Intracranial Electrodes
Susan S. Spencer
Michael R. Sperling
D. Alan Shewmon
Philippe Kahane
Introduction
Reports and experience worldwide have shown that resection of the epileptogenic area of brain in uncontrolled epilepsy patients with stereotyped partial seizures can cure the epilepsy, or at least prevent recurrent seizures.30,57,81 The corollary of this statement is that if the surgery fails, the area removed was incorrect or incompletely resected or was only one of several cerebral regions responsible for seizures. Accordingly, accurate definition of the location and limits of this epileptogenic area has become a major preoccupation among epileptologists.
The first need is to define epileptogenic area, a term synonymous with epileptogenic region or epileptogenic zone. This is defined as the area of brain necessary to generate seizure activity,31 resection of which (or inactivation or complete disconnection of which) will result in cessation of seizures. We seek to differentiate this epileptogenic zone from the irritative zone (of interictal spiking), the ictal onset zone (where the electroencephalogram [EEG] shows spontaneous seizures to arise), the symptomatogenic zone (activation of which produces initial ictal symptoms or signs), the zone of functional deficit (with evidence of reduced or abnormal metabolism, blood flow, and cognitive ability), and the epileptogenic lesion (demonstrated by structural imaging).74 Although those areas usually overlap, they do not precisely coincide with the epileptogenic zone and, at times, appear to diverge. We might eventually develop techniques that allow visualization of the epileptogenic zone in other ways, but currently the demonstration of electrographic seizure onset offers the most logical means of verifying its approximate location,36 except in childhood catastrophic epilepsy, in which the ictal onset zone is typically reported to be generalized even if the causative, epileptogenic zone is localized.72 (An exception to this is the occasional case of childhood catastrophic epilepsy in which intracranial recording has demonstrated intrinsic epileptogenicity of a lesion, such as hypothalamic hamartoma.)48 Because the latter cases are not likely to be studied with intracranial electrodes, this chapter concentrates almost exclusively on the evaluation of partial seizure disorders.
The electrographic delineation of the epileptogenic zone can be supported by delineation of the irritative zone, the symptomatogenic zone, the area of focal functional deficit, and the epileptogenic lesion such that the complementary nature of the respective studies allows one to infer the limits of the epileptogenic zone from less precise electrographic information, a scenario most often found when an epileptogenic lesion is roughly colocalized with a surface EEG recording abnormality.32,33,83 In other situations, however, particularly in the absence of compelling data to characterize the epileptogenic lesion, irritative zone, and zone of functional deficit, the ability of the surface EEG to determine the ictal onset zone is suboptimal. For the purpose of designing an effective resective procedure for uncontrolled epilepsy, intracranial electrodes are then used to localize ictal onset as well as interictal spike distribution; this information is then used with the other data to infer the location of the epileptogenic area.
Intracranial EEG recording fills a specific need only in the context of a specific question. Extensive knowledge of seizure patterns, surface EEG expression of the ictal onset and irritative zones, and functional and structural abnormalities accompanying the epilepsy is necessary to properly design an intracranial EEG study. The nature of the procedure requires that its risk be sufficiently balanced by the possibility of useful gain in the localization, definition, and understanding of the uncontrolled epilepsy. In addition, the appropriate intracranial techniques must be used to answer the questions posed.
Intracranial EEG is indicated in the setting of uncontrolled epilepsy considered for resective surgical treatment when (a) there is likely to be a single region of seizure onset; (b) localization of this region is insufficiently precise or impossible based on the combination of ictal clinical manifestations, noninvasive EEG, and tests of focal functional deficit and structural abnormalities; and (c) the spectrum of findings in the individual allows a hypothesis about the suspected region or regions of seizure onset to be further investigated.
These considerations are important because intracranial recording has significant limitations, costs, and risks.91 Implanted electrodes necessarily record from a limited volume of cortex. They must therefore be placed appropriately to address the questions at hand. Furthermore, because chronic implantation carries the known risks of hemorrhage and infection and the unknown risks of cognitive effects and epileptogenic potential, it is desirable to restrict the number of implanted electrodes to as few as possible. This must be done without compromising the ability to detect the zone of seizure onset with sufficient precision to use in the determination of the epileptogenic area for subsequent surgery.
![]() FIGURE 1. Sagittal magnetic resonance imaging (MRI) view of temporal lobe depth electrodes inserted by a posterior approach, illustrating multiple contacts. |
Technological advances have altered the use of intracranial recording over the past decade in several ways. High-quality neuroimaging, with qualitative and quantitative magnetic resonance imaging (MRI) at the forefront, has improved our detection of macrostructural abnormalities in epilepsy, including certain gliomas, developmental abnormalities (cortical dysplasia, hamartomas), and restricted atrophic lesions such as hippocampal atrophy. As accumulated experience has supported significant correlations between excision of these lesions and seizure relief, finding evidence for a structural lesion has assumed increasing importance in the localization of the epileptogenic zone for epilepsy surgery.16,20,83,89 Now, the presence of certain “lesions” more often allows the less precise scalp EEG localization of the ictal onset zone to suffice because the additional information required to infer the localization of the epileptogenic area is provided by the MRI. Although scalp EEG localization to the region of the structural abnormality is still considered imperative to prove that the lesion is epileptogenic,
localization by interictal surface EEG without discordant information from ictal surface recording has become acceptable for this purpose at most centers. The opinion that only imaging might be sufficient to define a cortical region of epileptogenesis has been voiced, but some patients are known to have coincidental structural lesions irrelevant to the electrically abnormal area.34,84 In Yale’s recent experience, patients with discordant MRI and overall EEG localization represent approximately 10% of those with hippocampal atrophy, tumors, or developmental lesions.34 About half of these patients have had surgery based on ictal onset localization, with cure of seizures. This means that, although the MRI is highly sensitive in localizing epileptogenic structural lesions in uncontrolled epilepsy patients and these lesions are often where uncontrolled seizures begin, the structural abnormality does not necessarily indicate the epileptogenic zone.
localization by interictal surface EEG without discordant information from ictal surface recording has become acceptable for this purpose at most centers. The opinion that only imaging might be sufficient to define a cortical region of epileptogenesis has been voiced, but some patients are known to have coincidental structural lesions irrelevant to the electrically abnormal area.34,84 In Yale’s recent experience, patients with discordant MRI and overall EEG localization represent approximately 10% of those with hippocampal atrophy, tumors, or developmental lesions.34 About half of these patients have had surgery based on ictal onset localization, with cure of seizures. This means that, although the MRI is highly sensitive in localizing epileptogenic structural lesions in uncontrolled epilepsy patients and these lesions are often where uncontrolled seizures begin, the structural abnormality does not necessarily indicate the epileptogenic zone.
The increasing sophistication of imaging has also been responsible for generating a new population of patients for intracranial study who may not have been considered candidates before. These are patients with bilateral hippocampal atrophy or with so-called “dual pathology” such as a tumor or cortical developmental abnormality with hippocampal atrophy. In these situations, experts agree that intracranial recording is essential to determine which potentially epileptogenic lesion is indeed responsible for the uncontrolled seizure disorder.34 In one recent study, patients with bilateral hippocampal atrophy were found to be good candidates for cure of seizures after temporal lobe resection, with the side of resection based on intracranial ictal onset EEG localization and not on the severity of the atrophy.51 An MRI demonstration of dual pathology is also not always synonymous with dual epileptogenesis, although it might be.
Intracranial EEG has been in use since the 1950s. It was initially applied infrequently, mainly in patients with poorly localized surface EEG or evidence for bitemporal EEG abnormalities, who comprise 10% to 15% of surgical candidates. In the late 1960s and 1970s, some centers used intracranial EEG in all surgical candidates before resecting tissue for seizure control. This practice generated considerable historical and practical information but is no longer necessary. Now, some form of intracranial EEG investigation is performed on approximately 15% to 45% of adult patients at most centers that do epilepsy surgery. The proportion of patients considered for intracranial investigation depends on the referral population. In the pediatric population, both the need for and the practicality of intracranial recording diminish with decreasing age. Although invasive recordings can be done in infants,127 they are rarely necessary.23
Intracranial EEG is obtained with depth electrodes (Figs. 1 and 2), subdural strip electrodes (Figs. 3,4,5), subdural grid electrodes (Fig. 6), and/or epidural electrodes. They are multicontact, usually flexible electrodes that require an operative procedure for insertion in preselected locations based on prior evaluation. All of them can be used for chronic or acute recording over 1 to 4 weeks (rarely up to 8 weeks) for the purpose of localizing the epileptogenic zone by recording multiple spontaneous ictal events. They differ predominantly in the parts of brain that can be recorded. Only depth electrodes penetrate brain tissue directly. They are therefore best suited for recording from deep, buried structures such as amygdala and hippocampus (Figs. 1 and 2), planum temporalis, and insula. These electrodes are also well suited for recording from sulcal cortex, as well as near lesions in deep cerebral locations. Subdural electrodes are best suited for recording from the cortical surface, including dorsal, basal, and interhemispheric regions, and provide a method for safe, extensive cortical sampling. Subdural strip electrodes and depth electrodes can be inserted to record from multiple lobes and areas in both hemispheres. Subdural grid electrodes are restricted in insertion to previously defined zones.
![]() FIGURE 3. Sagittal magnetic resonance imaging (MRI) view of multiple subdural strips placed through a single burr hole; individual contacts can be identified. |
![]() FIGURE 5. Midline sagittal magnetic resonance imaging (MRI) view of interhemispheric placement of subdural strips. |
![]() FIGURE 6. Sagittal magnetic resonance imaging (MRI) view of subdural grid placed to record from temporal neocortex. |
The electrodes differ in the method of insertion, and with these differences come predictable differences in safety profiles. Depth electrodes are inserted with stereotactic MRI or computed tomographic (CT) guidance such that the trajectory, termination point, and location of each contact can be accurately predetermined.62,87,91 These electrodes are usually inserted bilaterally, and accurate symmetric placements can be routinely achieved, not an insignificant accomplishment when comparison of the homotopic areas for onset and propagation of seizure activity is paramount in interpretation (see Depth Electrodes, Interpretation further on in this chapter). The strategy for selection of target locations of depth electrode placement differs somewhat in North American and certain European centers. The differences are partly a result of the more frequent combination of depth with additional implanted (subdural) electrodes in North America, thus allowing a more restricted use of depth electrodes for only some of the regions to be studied. The approach at some European centers developed from the stereo-electroencephalography method of Talairach and Bancaud, and aims to simultaneously and three dimensionally display all areas potentially involved in the onset, propagation, and clinical manifestations of the ictus, using only depth electrodes.11,12,25,47,64,103,104 Both are based on hypotheses developed from noninvasive study. The European approach may incorporate more regions of suspected propagation and evolution, a consideration that influences the surgical approach of those centers. Subdural strip electrodes are inserted with the least precision because they are passed through burr holes and “slid” in the subdural space to the desired target (Figs. 3,4,5). Adhesions caused by prior surgery, infection, or injury often deflect the desired placement, and thus strict precision and homotopic sampling are rarely achieved. Depth and subdural electrodes can be removed at the bedside. Subdural grid electrodes require craniotomy for insertion and removal and can be placed accurately. Subdural grid electrodes are usually placed only when a reasonably well-defined epileptogenic zone is known, and they serve to aid in precisely defining the limits of resection. They provide optimal cortical coverage for functional mapping with stimulation if seizures begin near language, motor, or sensory cortex.
Increasingly, the intracranial EEG methods are combined to achieve optimal sampling of all suspicious areas for seizure onset. They can all be used at any age, although the range of indications tends to be more restricted in young children, especially infants. The specific indications, construction, insertion, interpretation, limitations, risks, cost, and accuracy of each of these methods are the subjects of the remainder of this chapter.
Indications for Intracranial Electrodes
Intracranial electrodes are indicated when it is reasonable to believe that a therapeutic resective procedure could be performed but the noninvasively obtained data are inadequate and more information is needed. Intracranial electrodes are then used to precisely identify the location of the ictal onset zone and can also delineate functionally important tissue that should be spared at the time of resection. Some protocols, particularly in certain European centers, also strive to delineate other regions of initial or early involvement that can be incorporated in designing the resection. Exact criteria for what constitutes sufficient concordant or discordant data vary somewhat from one epilepsy center to another.
Specific indications for intracranial electrodes in the evaluation of patients with refractory epilepsy include the following:
Insufficient concordance of noninvasively obtained data such that there remains uncertainty regarding the localization of the epileptogenic zone. Circumstances
leading to intracranial electrode use include the following:
An MRI showing an atrophic, developmental, or space-occupying lesion with nonlocalized interictal and ictal EEG.
A normal or nonlocalized MRI with insufficient evidence of localized functional deficit and localized abnormality of excitability in a single region.
Discordance of noninvasively obtained data, so that the existence of a single epileptogenic zone must be proven and better localized.
Occupation by the epileptogenic lesion of territory within or immediately adjacent to vital cortex (e.g., motor strip, language areas) so that invasive functional mapping must be performed before resection in conjunction with precise localization of the epileptogenic zone.
Although there are no set criteria that can be systematically used to decide whether intracranial recordings should be performed, these statements give broad guidelines. Factors such as the presence of a lesion and the type of epilepsy (temporal versus extratemporal) influence to various degrees the need for invasive recordings. In the great majority of cryptogenic cases, for instance, the use of intracerebral recordings remains essential.65 In temporal lobe epilepsy, although diagnosis of mesial temporal lobe seizures and the side of ictal onset is now possible in the majority of patients using noninvasive investigation, additional long-term monitoring with intracranial electrodes is appropriate when the side of mesial temporal ictal onset is unclear, if neocortical or extratemporal ictal onset is considered possible, or when electroclinical arguments suggest that the epileptogenic zone extends outside the boundaries of a more traditional approach to the mesial temporal lobe resection. The delineation of extratemporal epileptogenic networks is much more difficult than that of temporal lobe regions of seizure origin, so that many more patients with extratemporal epilepsy undergo invasive EEG recordings: There are no clear extratemporal epilepsy “syndromes,” there is no equivalent of hippocampal sclerosis on MRI, scalp EEG is often mislocalizing and even mislateralizing, and there are no standardized resection techniques.
Certain electrode types are more suited to some locations than others and are therefore indicated under different circumstances. Because depth electrodes penetrate the substance of the brain, they are indicated when EEG recording is needed from buried cortex (e.g., hippocampus, amygdala, or basal or interhemispheric cortex inaccessible to subdural electrodes). In practice, depth electrodes are often used to record from the mesial temporal lobes when mesial temporal lobe epilepsy is suspected. They are also useful for recording from other deep cortical structures that can be involved (insula, planum temporalis). They are also essential for targeting deep regions of suspected seizure onset, for example, one or several heterotopic regions of gray matter.2,76,105 Subdural electrodes overlie the surface of the brain and are most suited for bilateral neocortical EEG recording, often in combination with depth electrodes. Subdural grids are ideal for functional mapping of cortex, though regional preoperative localization is needed to select the (restricted) sites for placement. In practice, subdural strips are also often used additionally with grids for better sampling of other lobes ipsilaterally or contralaterally.
Depth Electrodes
Construction
Most commercially available depth electrodes are multicontact with platinum contacts. In the past, nichrome alloy and stainless steel were also used.87,91 These kinds of electrode contacts are all considered safe for postplacement MRI documentation of electrode contact positions with respect to anatomic landmarks,129 although high-resolution CT is often used for this purpose, with later MRI coregistration to improve localization in relation to anatomic definition. These multicontact electrodes carry 4 to 12 contacts along their length, usually spaced 5 to 10 mm apart at variable or constant intervals. Others have up to 18 contacts with 1.5 mm spacing. Exact locations of contacts along the shaft can be varied to start and terminate at predetermined locations. The electrodes are commercially available from several vendors. They are flexible and are inserted with a rigid introducer that lies alongside or within the electrode. Rigid electrodes are also used.
Insertion
Insertion of depth electrodes is now routinely done by standard stereotactic methodology utilizing a Todd Wells, BRW, or Lexell frame63,79; more recently, frameless stereotactic methodology has been used. Electrodes enter through burr holes with usual targets of medial temporal and medial frontal regions (Figs. 1 and 2); deep, extratemporal locations are also targeted. The total number of electrodes varies but rarely exceeds 15, the total depending on the hypotheses generated and the array of electrodes selected to adequately address those. Electrodes can be inserted parasagittally or orthogonally such that, for example, they traverse the occipital lobe and occipitotemporal junction or lateral temporal lobe en route to medial temporal regions. Because the electrodes sample multiple locations, additional areas of preferred sampling can also determine which approach is used; some centers use either parasagittal or orthogonal placements, depending on the individual situation, but most have a preference for one or the other approach based on prior experience. Some centers use a computer-driven robot for electrode implantation,15 particularly when nonorthogonal implantation is preferred either because of anatomic constraints or particular targets (insula, gyrus rectus). Neither approach is reported to be associated with a higher risk of complications. Lateral (orthogonal) placement is more common.
Recording
Commercial digital recording equipment allows referential recording and reformatting with desired electrode combinations, filter settings, and bipolar and referential display montages. This provides considerable flexibility. Most commercial equipment now allows sampling at 200 to 800 Hz; recent work in patterns of seizure onset suggests a potential benefit of using higher sampling rates. The multiple contacts, especially with combined procedures, usually require at least 64 channels of simultaneous EEG recording to display and interpret the information adequately, and often twice that number or more are optimal.
Electroencephalographic recording is done in parallel with continuous audiovisual correlation. The main goal is localization of the region of ictal onset recorded during spontaneous seizures. It is critical to scrutinize all electrode contacts carefully in relation to one another and in relation to the time of synchronized clinical seizure activity.
Seizures are precipitated by controlled withdrawal of antiepileptic medications during continuous recording. Current literature suggests that carbamazepine, phenytoin, valproate, or lamotrigine withdrawal does not produce false localization or atypical seizures59,90,94,111; the other, newer antiepileptic drugs have not been assessed in this regard. Barbiturate and benzodiazepine withdrawal should be avoided
because they can produce unreliable and/or false locali-zation.116 Usually, spontaneous seizures are recorded within 7 to 10 days despite the patient’s inactivity; seizures that occur earlier or later during the session do not have different profiles or accuracy for confident localization of the epileptogenic zone. Most centers prefer at least three seizures for analysis, and some require up to ten for the study to be considered complete. No rules can be developed, however, because seizures vary in their frequency, intensity, similarity to the usual clinical events, and similarity to one another. To this is added a variable number of subclinical seizures, recording mishaps, immediate postictal seizure onsets, immediate postoperative seizure onsets, insufficient clinical examinations, and other confounding variables that make the protocol and the interpretation as much an art as it is a science.
because they can produce unreliable and/or false locali-zation.116 Usually, spontaneous seizures are recorded within 7 to 10 days despite the patient’s inactivity; seizures that occur earlier or later during the session do not have different profiles or accuracy for confident localization of the epileptogenic zone. Most centers prefer at least three seizures for analysis, and some require up to ten for the study to be considered complete. No rules can be developed, however, because seizures vary in their frequency, intensity, similarity to the usual clinical events, and similarity to one another. To this is added a variable number of subclinical seizures, recording mishaps, immediate postictal seizure onsets, immediate postoperative seizure onsets, insufficient clinical examinations, and other confounding variables that make the protocol and the interpretation as much an art as it is a science.
Although the recording of spontaneous seizures remains the best way to assess the extent of the epileptogenic zone, some centers, mostly in Europe, use electrical stimulation, particularly when ictal discharges are poorly defined, multifocal, or widely extended from the onset of the seizure. Observation of a complete and habitual seizure sequence after such stimulation is the goal, to provide insight into the spatial organization of the epileptogenic zone and other structures. The activation of regions removed from the specific site of electrical stimulation, however, that are unsampled by the electrodes represents a difficult, potentially confounding aspect of interpretation. Chemical stimulation is generally avoided because of its potential to precipitate nonhabitual seizures.12
Risks
The risk of intracerebral hemorrhage after depth electrode study ranges from 1% to 4%.110 The use of intraoperative angiography, visualization of the cortical surface, and MR guidance might have reduced the hemorrhage rate, but insufficient information is available to conclude this definitively. Intracerebral hemorrhage is the most serious complication of intracranial electrode study and is reported only after use of depth electrodes, with several deaths as a result. Infection also occurs, but fatalities and long-term morbidity are rare.
Whether rigid electrodes have more potential for damage to normal cortical tissue than flexible ones is not established. One study reviewed the MRI appearance of 210 stainless steel depth electrode tracks 1 to 64 months after electrode removal.63 Of 57 patients studied, 33 had rigid electrodes and 24 had flexible ones, all inserted in paramedial locations from occipital or frontal entry points targeted to medial temporal and frontal locations. Punctate 1- to 2-mm hyperintensities along the electrode track were the most common abnormality, found in 41% of cases. Four tracks (2%) showed punctate signal voids consistent with microhemorrhage, and one patient had a macroscopic occipital lobe hemorrhage. The authors did not note any difference between rigid and flexible electrodes with respect to these findings. Another comparison study of complications from depth and subdural electrodes found consistent moderate to severe multifocal inflammatory reactions to subdural electrodes and only mild to moderate reaction to depth electrodes. Although depth electrodes were on occasion associated with clinically unsuspected hematoma formation, microhemorrhage was much more common under subdural electrodes. Smooth, blunt construction with adequate insulation between contacts is essential.
There seems to be no substantive long-term risk of depth electrodes to otherwise normal cortical tissue.3,102 They do not appear to cause epilepsy. Centers routinely employing a bilateral depth electrode study before surgery for epilepsy have similar short- and long-term success rates for seizure control to those that do not. Long-term relapse after apparent seizure cure occurs occasionally whether or not depth electrodes were part of the preoperative evaluation. Occasionally, the depth electrodes themselves (or the procedure under general anesthesia) seem to “cure” the epilepsy, an observation made on <0.5% of patients at several centers.91 These considerations suggest that depth electrodes are indeed not epileptogenic. With respect to clinically significant damage to the function of underlying tissue, in the absence of other complications or subsequent surgery, the literature does not document any negative functional effects of depth electrode investigation.87,91
Interpretation
Background
Background activity in depth electrodes conforms to expected rhythms on scalp EEG. Interpreters must remember, however, that because of the loss of frequency filtering from scalp and skull, and because recording is from a smaller volume of tissue, the waveforms are sharper and higher frequencies are noted, and some normal activity takes on an unusual appearance if not an unusual location. For example, high-amplitude potentials in motor cortex can mimic spikes. Occipital alpha activity, central mu rhythm, frontal beta predominance, lambda waves, and sleep spindles are seen as on surface EEG. Asymmetric activity, especially slowing, has the same significance as on scalp recording. Asymmetric or localized flattening of the recording as well as slowing can suggest progressive hemorrhage, fluid collection, or infection. However, a focal depression of activity might be seen with electrodes in white matter. In addition, some slow activity can occur in the first 48 hours after implantation and resolves spontaneously.
Interictal Activity
Interictal spikes are more widespread in intracranial EEG recording than in scalp EEG recording.79.91,114 Although interictal spiking rates often are higher in the area where seizures begin, for example, in medial temporal lobe epilepsy, this relationship does not always hold. Furthermore, multifocal extratemporal interictal spikes in both temporal and extratemporal epilepsy with a single ictal onset zone are common. Accordingly, most experts agree that interictal spikes recorded with depth electrodes (or any intracranial electrodes)
are less important for localization of the epileptogenic region than ictal onset.66 However, although only some of the interictal spikes recorded intracranially are associated with the epileptogenic area, some interictal spikes are generated there, and the ability to reliably distinguish those, perhaps because they are associated with particular background changes or have unique morphology, could advance localization. Accordingly, interictal paroxysmal abnormalities are always scrutinized because they can provide additional confirmation of localization, and eventually may prove of even more important localizing value in certain settings. This seems to be true, for example, in focal cortical dysplasia of Taylor, in which the interictal spikes can be a good marker of the extent of dysplastic cortex that needs removal to abolish the seizures.22,106
are less important for localization of the epileptogenic region than ictal onset.66 However, although only some of the interictal spikes recorded intracranially are associated with the epileptogenic area, some interictal spikes are generated there, and the ability to reliably distinguish those, perhaps because they are associated with particular background changes or have unique morphology, could advance localization. Accordingly, interictal paroxysmal abnormalities are always scrutinized because they can provide additional confirmation of localization, and eventually may prove of even more important localizing value in certain settings. This seems to be true, for example, in focal cortical dysplasia of Taylor, in which the interictal spikes can be a good marker of the extent of dysplastic cortex that needs removal to abolish the seizures.22,106
Gotman and Koffler37 reported an increase in spikes postictally; other authors confirmed this observation.49 It can be well demonstrated with intracranial recording. Gotman and Marciani did not localize the postictal spikes to the region of seizure onset,38 but Katz and Spencer49 felt that certain populations of postictal spikes do emanate from the area of epileptogenesis. This issue requires resolution in larger numbers of temporal and extratemporal epilepsies recorded with surface and intracranial electrodes. Certainly, many interictal spikes recorded with depth electrodes (or any intracranial electrodes) cannot be seen with any noninvasive technique, and further work to investigate their significance is needed to assess what determines their expression on surface EEG as well.
Based on earlier work in epileptic rat hippocampus, recent investigators using microelectrodes examined higher sampling rates and recorded high-frequency oscillations termed “ripples” (96 ± 14 Hz) and “fast ripples” (250 to 500 Hz) in hippocampus and entorhinal cortex of patients with mesial temporal lobe epilepsy. Whereas ripples were considered physiologic oscillations, fast ripples were predominant in the areas of seizure onset, suggesting that they might reflect pathologic hypersynchrony in epileptogenic tissue.101 High-frequency oscillations were also described (60 to 100 Hz) in neocortical areas, and they sometimes appeared in anticipation of seizure onset.123 Investigation of this potentially important pattern is ongoing.
Ictal Activity
Onset.
Although the goal of intracranial recording is widely accepted to be localization of the ictal onset zone, the criteria for interpretation of ictal onset in intracranial EEG have been slow to evolve.50 A variety of EEG patterns and frequencies occur at the start of seizures, but certain patterns predominate in particular locations. Spencer and Spencer92 found that medial temporal lobe seizure onset, often termed hippocampal seizure onset, tends to vary in exact location and morphology, appearing in hippocampus or entorhinal cortex in different seizures, in patients with mesial temporal sclerosis. The medial temporal lobe (hippocampal/entorhinal) onset pattern is distinguished by a 10- to 16-Hz rhythm. Medial temporal lobe seizure onset can also present as a characteristic pattern of slow (1 to 2 Hz), periodic spike activity in the same location as the 10- to 16-Hz onset rhythm but before it begins (Fig. 7).107 This periodic pattern can last from 5 to >100 seconds. This periodic pattern is seen almost exclusively in the hippocampus and is inversely related to CA1 cell loss, one marker of mesial temporal sclerosis.86 Sperling also observed a peculiar high-amplitude triangular wave pattern at the onset of seizures arising in amygdala.98
Ictal onset patterns appear to be more reproducible and consistent in location from seizure to seizure in neocortical epilepsy.85 Neocortical seizure onset is characterized by a combination of often superimposed slower (4- to 10-Hz) and faster frequencies at seizure onset (Fig. 8).39,50,85 Although Spencer et al. reported that the fast frequencies were in the vicinity of 40 to 50 Hz, other studies suggest that the frequency of recorded neocortical seizure onset can approach 200 Hz.4,35,39,50,85,113 Isolated low- or high-frequency seizure onset recorded from neocortex or medial temporal structures, however, might represent a propagation pattern rather than actual ictal onset.
In any cerebral location, apparent rhythmic activity or frank ictal discharges differing from background can develop focally but not propagate or produce symptoms. This so-called subclinical seizure activity has been correlated with an excellent outcome after surgery,100 specifically surgery in the area of the subclinical seizure discharge.108 It may have a somewhat different morphology or location than the clinical ictal discharge or appear identical to the early parts of clinical seizures. The recording of such subclinical discharges does not substitute for the recording of the full clinically typical seizures on which localization is based for purposes of resection.10
Propagation Patterns.
Successive involvement of cerebral regions in the rhythmic patterns characteristic of seizure discharges has often been called propagation, although no physiologic mechanism is implied for the term used in this context. Propagation time from one hippocampus to the other has been studied by several authors, all of whom found that long (>20 sec) interhippocampal propagation time correlated with excellent outcome after medial temporal lobe resection, implying that it signified a true epileptogenic zone in the medial temporal region (Figs. 7 and 9).53,54,88 Spencer et al.88 found that interhippocampal propagation time correlated inversely and significantly with CA4 cell counts. Because CA4 is the origin of the main interhippocampal commissural connection, cell loss there might indeed prolong the process of seizure propagation. Fewer than 25% of medial temporal lobe onset seizures propagate first to the contralateral hippocampus; most initially propagate to ipsilateral temporal neocortex or frontal lobe, and both of these patterns are fairly common (Figs. 7 and 9).1,50,96,97,98,99 The spread pattern can vary depending on the exact region of medial temporal origin; Chabardes et al.21 found that temporal/polar seizures propagated to superior temporal gyrus/periopercular cortex, whereas other mesial temporal seizures propagated to basal and lateral temporal cortex. Parietal and occipital lobe seizures can show variable propagation by infra-Sylvian or supra-Sylvian routes, with subsequent temporal or frontal electroclinical evolution.119,122 Seizure propagation recorded with intracranial electrodes follows known pathways but can be modified by damage to these pathways or their origins. Thus, frontal lobe seizures often show very rapid propagation to the contralateral frontal lobe, often too rapid to allow determination by visual EEG inspection of which frontal lobe triggered the seizure.91,120,121 It is these situations that stand to benefit most from computerized EEG analysis.
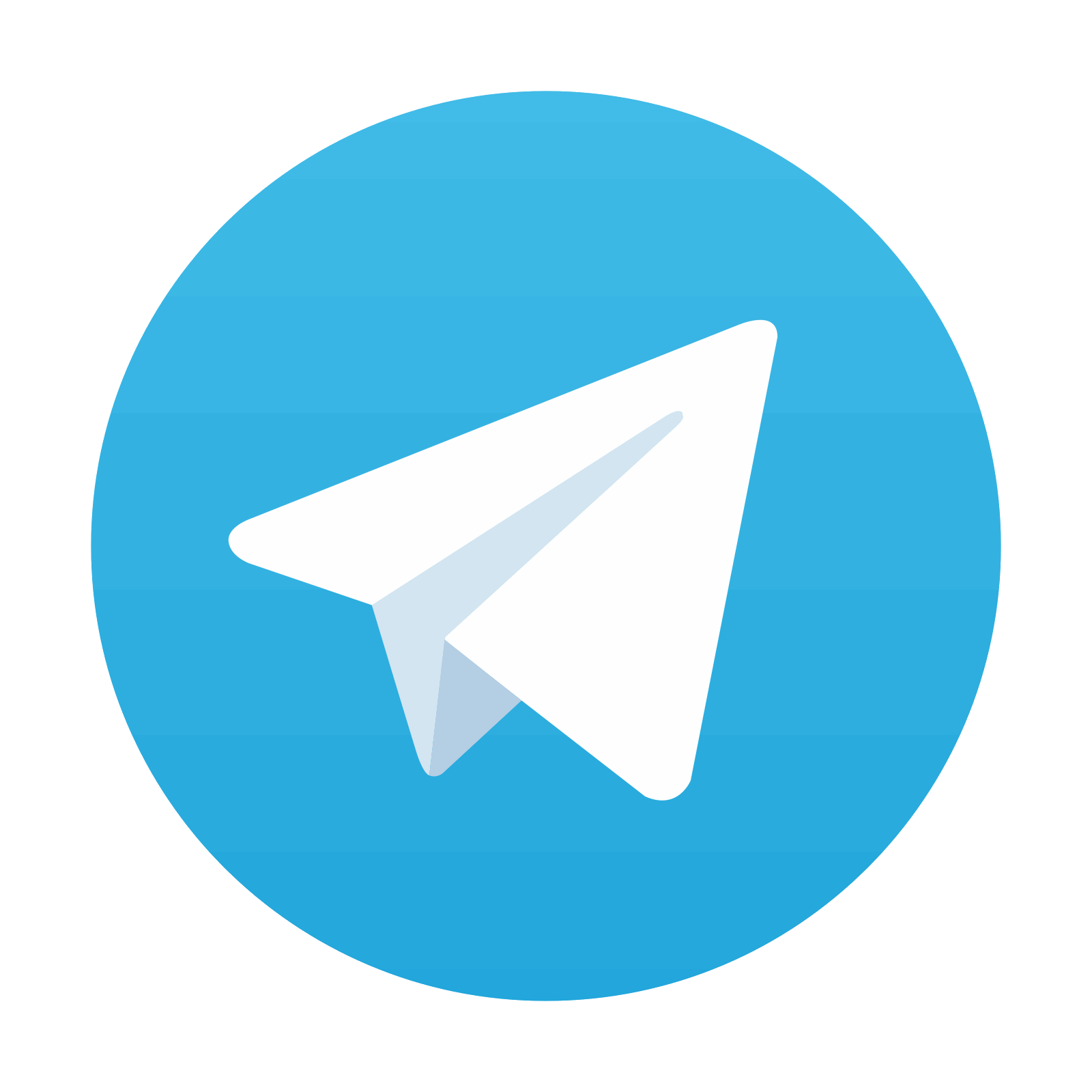
Stay updated, free articles. Join our Telegram channel

Full access? Get Clinical Tree
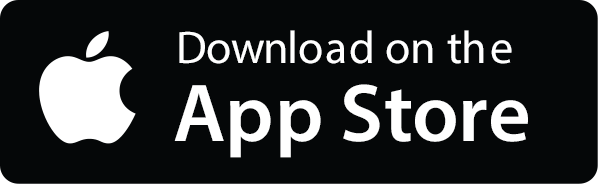
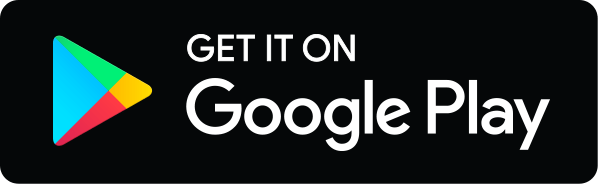
