Abstract
Paroxysmal sympathetic hyperactivity (PSH) is a dysautonomic syndrome characterized by episodic increases in sympathetic and motor activity. PSH occurs after acquired brain injury of diverse etiology [most often traumatic brain injury (TBI), stroke, or anoxic brain injury], and core clinical features include tachycardia, hypertension, tachypnea, hyperthermia, diaphoresis, and increased motor activity. PSH occurs in 8–33% of the moderate-severe TBI population and is associated with negative neurologic, cardiopulmonary, and psychiatric outcomes. Two major theories exist to explain PSH: the disconnection theory and the excitatory:inhibitory ratio model. Evidence from case studies and cohort studies currently guides pharmacological management. Common classes of medications used to treat PSH include opiate agonists, GABA agonists, dopamine agonists, beta-blockers, and alpha-2 agonists. One ongoing trial, DASH After TBI, is investigating the impact of combined adrenergic blockade on autonomic parameters, as well as short- and long-term neurologic and functional outcomes.
Keywords
Adrenergic blockade, Autonomic dysfunction, Clonidine, Dysautonomia, Paroxysmal sympathetic hyperactivity (PSH), Propranolol, Sympathetic storm, Traumatic brain injury (TBI)
Introduction
Paroxysmal sympathetic hyperactivity (PSH) is a dysautonomic syndrome characterized by paroxysmal and episodic increases in sympathetic and motor activity ( ). PSH occurs after severe acquired brain injury of diverse etiology, and core clinical features of PSH include tachycardia, hypertension, tachypnea, hyperthermia, diaphoresis, and increased motor activity ( ). Additional clinical features may include agitation, mydriasis, decreased level of consciousness, horripilation, and flushing. Most reported cases of PSH follow traumatic brain injury (TBI), stroke, and anoxic brain injuries ( ); however, case reports of patients with PSH have also included etiologies such as hydrocephalus ( ) and encephalitis ( ). Although the clinical presentation is similar across etiologies ( ), we will focus on PSH after TBI.
Nomenclature
The syndrome that we refer to as PSH has been known by at least 31 other names in the published literature ( ); common terms include dysautonomia, sympathetic storm, diencephalic or autonomic seizures, autonomic dysfunction or storm, and paroxysmal autonomic instability with dystonia. The Consensus Working Group proposed that the term PSH accurately and specifically describes the syndrome without making an assumption about the underlying pathology driving the symptoms and thus should be the term used to unify literature on the syndrome ( ).
Incidence
In the first seven days post-TBI, autonomic arousal is very common; the incidence in an ICU population of individuals with severe TBI reached 92% ( ). However, not all of the individuals with autonomic arousal experience it to a pathological level. PSH incidence estimates range from 8% to 33% in the moderate-severe TBI population ( ), though the higher estimates are likely inflated due to inconsistencies in diagnostic criteria (eg, diagnosis at time of admission to hospital; ) and inclusion criteria (eg, febrile patients in the ICU; ). Incidence of PSH in patients with moderate-severe TBI who survive to rehabilitation is estimated to be 5.3% ( ). Reports of PSH in the pediatric literature are rarer, but estimates of incidence in children with acquired brain injury range from 9.7% ( ) to 14% ( ).
Diagnostic Criteria
A previous literature review yielded nine unique sets of diagnostic criteria for PSH ( ); the authors called for the establishment of standard diagnostic criteria by expert consensus. In 2014, the aforementioned Consensus Working Group identified 11 criteria for the diagnosis of PSH ( ). In this chapter we classify these 11 criteria by precipitating factor, episode onset, frequency/duration, clinical features, differentiation, and management ( Table 9.1 ). Likelihood of PSH diagnosis is based on the number of diagnostic criteria met, as well as the severity of the clinical features (eg, tachycardia or tachypnea); failure to meet all diagnostic criteria does not imply lack of PSH. PSH is a diagnosis of exclusion; alternative diagnoses to be ruled out include infection, seizures, edema, deep vein thrombosis, pulmonary emboli, hypoxia, thyroid storm, myocardial infarction, drug withdrawal, pain, anxiety, neuroleptic malignant syndrome, malignant hyperthermia, lethal catatonia, Cushing reflex, and spinal cord injury ( ).
Category | Diagnostic Criteria |
---|---|
Precipitating factor | Antecedent acquired brain injury |
Episode onset | Episodes are paroxysmal in nature |
Frequency/duration | Features persist ≥3 consecutive days |
Features persist ≥2 weeks postinjury | |
≥2 episodes daily | |
Clinical features | Clinical features occur simultaneously |
Sympathetic overreactivity to normally nonpainful stimuli | |
Absence of intraparoxysmal parasympathetic features during episodes | |
Differentiation | Features persist despite treatment of alternative differential diagnoses |
Lack of alternative explanations | |
Management | Medication administered to decrease sympathetic features |
Risk Factors
The majority of published literature on PSH consists of case reports, so understanding of risk factors for PSH is limited. However, published cohort studies suggest that younger age ( ), male sex ( ), and increased severity of injury (eg, presence of diffuse axonal injury, brainstem injuries, preadmission hypoxia; ) may be risk factors for PSH after TBI.
Onset
The first episode of PSH usually occurs within the first weeks after TBI. One cohort study reported a range of 1–22 days to first presentation ( ), while another cohort study reported onset at 4–14 days ( ). Greater variation in onset exists in case reports ( ). Onset of the paroxysmal episodes is often correlated with the cessation of sedatives or neuroblocking medications ( ). Before the onset of the symptoms, individuals with TBI who will go on to develop PSH have lower maximum respiratory rates and higher temperatures than individuals with TBI who do not develop PSH. However, maximum heart rate does not differ between the two groups ( ). The frequency of paroxysmal episodes varies, with some individuals presenting with one cycle per day (which is less than the frequency identified in the Consensus Working Group’s criteria), and others up to 16 cycles per day ( ). These episodes are often reactive, occurring after a stimulus such as nursing care, mobilization, or pain ( ) and may last minutes to hours ( ). In one retrospective study, episodes continued for an average of 74 days (15–204) post-TBI ( ); however, another study found episodes to last an average of 19 days (3–68; ) In a long-term follow-up study, 80% of individuals were not exhibiting symptoms of PSH at 1 year post-TBI ( ). However, sympathetic hyperactivity in response to nociceptive stimuli at 5 years postinjury has been observed more frequently in patients with a history of PSH after TBI than in patients without a history of PSH, suggesting long-term physiological changes ( ).
Neuroanatomical Basis
Attempts to localize the damage that leads to PSH has been inconclusive; differences in findings may be due in part to imaging methodologies used ( ). found a trend toward more focal, intraparenchymal lesions on computed tomography scans in individuals with PSH as compared to individuals without PSH, but other studies have found an increase in diffuse axonal injury and brainstem injuries in individuals with PSH ( ). A 2015 diffusion tensor imaging study found that fractional anisotropy (a measure of white matter integrity) was decreased in the splenium of the corpus callosum and in the right posterior limb of the internal capsule in patients with PSH after severe TBI ( ). A better understanding of lesion location may help identify the mechanism driving PSH.
Mechanism: Theories
case study of a 41-year-old female with a tumor near the third ventricle who presented with episodes of autonomic hyperactivity was commonly believed to be the first published case of PSH. Penfield termed the patient’s syndrome diencephalic autonomic epilepsy. However, the patient’s paroxysmal symptoms (including hypertension, tachycardia, hypothermia, and lacrimation) were characteristic of mixed (ie, both sympathetic and parasympathetic) autonomic hyperactivity ( ) and were likely due to intermittent obstructive hydrocephalus and increased intracranial pressure ( ). Thus, this case does not meet the current diagnostic criteria for PSH; instead, a case study presented by which meets the diagnostic criteria described earlier, likely represents the first published case of PSH ( ).
Although once thought to contribute, seizure activity has been ruled out as a cause of PSH, as electroencephalography has revealed no epileptiform activity during the sympathetic episodes. Further, antiepileptics are not successful in management of the syndrome ( ). Instead, there are now two predominant theories of the pathophysiology driving PSH: the disconnection theory and the excitatory:inhibitory model. The disconnection theory postulates that sympathetic hyperactivity is driven by a disconnection between the sympathoexcitatory centers in the hypothalamus or brainstem and higher control centers ( ). The excitatory:inhibitory ratio (EIR) model is an extension of the disconnection theory ( ). The EIR model is not specific to PSH; rather, it attempts to account for all of the acute autonomic overactivity syndromes (eg, autonomic dysreflexia, malignant hyperthermia). This model proposes that allodynia (the reinterpretation of a normal stimulus as nociceptive) occurs at the level of the spinal cord. Disconnection between the spinal cord and the tonic inhibitory system (located in the diencephalon) leads to an increased sympathetic response to the allodynia, which accounts for the observed symptoms in PSH. The model presumes that the damage is located below the diencephalon and that a triggering event is responsible for the onset of the sympathetic hyperactivity.
Neurological and Functional Outcomes
It is well established that patients who sustain trauma experience an increase in catecholamines postinjury ( ). Regardless of the mechanism behind the excessive sympathetic outflow in PSH, the observed catecholamine surge (a marker of sympathetic hyperactivity) can have deleterious effects. In patients with TBI, the level of catecholamines (especially norepinephrine) present in the first week postinjury is correlated with injury severity ( ) and predictive of short-term outcomes: higher levels of catecholamines are correlated with poorer neurological recovery ( ). However, not all studies support these findings ( ).
The occurrence of PSH is also associated with poorer outcomes. Some studies have found that PSH is related to longer stays in the ICU ( ), hospital ( ), and/or rehabilitation ( ). Additionally, findings suggest that individuals with PSH may require tracheostomy and mechanical ventilation at higher rates and for longer duration ( ), though data are inconsistent. Individuals with PSH remain in a coma longer ( ), have more systemic infections ( ), higher rates of spasticity ( ), and longer posttraumatic amnesia ( ). Findings related to global neurologic and functional outcomes are mixed; some studies have found greater disability outcomes among individuals with PSH ( ), while other studies have not ( ). As with estimates of incidence, it is difficult to synthesize the literature related to outcomes after PSH due to differences in diagnostic criteria and study methodologies. However, when differences are found between individuals with PSH and individuals without, those with PSH have poorer outcomes across studies, follow-up times, and domains.
Systemic Consequences
Up to 89% of patients with severe TBI experience nonneurologic organ dysfunction during their ICU stay, which is an independent predictor of in-hospital mortality and neurologic outcome ( ). Nonneurologic organ dysfunction accounts for 40% of in-hospital deaths after severe TBI ( ); the cardiovascular and respiratory systems are the most commonly affected ( ). Cardiovascular and/or respiratory dysfunction occurs most commonly between 2 and 4 days postinjury ( ), but may occur days to weeks later. The cardiopulmonary consequences of TBI are due in part to the excess of sympathetic outflow. For example, cardiac arrhythmias are often observed after TBI, though the majority resolve within 2 weeks of injury ( ). However, permanent changes on ECG, including QT interval prolongation and U waves, have been observed ( ); prolonged QT interval is a risk factor for fatal ventricular arrhythmias ( ). Additionally, myocardial injury and necrosis may occur, with left ventricular dysfunction as a common outcome ( ). A long-term decrease in heart rate variability is also associated with dysautonomia ( ). This “cardiac uncoupling” has been shown to be an independent predictor of mortality in a trauma ICU population ( ). PSH can also cause pulmonary hypertension and pulmonary edema ( ). Other systemic consequences of the sympathetic hyperactivity observed in PSH include immune suppression ( ) and hypermetabolism ( ).
Psychiatric Consequences
Episodic agitation, though not a core clinical feature in the Consensus Working Group’s definition, is often observed in individuals with PSH ( ). Agitation after TBI can be defined as a state of excess behavior that occurs after TBI while a patient is experiencing posttraumatic amnesia (or in some cases even after the resolution of posttraumatic amnesia; ) that can include aggression, akathisia, disinhibition, or emotional lability ( ). Estimates of agitation after brain injury range from 11% ( ) to 96% ( ). The commonly used Rancho Los Amigos Levels of Cognitive Function Scale includes agitation as one of the defining characteristics of emergence from coma after TBI ( ). Individuals who are agitated post-TBI have a longer duration of posttraumatic amnesia, longer length of stay in rehabilitation, and reduced cognitive function at discharge from rehabilitation. Differences in functional outcome between TBI patients with agitation and TBI patients without are not present by 6 months. Persistent agitation is correlated with persistent functional deficits ( ). Depression and younger age at time of injury are predictive of long-term aggression ( ). Simple agitation associated with emergence from coma can be differentiated from agitation due to PSH by the lack of autonomic instability in the former ( ). The incidence of agitation associated specifically with PSH is unknown.
Management of PSH
Management of PSH consists of efforts to eliminate the cause of the sympathetic hyperactivity, to decrease the frequency of episodes, or to minimize the intensity of the episodes ( ). With the exception of a case series using hyperbaric oxygen therapy with success ( ), published attempts to manage PSH have been primarily pharmacological in nature. Three approaches to pharmacological management of PSH exist ( ). The first approach targets allodynia (the trigger for PSH as described in the EIR model). Baclofen (a GABA-b receptor agonist) and gabapentin (a structural analogue of GABA) can be used in this manner. The second approach is inhibition of the increased sympathetic outflow itself by using centrally acting medications. This approach is consistent with both mechanistic theories. Commonly used medications in this category of intervention include morphine (an opiate agonist) and bromocriptine (a dopamine agonist). The third approach, also consistent with both mechanistic theories, is to block the impact of the catecholamines at the end organ. Medications in this category will be discussed in the following. Strong evidence to support the use of a specific medication regimen within any of these approaches is lacking; the literature is limited primarily to evidence from case reports and cohort studies. Discrepancies in the efficacy of medications within case report and cohort findings are likely due to medication factors (eg, timing, dosage, medication interactions), personal factors (eg, age, comorbidities), and other unknown modifiers.
Adrenergic Blockade
Beta-blockers, or beta-adrenergic antagonists, have been used to block the effects of the catacholemine surge seen in individuals with PSH after TBI. Class III evidence suggests that prophylactic and postinjury beta blockade reduce the risk of in-hospital mortality in patients with TBI ( ); however, the mechanism by which beta-blockers decrease the risk of mortality in the TBI population remains unclear. describe three potential mechanisms by which mortality is reduced: (1) beta blockade causes decreases in heart rate, stroke volume, and blood pressure, thereby lessening the burden on the heart and ultimately reducing cardiac dysfunction; (2) beta blockade reduces cerebral metabolism; (3) beta blockade reduces catabolism associated with an increase in catecholamines. The benefits of beta-blocker therapy may be moderated by other factors; for example, found that beta-blockers only provided a survival advantage in individuals with TBI who had elevated serum troponin, a marker of myocardial injury. Caution should be used when treating sympathetic hyperactivity with beta-blockers after TBI, as hypotension that can occur as a consequence of beta blockade is a known independent predictor of poor outcomes after TBI ( ).
Propranolol, labetalol, and metoprolol have all been used to treat PSH. Propranolol, a nonselective beta-blocker, is the most commonly used beta-blocker. Propranolol crosses the blood–brain barrier, and administration of propranolol in animal models of TBI has demonstrated reduced edema, increased cerebral perfusion, decreased cerebral hypoxia, and improved short-term (1 h) neurologic outcomes ( ). Additionally, clinical studies have demonstrated that propranolol reduces the resting metabolic rate ( ), reduces cardiac necrosis ( ), and decreases arterial catecholamine levels in patients with TBI ( ). Propranolol also reduces the intensity (though not the frequency) of agitation post-TBI ( ) and frequency and intensity of assaultive behavior in a mixed-etiology population of individuals with acquired brain injury ( ). Finally, propranolol has been shown to impact memory, though this has not been demonstrated in the TBI population ( ). Although propranolol is the most commonly used beta-blocker in the treatment of PSH ( ), both metoprolol (a selective beta-1 blocker) and labetalol (a nonselective beta-blocker and selective alpha-1 blocker) have been used in management post-TBI, though outcomes have been inconsistent ( ).
Clonidine, an alpha-2 agonist, has also been used for the treatment of PSH ( ). In both animal and human models, clonidine has been shown to reduce catecholamines ( ). However, successful management of PSH with clonidine has been variable in case studies ( ). Additionally, clonidine may be used for lowering blood pressure ( ) and has an analgesic effect ( ). Evidence that clonidine may impact attention and memory in healthy and patient populations is mixed and has not been demonstrated in a TBI population ( ).
The use of combined adrenergic blockade is supported by evidence from two randomized controlled trials (RCTs) that have found a survival advantage (at 4 weeks and at 12 months) for patients who receive antihypertensive adrenergic blockade as part of guidelines to decrease intracranial pressure and improve perfusion after TBI ( ). These guidelines, known as “the modified Lund Concept,” call for the use of metoprolol (a selective beta-1 agonist) and clonidine (an alpha-2 agonist).
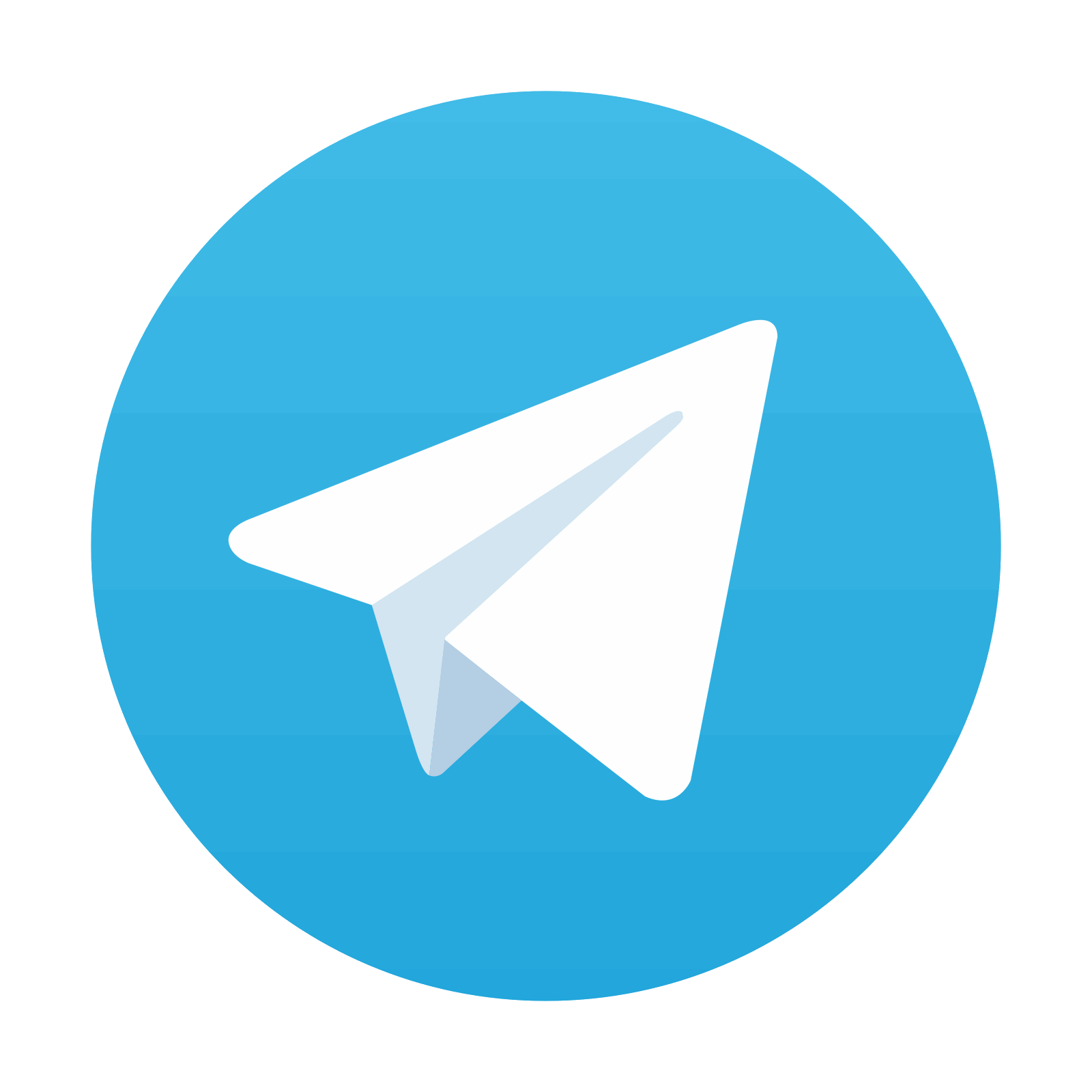
Stay updated, free articles. Join our Telegram channel

Full access? Get Clinical Tree
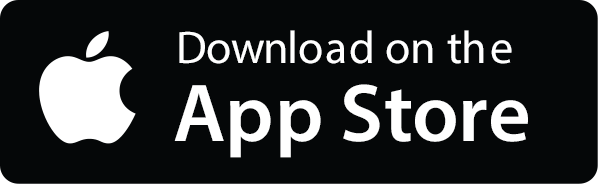
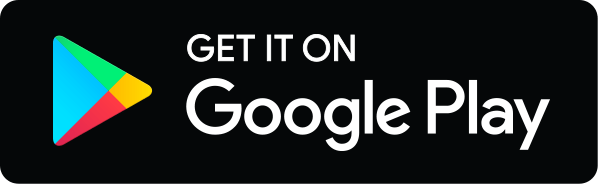