgery are often significant. Because of the attachment of these tumors to critical neurovascular, endocrine, and visual structures, patients are often unable to undergo radical resection with an acceptable morbidity. For most of these patients, multimodality management, administered over the course of years, is frequently necessary.10,22 The options for craniopharyngioma management include fractionated radiation therapy,22 cyst management with intracavitary irradiation, intracystic chemotherapy with bleomycin,23–28 endoscopic resection, and, more recently, phase I trials with interferon. Because craniopharyngiomas frequently are detected in childhood, we generally try to withhold conventional fractionated radiation therapy, even when it can be administered with modern techniques of image-guided radiation therapy.22 Intracavitary irradiation with 32P is an additional tool for treating patients with cystic craniopharyngiomas. Using modern imaging techniques, we find that most patients have a solid, small tumor component with one or more cysts arising from it. Intracavitary irradiation provides symptomatic cyst control in a high percentage of patients, but it does not solve the ultimate progression of the solid component of the tumor. It also does not prevent the formation of new tumor cysts. In such patients, repeat surgery or stereotactic radiosurgery may be necessary.9,10 The continuing goals for the management of craniopharyngioma include preservation of visual and endocrine function and reduction in the risk for delayed cognitive dysfunction. Intracavitary irradiation with 32P has more than a 60-year history and remains a valuable tool. The use of this tool requires training and expertise, as emphasized by Leksell et al1 and Backlund.3 Access to pure β emitters is critical to the successful continued management of tumor cysts when they develop. The need for delayed stereotactic aspiration with precise frame-based imaging guidance, especially when tumors are wrapped around critical vascular and optic nerve structures, is critical. Stereotactic 32P intracavitary irradiation has a relatively low risk. It is an effective strategy for the primary treatment of monocystic craniopharyngiomas and for the adjuvant management of the cystic component of multicystic or mixed solid craniopharyngiomas. Craniopharyngiomas are one of the most difficult tumors that neurosurgeons encounter. Efforts to minimize morbidity from the tumor or from its treatment can reduce the significant dysfunction that patients with cranopharyngioma experience. Minimally invasive treatment strategies that pursue the goals of cognitive, endocrine, and vision preservation remain critical to the long-term successful management of these patients.
Rationale for Intracavitary Irradiation
Initial Experience
Stereotactic Technique for Intracavitary Irradiation
Follow-up
Results
Additional Treatment
Clinical Response
Endocrinologic Response
Other Complications
Discussion
Conclusion
References
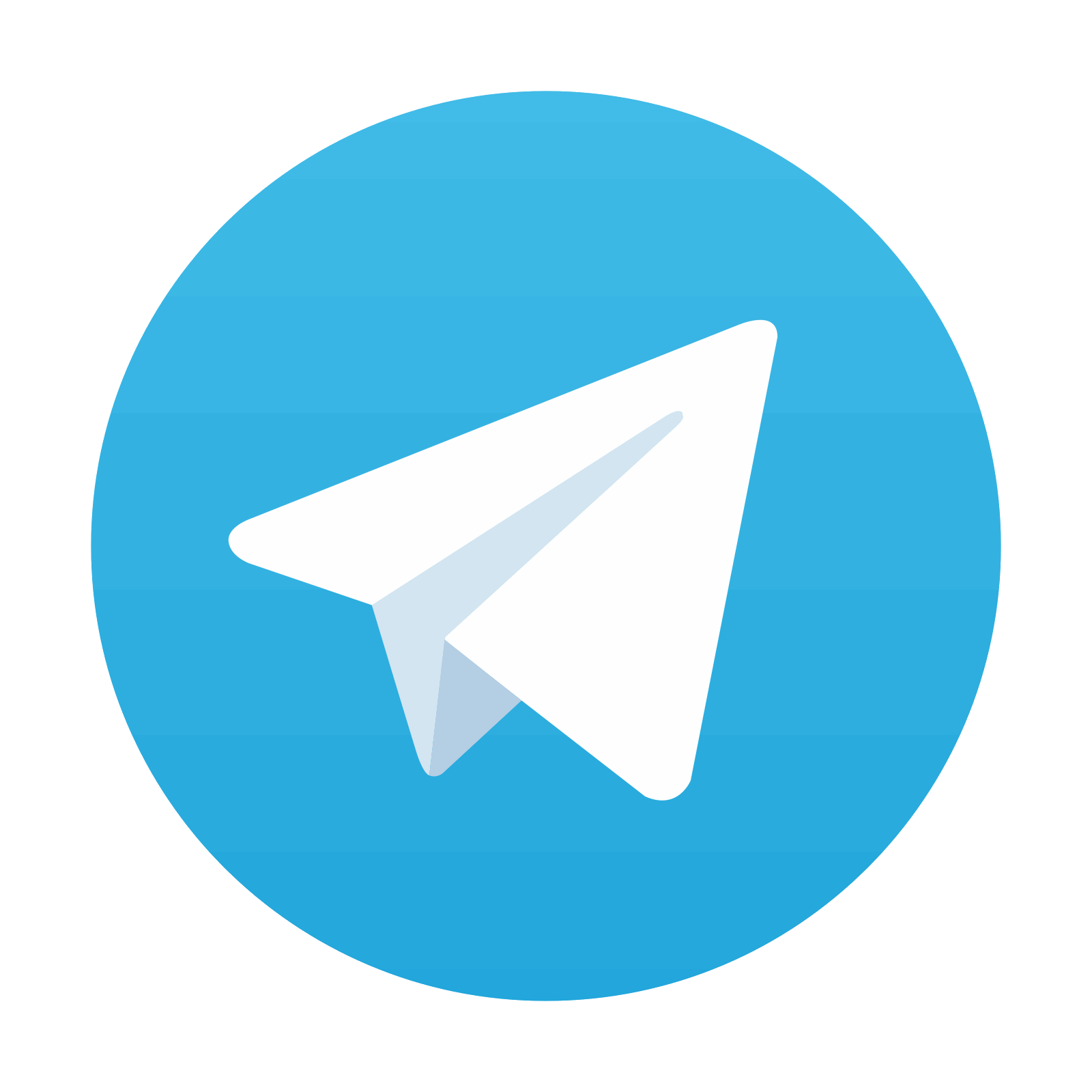
Stay updated, free articles. Join our Telegram channel

Full access? Get Clinical Tree
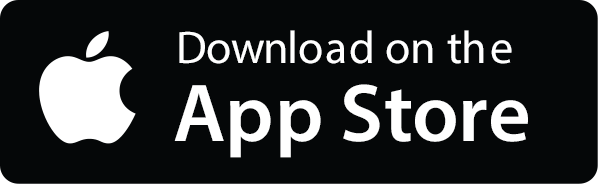
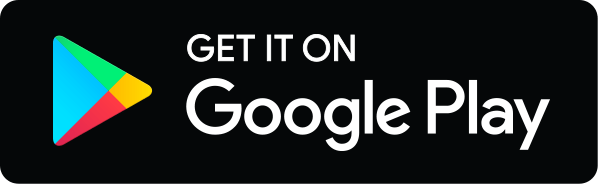