Local Motor Control
The final output from the central nervous system to skeletal muscle is from the motor neurons in the ventral horn of the spinal cord or in the brainstem. Since every action we carry out is the result of the activity of these cells, we need to look in some detail at what they do and the way in which they relate to each other at the spinal level. 

Motor neurons
Motor neurons are large, reflecting the length of their axons. Each controls a scattered group of individual muscle fibres called a motor unit: a unit may comprise as few as a dozen fibres in some of the muscles of the middle ear and in eye muscles, or as many as 1500 in large and crude muscles such as gluteus maximus.
In the cat’s leg, a single unit is capable of lifting some 10 g. Force is controlled partly by changes in the firing frequency of individual motor neurons, and partly by the process of recruitment, discussed in Chapter 2 (p. 38), in which more and more units are brought into play as the required muscle tension increases. Normally it is the smallest neurons, innervating the most ‘tonic’ and least fatigable muscle fibres, that are the first to be recruited.
Although individual motor neurons may sometimes fire at very low frequencies, this does not normally cause discrete twitching of the muscles because different units fire out of synchrony with one another. One of the functions of the Renshaw cells, mentioned in Chapter 3, is probably to provide a kind of lateral inhibition between motor neurons that discourages synchronization of this kind.


Motor neurons have an orderly and systematic arrangement within the cord, in clumps not quite well enough defined to be called nuclei, which reflects the topology of the muscles they serve. Medial neurons innervate the muscles of the trunk, the most distal parts of limbs are governed by the most lateral neurons, and flexors and extensors tend to be under the control of the more dorsal and ventral groups, respectively. Because these cells represent the ultimate funnel through which all nervous excitation must pass whenever a motor act is made, whatever its source, together they form what is sometimes called the final common path. On these cells terminate all the afferents from interneurons, and in some cases receptors, that serve the various spinal reflexes and responses, as well as some of the descending paths from higher levels. The quantity of information that thus converges on a single ventral horn cell is enormous, and is reflected in the huge size of its dendritic tree, which may often extend over a large part of the grey matter of the cord.
![]() |
![]() |
Sensory afferents entering the dorsal roots can give rise to spinal reflexes, by projecting – either directly or via interneurons – on to motor neurons (p. 55). It is never easy to define exactly what is meant by a reflex. Any response
that can be elicited from a spinal animal is certainly a spinal reflex.
But we have already seen that although a response may be essentially a spinal one, in the sense that its neural circuitry lies entirely within the spinal cord, yet one may not be able to elicit it in the spinal preparation because the usual facilitating, permissive, influences descending from the brain are absent. It is not quite enough to describe a reflex as an automatic, reproducible, response that is independent of the will, as one may readily influence one’s own spinal reflexes by willed inhibition or facilitation from above. For example, there is a mechanism in the cord called the withdrawal or flexion reflex that causes a rapid flexion when the skin is touched by a hot object or other noxious stimulus: yet we all know from personal experience that in cases of necessity – when carrying a plate that proves to be hotter than we thought when we picked it up – we can (up to a point) inhibit the reflex completely. We shall see later on that what at first sight appears the simplest and most automatic spinal reflex of all, the monosynaptic stretch reflex, is actually under almost total control from other, mainly descending, influences. In fact when we look at the mode of termination of the tracts descending from the brain, we find that the great majority of them end not on the motor neurons themselves, but rather on the interneurons that form part of these reflex arcs. Descending control is not so much of muscles as of actions, amounting to a selection from the cord’s repertoire: the brain plays on the spinal cord not as one plays a piano, but rather as one selects a disc from a juke box.
that can be elicited from a spinal animal is certainly a spinal reflex.

Clinical box 10.1 Motor neuron diseases
There exist a number of disease conditions involving the motor neurons, exhibiting a range of clinical and pathological patterns but all characterized by motor neuron degeneration and disabling motor dysfunction. The most common such condition is ‘amyotrophic lateral sclerosis’ (ALS) or Lou Gehrig’s disease, after the famous baseball player who succumbed to the disease. It is an incurable, relentlessly progressive and ultimately fatal degenerative condition. Clinically, the condition is suggested by progressive weakness and examination findings in keeping with both classical ‘upper’ and ‘lower’ motor neuron lesions, that is: increased tone and hyper-reflexia (UMN), alongside fasciculations and muscle wasting (LMN).
As well as the well-known motor manifestations, patients with ALS and its variants may exhibit the executive dysfunction typically seen with fronto-temporal dementia (poor judgement, personality change, obsessive behaviour), autonomic dysfunction and Parkinson-like features. Sensory symptoms, such as tingling, can occur but are usually mild and rarely manifest objective impairment of discrimination. Pathologically, as well as degeneration of pure ‘motor’ neurons and their glia, it is also recognized that cortical regions not traditionally considered motor also show pathological changes.
Weakness usually starts with the hands and lower legs, i.e. distal muscle weakness, difficulty with fine manipulation and tripping over as the foot dorsiflexors fail to lift the foot over obstructions in the sufferer’s path (foot drop). Weakness typically ascends throughout the limbs, culminating in near paralysis and inability to maintain an erect sitting posture. Swallowing and speaking become difficult as the bulbar musculature is affected. This is severely disabling as communication and even keeping food, drink and secretions out of the lungs becomes impossible. The respiratory muscles grow weak, the cough becomes inadequate to clear the chest, and most patients ultimately die of pneumonia and respiratory failure. Some patients choose to have a tracheostomy and percutaneous gastrostomy sited so they can be mechanically ventilated and fed directly into the stomach without the risk of choking.
In a small minority of cases, ALS is familial and a plethora of potential genetic culprits have been identified including superoxide dismutase (SOD). This enzyme catalyses the transformation of the superoxide to oxygen or peroxide. Superoxide is highly toxic, wantonly oxidizing and destroying sensitive and vital cellular structures. Indeed, superoxide is deliberately produced by the immune system to kill invading pathogens; SOD is the body’s defence against its own lethal weapon and when it is dysfunctional, it is easy to see how a neurodegenerative disease can ensue.
It goes without saying that ALS is a most cruel disease, provoking a range of reactions in sufferers and their families particularly regarding end-of-life decisions.
Descending pathways
Five important tracts descend from brain to spinal cord; four of these come from closely neighbouring parts of the brain, in the brainstem and medulla. These are the reticular formation, the vestibular nuclei, the red nucleus, and the tectum; the fifth source of descending fibres is the cerebral cortex. 

Reticular formation
If you take the brainstem, and remove all its sensory and motor nerve nuclei, all the fibre tracts that have to go through it, and all other well-demarcated structures, a considerable area of terra incognita is left unaccounted
for. This is the reticular formation (‘reticulum’ = net; RF), described by Brodal as formed of ‘diffuse aggregations of cells interspersed with fibres going in all directions’,
full of axons and dendrites weaving inextricably between one another, and with long branching axons going both upwards to the midbrain and forebrain, and also down to the spinal cord. However, one should not exaggerate its homogeneity and randomness. There are nuclei within it: although they can’t always be made out by classical light microscopy, they can be defined by their transmitters or their connections or functions, rather than being apparent using conventional histological techniques.
for. This is the reticular formation (‘reticulum’ = net; RF), described by Brodal as formed of ‘diffuse aggregations of cells interspersed with fibres going in all directions’,

![]() |
It is one of the most important and oldest structures in the brain, a direct descendant of the nerve nets controlling creatures like the sea anemone.
It stretches from the superior cervical spinal cord up to the intralaminar thalamic nuclei with which it merges at the top. One feature shared by cells in these primitive networks and in the reticular formation is the existence of long ascending and descending axons, as can be seen in the rat longitudinal section below. Another defining feature of the reticular formation is that dendritic fields tend to lie in slices perpendicular to the axis of the brainstem: hence the term isodendritic core. These dendrites receive profuse axon collaterals from sensory fibres ascending to higher levels, as well as from descending motor pathways (shown in red in this section of rat brainstem).

![]() |
Nevertheless, the reticular formation is not entirely shapeless and unstructured. Overall, it consists of essentially three longitudinal zones:
the raphe, very close to the midline (‘raphe’ = seam);
the medial zone, with cells that are particularly large because they are the origin of long ascending and particularly descending projections. Some of its subdivisions are shown below: the most important are the magnocellular (gigantocellular) nucleus with unusually large cell bodies, and the locus ceruleus;
the lateral zone, with smaller cells, and somewhat shorter axons; in particular, the parvocellular nucleus, which is more obvious at the bottom of the pons and top of the medulla, and has close association with cranial nerve nuclei: in a sense it is more sensory than motor, projecting predominantly upwards, partly via the mesencephalic reticular formation at the top.
![]() |
![]() |
So what does all this do? Despite being so diffuse and apparently homogeneous, its functions fall into two fairly clear-cut categories, both a kind of integration. In its upwards projections, it is concerned with the regulation of the level of activity of the brain, including such functions as
attention, sleep, arousal; these are dealt with in Chapter 14. Its downward-projecting systems are concerned with the generation of patterns of response, which can often be quite stereotyped. These in turn can be roughly divided into two kinds. Firstly, the reticular formation provides in a sense the executive brains behind much visceral and vegetative activity: the control of heart rate and the generation of the rhythms of breathing, for instance. Secondly, similar circuits seem to underlie other timing functions that are more obviously motor in the conventional sense. One of the best understood is the generation of saccadic eye movements, and it’s quite helpful to look at this in a little detail as an example of the rather sophisticated things the reticular formation is capable of.
attention, sleep, arousal; these are dealt with in Chapter 14. Its downward-projecting systems are concerned with the generation of patterns of response, which can often be quite stereotyped. These in turn can be roughly divided into two kinds. Firstly, the reticular formation provides in a sense the executive brains behind much visceral and vegetative activity: the control of heart rate and the generation of the rhythms of breathing, for instance. Secondly, similar circuits seem to underlie other timing functions that are more obviously motor in the conventional sense. One of the best understood is the generation of saccadic eye movements, and it’s quite helpful to look at this in a little detail as an example of the rather sophisticated things the reticular formation is capable of.
![]() |
Saccades were introduced in Chapter 9, and their visual control was discussed in Chapter 7. We saw that they are incredibly fast, as little as 20-30 ms in duration and with velocities around 800°/s. Yet the muscles are not intrinsically particularly fast, and – as can be seen above – in response to a step increase in motor neuron firing may take some half a second to settle down at a new length, which seems paradoxical. How can such sluggish muscles produce such extraordinarily fast responses? The answer comes from looking at the time course of the signal that is sent to them by their motor neurons to create a saccade. It consist of two components: a step of activity, designed to be just enough to hold the eye at its new position, and a very brief pulse of maximal firing, that kicks the eye as rapidly as possibly into place. It turns out that this very sophisticated pattern is generated by neural circuits in the prepontine and mesencephalic reticular formation, close to the motor neurons in nuclei III, IV and VI. Two kinds of neuron are found there, called tonic units and burst units, that provide the step and pulse components by summing together at the motor neuron. The burst neurons drive the tonic neurons through an indirect pathway, and descending from the superior colliculus and some other areas trigger whole thing off: a very neat piece of neural mechanism. It is easy to imagine similar special-purpose neural circuits for all kinds of movements, and we know that the machinery for much of the very basic but beautifully coordinated patterns of behaviour like walking are in the reticular formation, as well as some even more biologically basic behaviours such as chewing, grooming, suckling (lateral and pontine regions), coughing, swallowing, sneezing (ventrolateral) and facial expression.
![]() |
What are the routes by which all these movements are generated? In the case of eye movements, there are relatively direct projections on to the cranial nerve nuclei. But movements mediated by the spinal cord are brought about essentially by two large descending tracts that go down to the spinal cord, and terminate on both alpha and gamma motor neurons, in some cases causing excitation and sometimes inhibition: these are the two reticulospinal tracts:
![]() |
medial RST: ipsilateral from pons (nucleus pontis caudalis);
lateral RST: mostly ipsilateral from medulla (nucleus gigantocellularis).
Most of the fibres terminate diffusely on interneurons rather than on the motor neurons themselves, except for certain fibres of the lateral tract; they influence muscles of the trunk and proximal parts of limbs rather than the extremities. Other fibres in the lateral tract contain enkephalin and are likely to modulate the spinal transmission of afferent responses to noxious stimuli (p. 94).
In addition to acting more or less directly on the spinal cord, the reticular formation also has extraordinarily widespread indirect influence on the central motor system. It projects to the cerebellum, via the lateral and paramedian
nuclei, and to the basal ganglia via the mesencephalic reticular formation; and this is quite apart from the more diffuse ascending projections to basal ganglia and cortex that we’ll be looking at later. Conversely, nearly every level of the motor system sends projections down to the reticular formation: basal ganglia, cerebellum, superior colliculus, vestibular nucleus, substantia nigra, and much of the cortex, including motor and somatosensory areas. It is clear that the reticular formation provides a major alternative output mechanism for the higher control of movement, as well as doing things on its own initiative.
nuclei, and to the basal ganglia via the mesencephalic reticular formation; and this is quite apart from the more diffuse ascending projections to basal ganglia and cortex that we’ll be looking at later. Conversely, nearly every level of the motor system sends projections down to the reticular formation: basal ganglia, cerebellum, superior colliculus, vestibular nucleus, substantia nigra, and much of the cortex, including motor and somatosensory areas. It is clear that the reticular formation provides a major alternative output mechanism for the higher control of movement, as well as doing things on its own initiative.
Clinical box 10.2 Clinical aspects of the reticular formation
Because this area is anatomically and functionally diffuse, problems here rarely present as discrete, remediable lesions: any injury is likely to be accompanied by damage to structures with less subtle and more easily identified consequences, such as frank paralysis in the case of cord trauma affecting the corticospinal tract, or cranial nerve palsy if a nearby cranial nerve nucleus is affected by, for example, an inflammatory plaque of multiple sclerosis. Furthermore, injuries affecting the brainstem, as we shall see later, also tend to affect the conscious level, and identifying neurological deficits in comatose patients is very tricky. Because lesions to the reticular formation are difficult to identify, and even more importantly, there is little we can do about them, the reticular formation receives little formal attention from clinicians. However, injury to the brainstem can lead to several manifestations likely to be attributable to a damaged reticular formation.
Reduced conscious state
The reticular formation seems to be essential to maintain arousal and ‘consciousness’. With all higher centres intact, humans remain comatose if the reticular formation is injured. On the other hand, quite profound higher neurological injury is compatible with a degree of alertness if the reticular formation is intact, a sometimes ethically and philosophically challenging scenario.
The Cushing reflex
Raised intracranial pressure from a bleed, oedema or a tumour is associated with impaired blood flow into the cranium (which is, essentially, a non-expandable box). The resulting paucity of blood flow (ischaemia) stimulates the regions of the reticular formation responsible for augmenting blood pressure in a last ditch attempt to raise the blood pressure enough to force some blood into the cranium and keep the neurons alive. This premonitory clinical picture mandates emergency decompression, usually involving surgical removal of part of the skull.
Abnormal respiratory patterns
The central genesis of normal respiratory pattern is a complex and poorly understood area, but seems to reside largely in the reticular formation. Lesions of the reticular formation can yield a range of different breathing patterns depending on which precise region is injured – too fast, too slow, too shallow, too deep, or irregular and jerky. ‘Ondine’s curse’ is a rare condition which can be congenital or associated with brainstem injury, whereby the generation of breaths is no longer automatic. Patients suffer prolonged, potentially fatal, periods of apnoea when they fall asleep and in some cases the only remedy is mechanical ventilation throughout the night. (Ondine was a jealous nymph whose rather foolish lover swore that his every waking breath would be a testimony to his love for her. He was then caught out in his infidelity and cursed by Ondine’s king to forget to breathe should he fall asleep, which, the tale pre-dating Red Bull, he eventually did.)
The vestibular nuclei
In the course of evolution, areas of the reticular formation concerned with particular sources of sensory stimulation have tended to condense together to form separate nuclei, and migrate towards their common source of excitation. The vestibular nuclei seem to have emerged in this way under the influence of afferent fibres from the vestibular apparatus, which as we saw in Chapter 5, are concerned with sensing movements of the head and the direction of gravity. At least four nuclei make up the vestibular complex, of which the lateral (Deiter’s) nucleus is the origin of an important descending motor tract, the lateral vestibulospinal tract. As might be expected from the reticular origin of the vestibular nuclei, the course of this tract is similar to those of the reticulospinal tracts: virtually all the fibres are uncrossed, most end on interneurons, but some excite motor neurons monosynaptically. A medial vestibulospinal tract also exists, projecting bilaterally from the medial vestibular nucleus mainly to cervical and upper thoracic regions; some of its fibres are inhibitory. The functions of these tracts are essentially to maintain posture and to support the body against the force of gravity; consequently they are mostly concerned with the control of extensors rather than flexors. The vestibular nuclei are also closely associated with the cerebellum, one of the largest and most important higher motor areas, and the descending tracts may provide one way in which the
cerebellum may control the spinal cord: it has no direct projections of its own.
cerebellum may control the spinal cord: it has no direct projections of its own.
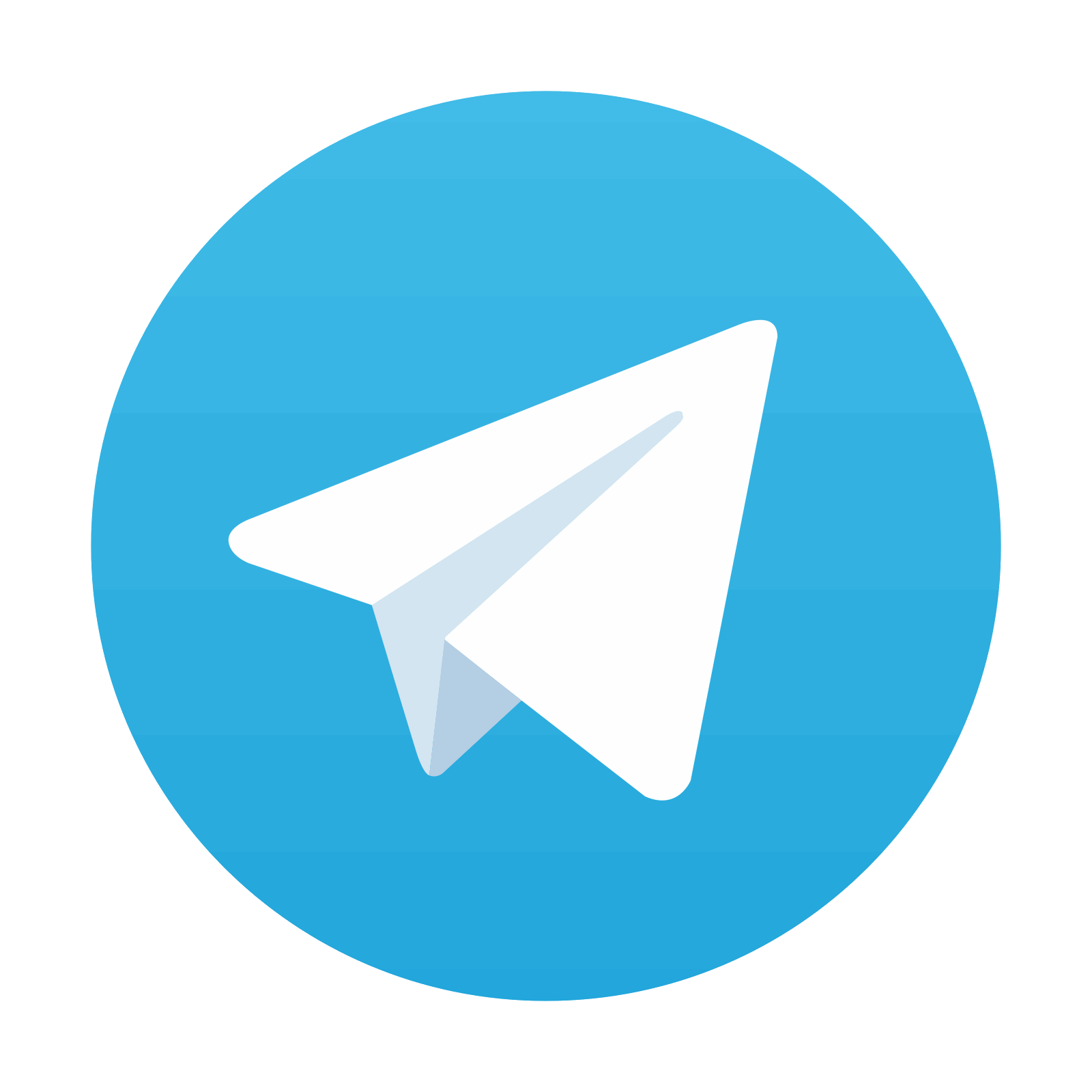
Stay updated, free articles. Join our Telegram channel

Full access? Get Clinical Tree
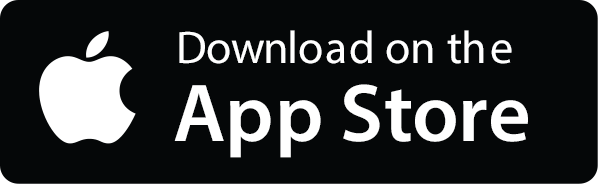
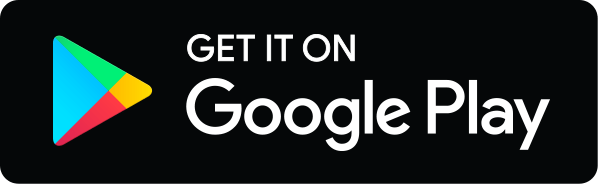