Intracranial Electroencephalography and Localization Studies
Selim R. Benbadis
Elaine Wyllie
William E. Bingaman
In recent years, as new neuroimaging techniques have revealed hippocampal sclerosis, focal cortical dysplasia, and other subtle epileptogenic lesions, the use of chronically implanted invasive electrodes has decreased at many epilepsy surgery centers. The risks of invasive electrodes are not warranted when magnetic resonance imaging (MRI) and extracranial electroencephalography (EEG) disclose concordant evidence of an epileptogenic zone in a safely resectable area. Neuroimaging is now so advanced that the majority of patients who undergo resective epilepsy surgery do not require invasive EEG studies. In some cases, however, invasive techniques remain important because of discordant or poorly defined results from noninvasive testing or close proximity of the epileptogenic zone to eloquent cortex.
EXTRACRANIAL ELECTROENCEPHALOGRAPHY: THE STARTING POINT
The questions to be answered with intracranial electrodes are shaped by the results of the noninvasive evaluation, including extracranial EEG (1, 2, 3). Ideal for the initial identification of a suspected region of epileptogenesis, extracranial EEG provides a broad survey of EEG rhythms throughout both hemispheres and should be designed to yield the maximum localizing information about the epileptogenic zone (3). Sphenoidal electrodes (Fig. 77.1) may be helpful when mesial temporal lobe epilepsy is suspected. Generally, surface EEG-video monitoring is the initial test for any patient with frequent seizures despite medications, as 20% to 30% of adults will turn out to have psychogenic seizures (see Chapter 42).
The main limitation of extracranial EEG is decreased sensitivity to cortical generators (4,5). Intracranial electrodes overcome the sensitivity limitations of extracranial electrodes because they are closer to the cortical focus and free of the dampening effect of the skull and scalp. This increased sensitivity, however, is at the expense of more restricted sampling, or “vision,” and involves an enhanced risk of complications.
Intracranial EEG may fail to define further the epileptogenic zone if problem areas are insufficiently covered, but the use of large numbers of electrodes is limited by the proportional increase in the rate of complications. For this reason, intracranial electrodes should be used only after noninvasive testing (i.e., EEG, video semiology, and imaging) has “narrowed down” the epileptogenic zone to a limited brain region that can be covered safely and adequately by the chosen invasive technique.
The strength of the hypothesis based on the results of the noninvasive evaluation is a key to successful use of invasive techniques. The clearer the question formulated for testing, the greater the chance of success with the invasive evaluation.
This chapter provides an overview of the invasive techniques available for these difficult cases and reviews the major clinical situations and how they can be approached.
INTRACRANIAL ELECTRODES: AN OVERVIEW
Depth and subdural electrodes are the most commonly used intracranial electrodes. Because of limited sensitivity, foramen ovale and epidural peg electrodes are rarely or no longer placed.
DEPTH ELECTRODES
Surgical Aspects
Depth electrodes are multiple-contact “needles” of polyurethane or other material that typically are inserted into the brain by way of twist-drill skull holes under stereotactic guidance (6, 7, 8). Modern computer-assisted image-based stereotaxy has greatly improved the ease and precision of depth electrode placement. A target is chosen on the MRI scan, and entry point, trajectory, and depth are calculated by the computer to result in precise placement of the electrode tip within 1 mm of the target.
A common approach for patients with suspected bitemporal epilepsy uses three electrodes, each with eight contacts that are advanced transversely through punctures in the middle or inferior temporal gyri into the amygdala and anterior and posterior hippocampus on each side (Fig. 77.2). These allow the survey of electrical activity from the mesial structures, from infolded gray matter of basal temporal gyri, and from the lateral temporal lobe.
An alternative trajectory for the evaluation of mesial temporal epilepsy is the longitudinal placement of depth electrodes by way of occipital burr holes (8, 9, 10). With this approach, the electrode traverses the course of the hippocampus along its axis, sampling electrical activity throughout its length. Extratemporal foci are surveyed by carefully locating the electrodes according to structural lesions or particular gyri with suspected involvement in the epileptogenic zone (11).
Depth electrodes may be placed under local or general anesthesia, with the latter preferred for lengthy procedures involving multiple insertions, and can be removed under local anesthesia. Previous insertion of depth electrodes does not significantly limit further options of epilepsy surgery, including the subsequent use of other electrode types.
Advantages
The main advantage is direct access to deep structures for EEG recording very close to potential generators (12, 13, 14). Electrodes can be left in place for days to weeks with minimal risk of infection, permitting extensive ictal recording. Ictal EEG onset with depth electrodes often precedes onset with scalp and sphenoidal electrodes by 20 or 30 seconds, and in some cases, especially auras (“simple partial seizures”), the EEG seizure pattern may be seen only with depth recording. Depth electrodes may clearly locate seizure onset when extracranial localization is unclear. In addition to seizure onset, seizure termination may also have localizing and prognostic value, with unilateral termination
(as opposed to simultaneous bilateral, contralateral, or mixed termination) predicting better outcome after temporal lobectomy (15).
(as opposed to simultaneous bilateral, contralateral, or mixed termination) predicting better outcome after temporal lobectomy (15).
Disadvantages
Depth electrodes sample only a relatively small brain region, providing a very detailed but also very focused EEG sample. This focus may be inadequate when the issue is localization of seizure onset within a relatively large region such as the frontal lobe.
In addition, placement requires brain penetration. This raises theoretical concerns about damage to cortical areas outside the resection site and also makes depth electrodes inappropriate for the study of potential epileptogenic foci near vascular malformations. The examination of resected tissues has revealed gliosis, cystic degeneration, or microabscesses along the tracks of depth electrodes, but several studies (10) have failed to demonstrate any functional sequelae in the absence of clinically apparent bleeding or infection, and overall depth electrodes are safe (16).
The risk of bleeding or infection is only 0.5% to 5% (12,17). Routine imaging studies commonly reveal asymptomatic subdural collections of blood, but intraparenchymal hemorrhage is very rare [less than 1% in series (7,10) using modern stereotactic techniques]. The risk of significant hemorrhage is decreased by careful attention to electrode trajectories on preoperative planning studies so as to avoid major vascular structures.
SUBDURAL ELECTRODES (GRIDS AND STRIPS)
Surgical Aspects
Probably the most commonly used invasive electrodes, subdural electrodes are embedded in strips or sheets of polyurethane or other material and may be implanted subdurally over epileptogenic regions (Fig. 77.3) (18, 19, 20, 21, 22, 23, 24, 25). These discs of stainless steel or platinum alloy, approximately 2 to 4 mm in diameter, are embedded in polyurethane at fixed interelectrode distances, typically 10 mm, in various arrays. The strips and grids include one or more cables with bundled insulated wires connecting to the individual electrodes. Cables can be connected by means of various interface blocks to conventional EEG equipment for recording and stimulation. Other subdural grids have been designed with electrode contacts on both sides of the polyurethane sheet for recording from both surfaces, as in interhemispheric locations.
Strips can be inserted under fluoroscopic guidance through individual burr holes or trephines for bilateral placement when the side of seizure onset must be determined. The cables exit through a stab wound separate from the main incision to assist with anchoring of the strip and to decrease cerebrospinal fluid leakage and infection. Subdural strips may be placed under local or general anesthesia, although general anesthesia is preferred for multiple burr holes and multiple strip insertions. The risk of infection and hemorrhage with insertion of subdural strips has been reported to be less than 1% (9,21). Because mobility of implanted subdural strips may change the position of electrodes in relation to the intended recording target, serial skull roentgenograms should be performed to verify stability of position.
Grids are inserted by way of open craniotomy (Fig. 77.4). Flap design allows coverage of all regions of suspected epileptogenicity and subsequent access to any possible resection to the region of interest. Subdural plates may be “slid” beyond the edges of the craniotomy to cover adjacent areas, including basal temporal, basal frontal, and interhemispheric regions. Subdural grids are sutured to the overlying dura mater to prevent movement. A watertight dural closure around the electrode cables lessens the possibility of cerebrospinal fluid leakage. Whenever feasible, the overlying bone flap should be osteoplastic (attached to a vascularized muscle and periosteal pedicle) to prevent flap osteomyelitis. The electrode cable exits through a stab wound separate from the main incision, and watertight sutures are used at the exit site to reduce cerebrospinal fluid leakage. Despite these precautions, minor leakage frequently occurs without serious complications.
After completion of the evaluation with subdural electrodes, the patient is returned to the operating room for reopening of the craniotomy, removal of the subdural electrodes, and final resection of the mapped epileptogenic zone. This second operation typically is performed using general anesthesia, although local anesthesia is an option when further brain mapping is necessary. At reoperation, cultures are obtained from all layers of the wound, all electrode hardware, and the bone flap. If bacterial colonization of one or more wound layers is observed, the patient receives vigorous intravenous antibiotic therapy directed against the cultured organism(s) for two weeks following removal of the electrodes to reduce the risk of flap osteomyelitis.
With an overall rate of 26%, subdural grids present the greatest potential for complications (26). These include infection (12%), transient neurologic deficit (11%), epidural hematoma (2.5%), increased intracranial pressure (2.5%), infarction (1.5%), and death (0.5%). The occurrence of complications is associated with large numbers of grids/electrodes (more than 60 electrodes), lengthy monitoring (more than 10 days), older age, left-sided grid insertion, and burr holes in addition to the craniotomy. Improvements in grid technology, surgical technique, and postoperative care may reduce the rate of complications (26, 27, 28).
Functional Localization Studies
Functional localization techniques with subdural electrodes include cortical stimulation and evoked potential studies. Cortical stimulation involves passage of a small electrical current through individual electrodes, with close observation for symptoms or interference with cortical function (20,29). An alternating current is applied for 5 to 10 seconds, with subsequent stepwise advancement from 1 mA to a maximum of 15 mA or until symptoms or afterdischarges on EEG develop. Symptoms during stimulation may include positive motor phenomena (tonic or clonic contraction of a muscle group), negative motor phenomena (inhibition of voluntary movements of the tongue, fingers, or toes), somatosensory phenomena (tingling, tightness, or numbness of a part of the body), or language impairment (speech hesitation or arrest, anomia, or receptive difficulties). To screen for negative motor or language impairment during stimulation, the patient may be challenged to read or perform rapid alternating movements of the fingers, toes, or tongue. Signs or symptoms during stimulation of an electrode are interpreted to mean that the underlying cortex has importance for the affected function.
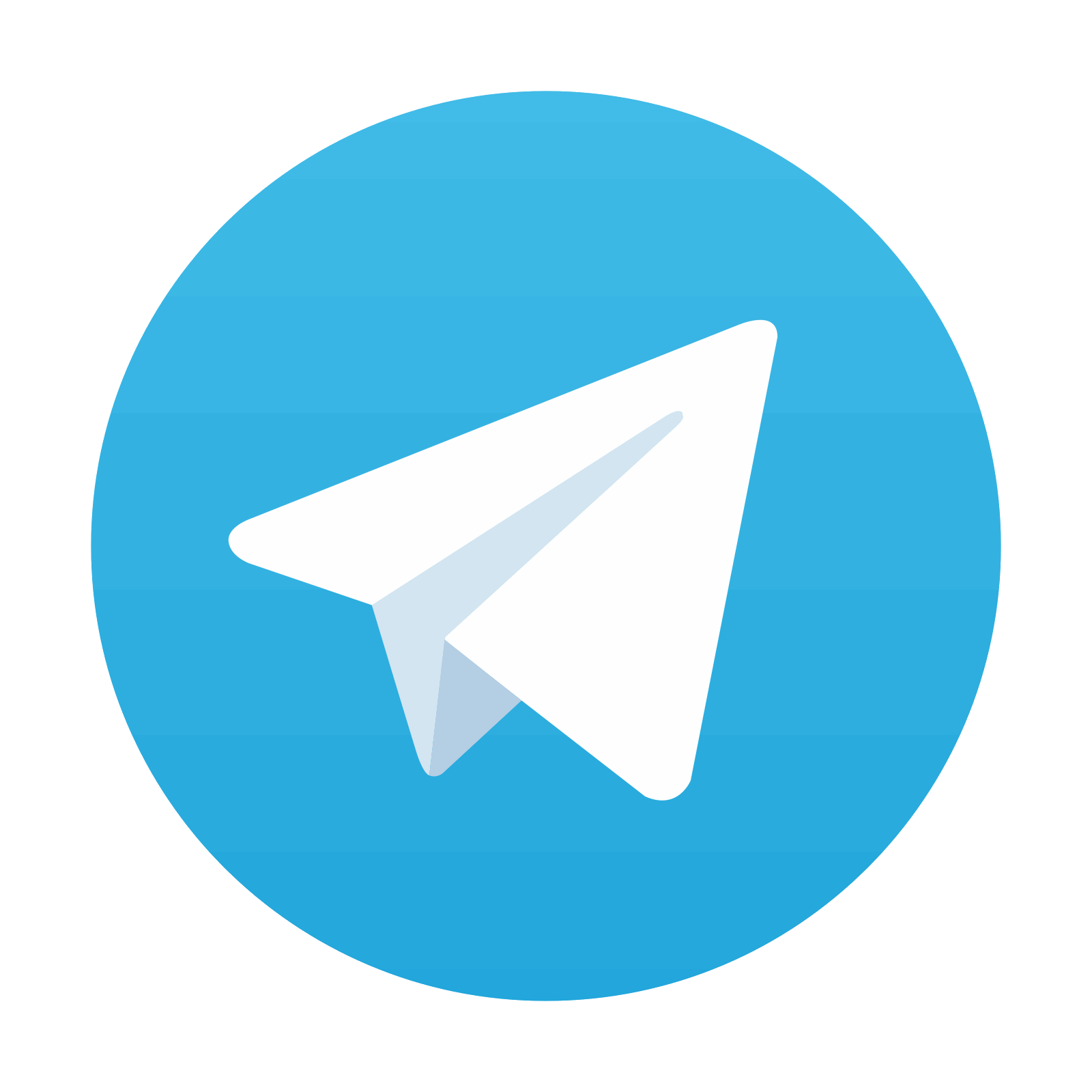
Stay updated, free articles. Join our Telegram channel

Full access? Get Clinical Tree
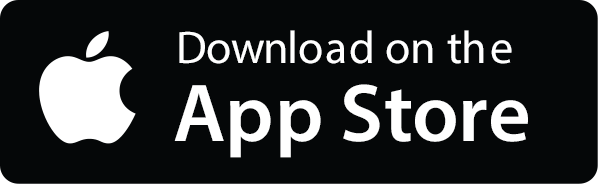
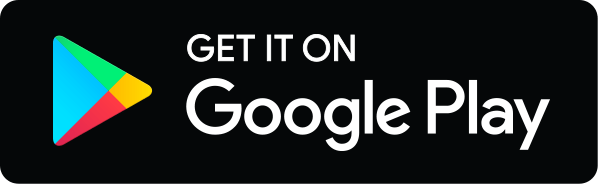