on a 1.5-T system, older MRI techniques may not include all of the modern sequences. Phased-array imaging at 1.5 T or 3 T, can increase lesion detection and should be considered for patients with focal epilepsies, particularly medically refractory seizures, in whom 1.5-T studies with a standard head coil yield normal or discordant results (15,16). The imaging sequences performed will depend on the duration and type of epilepsy as well as the age of the patient because the differential diagnosis differs in each of these categories (Table 74.1). The protocol for new-onset seizures in adults typically includes postcontrast studies; in children, whose seizures are less often secondary to neoplasms, a high-resolution protocol with contrast is used only on identification of a lesion. High-resolution T1-weighted and T2-weighted images through the hippocampi are recommended for mesial temporal lobe epilepsy. The same type of weighted images are appropriate for focal neocortical epilepsies but through the lobe of interest if no lesion is obvious and including in adults a gradient-echo T2 sequence. Therefore, when MRI studies are requested, new-onset seizures should be distinguished from epilepsy syndromes and the type of epilepsy should be described. Imaging protocols are discussed below.
TABLE 74.1 3T PROTOCOLa | ||||||||||||||||||||||||||||||||||||||||||||||||||||||||||||
---|---|---|---|---|---|---|---|---|---|---|---|---|---|---|---|---|---|---|---|---|---|---|---|---|---|---|---|---|---|---|---|---|---|---|---|---|---|---|---|---|---|---|---|---|---|---|---|---|---|---|---|---|---|---|---|---|---|---|---|---|
|
magnification. Increased T2 signal, which reportedly has a sensitivity of 93% and a specificity of 74% (28), can be detected in specific sectors, along with blurring of the internal architecture (Fig. 74.1). In more severe cases, decreased undulations in the hippocampal head can often be identified (29). T2-signal increases in the collateral white matter and blurring of the gray-white junction are also visible on this sequence. Table 74.2 summarizes the radiographic findings of MTS (5,9,29,30, 31, 32, 33, 34, 35, 36, 37, 38, 39, 40, 41, 42, 43, 44).
intensity on all sequences. Occurring in about 10% of the population, hippocampal sulcal remnants are 1- to 2-mm structures between the dentate gyrus and the cornu ammonis that represent a failure of normal embryogenic involution of the hippocampal sulcus. Choroidal fissure cysts, located just above the hippocampus, become more frequent with age (29,51).
TABLE 74.2 PRIMARY AND SECONDARY RADIOGRAPHIC FINDINGS OF MESIAL TEMPORAL SCLEROSIS | ||||||||||||||||||||||||||||
---|---|---|---|---|---|---|---|---|---|---|---|---|---|---|---|---|---|---|---|---|---|---|---|---|---|---|---|---|
|
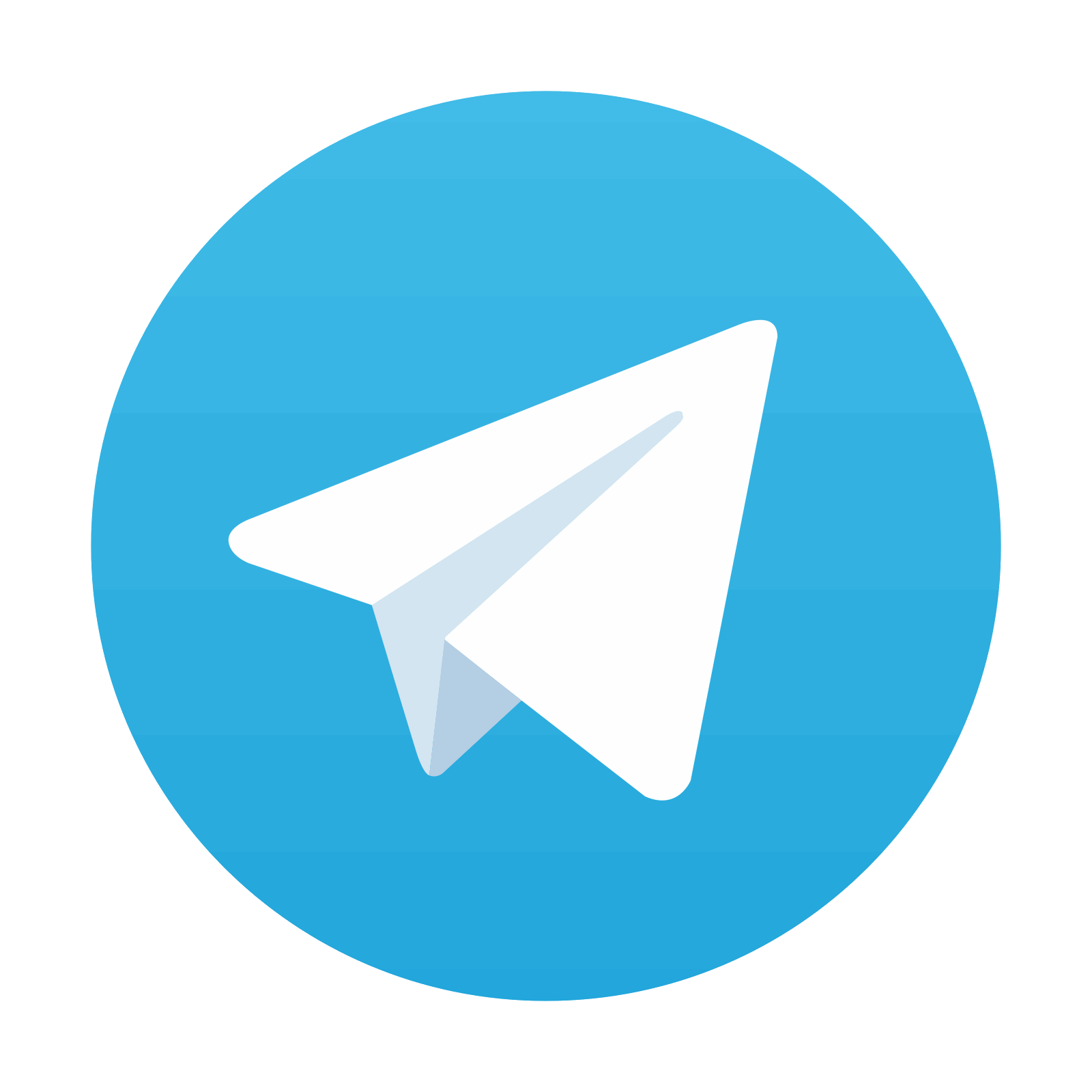
Stay updated, free articles. Join our Telegram channel

Full access? Get Clinical Tree
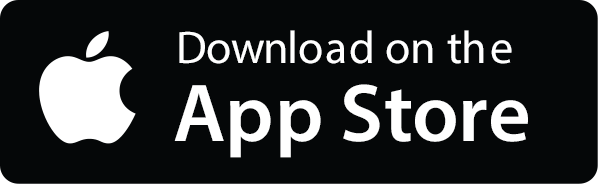
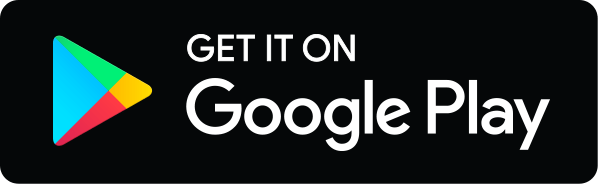