Chapter 36 Melatonin and the Regulation of Sleep and Circadian Rhythms
Abstract
All biological processes are organized according to time-dependent cycles, the most basic ones being the 24-hour cycle (the circadian rhythm)1,2 and seasonal cycles (the circannual rhythm).3 Such temporal regulation is universal in living species including humans, because for a billion years Earth’s rotation around its own axis, which determines the day–night alternation, has lasted about 24 hours.
Healthy subjects exhibit a circadian rhythm of secretion of many hormones, including melatonin, cortisol, and adrenocorticotropic hormone (ACTH), with additionally a circannual rhythm for testosterone in men and monthly cycles for reproductive hormones in women.4 There is also a circadian rhythm for body temperature, blood pressure, heart rate, and urinary excretion of electrolytes.5,6 As a result, there is harmonious synchronization in the functioning of cells, allowing the different physiologic functions to adapt to the needs of the organism with respect to the rest–activity cycle. Most documented rhythms are described for hypothalamic, hypophysial, gonadal, and adrenocortical functions. This is of interest because it is now well established that the hypothalamic pathways can integrate all the sensory information from the internal and external environment and fine-tune the integrated information with the intricate balance between the needs and demands of various physiologic systems, including brain function, the cardiovascular system, glucose and lipid metabolism, the gastrointestinal system, reproduction, and immunology.7
After a million years of evolution, the central clock located in the suprachiasmatic nucleus (SCN) has acquired autonomous functioning, with an endogenous period slightly longer than 24 hours,8 which persists even in the absence of temporal signals. However, in normal conditions, the circadian master clock is closely synchronized to the 24-hour environmental cycle, thanks to synchronizers, of which the external light–dark cycle is the dominant one.9 Light thus is the major zeitgeber for the SCN. Photic stimuli are transmitted to the SCN through the retinohypothalamic and the retinogeniculohypothalamic pathways.
The SCN circadian pacemaker function is regulated by the neurohormone melatonin, which is secreted in the pineal gland. Photic information is transmitted from the eye to the SCN, from the SCN to the paraventricular nucleus (PVN) of the hypothalamus, then to the pineal gland through a multisynaptic pathway. Melatonin is secreted at night and suppressed by light during the day. Thus melatonin secretion modulates the function of the circadian clock by signaling day–night information to the endogenous pacemaker. Because melatonin receptors are expressed in most organs, glands, and tissues, melatonin regulates the circadian pattern of secretion of hormones, including cortisol, the rest–activity cycle, and core body temperature (Fig. 36-1).10
Melatonin: Synthesis, Receptors, and Circadian Secretion Pattern
Biosynthesis
The neurohormone melatonin is secreted by the pineal gland. Melatonin derives from serotonin, which is first N-acetylated, then O-methylated to lead to N-acetyl-5-methoxytryptamine or melatonin, which is directly released into the general circulation.11 This synthesis occurs only during the night in all species, regardless of diurnal or nocturnal activity.12–15 Norepinephrine released during the night stimulates the beta-adrenergic receptors present on the pinealocytes and hence the synthesis of the enzyme N-acetyltransferase, which is synthesized and activated only during the night, and finally drives the rhythmicity of melatonin secretion (Fig. 36-2).16–18
Melatonin levels in the pineal gland are low during the day, begin to increase shortly after onset of darkness, reach peak levels at mid darkness, and then decrease late in the night to reach daytime levels shortly before light onset. Melatonin secretion is inhibited by light. This action is mediated via a photoreceptor system in melanopsin-containing, light-sensitive retinal ganglion cells.19
The activity of the pineal gland is very sensitive to the circadian and seasonal variations in length of the photoperiod, namely, the respective duration of light and dark exposure. In hibernating animals, melatonin regulates seasonality parameters. Exogenous administration of melatonin can also control the rest–activity rhythm entrainments through melatonin receptors located in the SCN (Fig. 36-3).
Melatonin Receptors
Three receptors have been cloned. The signaling pathways of melatonin receptors depend on cell type. MT1 and MT2 receptors are G protein–coupled receptors with seven transmembrane domains (Fig. 36-4). MT1 and MT2 receptors are coupled to cyclic adenosine monophosphate (cAMP) and calcium, whereas only MT2 receptors are coupled to cyclic guanosine monophosphate (cGMP) (Fig. 36-5).
In mammals, more than 100 structures containing melatonin receptors have been identified in the central nervous system, thus reflecting the important role played by melatonin at the cellular level on brain structures. MT1 and MT2 receptors are also present in peripheral tissues including the retina, adrenal glands, liver, arteries, heart, kidneys, gastrointestinal tract, macrophages, adipocytes, and blood platelets.20–22 The sensitivity of these receptors occurs at the dark-to-light transition and coincides with onset of melatonin secretion, thus explaining why exogenous melatonin is only active when administered at the proper time—transition from day to night, whatever the species (diurnal or nocturnal)—because melatonin-induced sensitization is present only during the night period.23,24 cAMP is a core component of the circadian pacemaker, suggesting that daily activation of cAMP signaling driven by transcriptional oscillators sustains the progression of transcriptional rhythm and could participate in synchronization.25
The third binding site of melatonin, MT3, has been characterized as a melatonin-sensitive form of the quinine oxidoreductase 2 (QR2, EC 1.6.99.2). MT3 (QR2) binding sites are widely distributed in mammals.26,27 The role of the MT3 binding site is not fully understood. In view of the relationship of QR2 with the redox status of cells, it might participate in the antioxidant activity of melatonin.28
Finally, melatonin has been shown to bind to a nuclear receptor identical to nuclear orphan receptors, called RZR/ROR.29,30
Melatonin Secretion
Melatonin secretion is characterized by a peak that occurs in the middle of the night, as shown by serial serum or saliva determinations over 24-hour periods. The presence of two peaks of secretion has also been reported at times31,32 and is related to possible dual oscillator control of melatonin synthesis.
In healthy persons the timing, amplitude, and even discrete profile of melatonin secretion are highly reproducible from day to day.33 No consistent gender differences have been found.
There are seasonal variations in human melatonin secretion, with an earlier phase in summer.34 Melatonin secretion level and duration increases in autumn and winter in northern latitudes in response to the shortening of the photoperiod.35 This allows the organism to adapt metabolic processes to the cold period by regulating thermogenesis36 and to adapt to hibernation with its prolonged sleep in hibernating animals.37 Melatonin secretion declines during development and with aging.38–40
Physiologic Role of Melatonin in the Rest–Activity Cycle: Consequences for Sleep
There is a close parallel between the circadian rhythms of rest–activity alternation and of melatonin secretion in normal diurnal animals and humans. Conversely, in nocturnal animals, onset of melatonin secretion coincides with locomotor activity. For this reason, a direct role of melatonin on sleep is not credible. There is no demonstrated causal relationship between onset and duration of melatonin secretion in normal conditions of sleep. In fact, melatonin does not affect the sleep pattern in rats with normal circadian rhythms. A possible sedative effect is excluded in rats in view of the lack of activity of melatonin in the spontaneous motor activity test, because melatonin does not decrease locomotor activity at doses up to 16 mg/kg (including those that re-entrain rest–activity cycles), and it does not induce behavioral changes at doses up to 32 mg/kg. These data are in agreement with other data obtained in mice in a free exploration test, where no sedative effect was observed with melatonin (up to 30 mg/kg). Furthermore, melatonin does not potentiate barbitural sleep.41
Normal human subjects living in usual conditions (active period in the day and rest in the night) have characteristic patterns of sleep-onset latency, sleep duration and efficiency (ratio of total sleep time to total sleep period), slow-wave sleep (SWS), and rapid eye movement (REM) sleep. Thus, objective measurements of sleep by means of polysomnography recording, together with subjective assessment of sleep quality, allow researchers to evaluate sleep–wake cycle disturbances in pathologic conditions by comparison with mean values recorded in healthy subjects.42
In addition, serial measurements of physiologic parameters (body temperature, heart rate, blood pressure) and circulating levels of hormones allow researchers to determine the normal circadian rhythm typically observed in healthy subjects, thus providing biomarkers of normal circadian rhythm, the ones most clearly dependent on circadian rhythm being body temperature and cortisol secretion. Core body temperature exhibits a clear circadian rhythm, with the highest value in the evening and the lowest in the middle of the night. Conversely, maximal secretion of cortisol takes place in the morning, with a progressive decline over the day to reach its lowest level in the evening, after falling asleep.10
Exogenous melatonin administration has a phase-shifting effect in the SCN’s neuronal activity, the chronobiotic effect. This effect varies according to the time melatonin is administered.43 In diurnal species, daily administration of exogenous melatonin in the evening, before endogenous melatonin secretion, advances the phase of the circadian rhythms, thus resulting in earlier falling asleep and waking.
Animal models of circadian rhythm disruption have been developed in order to reproduce the disorders observed in humans and analyze their mechanisms. In the murine models, various parameters have been assessed such as behavior, melatonin secretion, biomarkers, functionality of the hypothalamus, and clock genes. Concerning rest–activity disorders, several animal models have been set up, such as free-running conditions as a model of time-zone change syndrome, negative phase angle as a model of delayed sleep phase syndrome, and light-hour phase advance shift as a model of shift-work sleep disorders. In such conditions, wake–sleep cycles can be analyzed with computers using actimeters or transmitters recording locomotor activity and body temperature. In these different animal models, melatonin (0.1-3 mg/kg) was able to resynchronize disrupted rest–activity cycles.44,45 Similarly, administration of exogenous melatonin in healthy volunteers in the evening or at bedtime at small doses (0.3-10 mg) induces drowsiness and shorter sleep latency.33
Through its receptors in most organs and cells, melatonin modulates the circadian patterns of thyroid, growth, and hypophysogonadal hormones,46 as well as regulation of carbohydrate and lipid metabolism, vasoconstriction and relaxation of cerebral and coronary arteries, urine output, and immune and antioxidant responses and processes. Therefore, it is not surprising that melatonin secretion has been found to be altered in a number of pathologic conditions associated with the desynchronization of circadian rhythms.
Alterations in the usual rest–activity rhythm from exogenous, environmental causes (such as night work or long transmeridian flights) or endogenous misalignment of the circadian clock with the light–dark cycle result in sleep disorders, collectively defined as circadian rhythm sleep disorders (CRSDs).42,47–49 In all of these CRSDs, melatonin secretion is altered, as shown by determination of the dim-light melatonin onset.50
In view of the chronobiotic resynchronizing properties of melatonin, timed exogenous melatonin administration has been tried to improve sleep disorders associated with disturbed biological rhythms,47,51 and melatonin receptor agonists have been designed for this purpose.49
Exogenous melatonin administration has direct and circadian effects on sleep, without concomitant changes in sleep duration.52 Distal heat loss, via increased skin temperature, seems to be intimately coupled with sleep induction. Exogenous melatonin administration during the day, when melatonin is essentially absent, mimics the endogenous thermophysiologic processes occurring in the evening and induces sleepiness. Thus the sleep-facilitating effect of melatonin may be related to a hypothermic response mediated by peripheral vasodilation.53
The effects of administration of exogenous melatonin on sleep and endogenous melatonin secretion time54,55 were first studied in healthy volunteers and in patients with insomnia. Overall, administration of exogenous melatonin to healthy volunteers in the evening or at bedtime at small doses (0.3-10 mg) induces drowsiness and decreases sleep latency.56 In insomnia, no study showed a significant effect in nonelderly adults at doses of between 0.3 and 5 mg,57 but a single study demonstrated subjective improvement in sleep quality at a dose of 75 mg.58
Circadian Rhythm Sleep Disorders of Exogenous Causes Associated With Altered Melatonin Secretion Pattern
Shift Work and Shift Work Sleep Disorder
Shift working (or night work)—that is, working in the dark period normally devoted to sleep—is a major cause of desynchronization of biological rhythms and has multiple adverse clinical consequences including behavioral changes, sleep disorders, safety problems at work, altered hormonal and metabolic regulation, and susceptibility to hormone-dependant cancers.59–62
Impairment of Attention
Disturbances of attention have important implications in occupational medicine62,63 inasmuch as night work is becoming increasingly prevalent.64 Nowadays, nearly 20% of workers in industrialized countries are shift workers—that is, persons who work at night on regular or rotating schedules65—and 5% to 10% of them suffer from shift-work sleep disorder (SWSD),66 defined as excessive sleepiness during nocturnal working hours with insomnia in the daytime sleep period. Loss of alertness during working hours, mainly in the early morning, has important safety issues in drivers, especially in long-haul truck drivers,67 and in night nurses or physicians on duty in emergency departments, who are prone to professional errors.59,68 Tolerance to longer or irregular night work varies among individuals69–71 according to their spontaneous flexibility to changes in biological rhythms,62,63,72 which itself can depend on genetic determinants.73,74
Various combinations of timed bright light, scheduled dark, and melatonin have been proposed to reset circadian rhythms and improve sleep quality in night-shift workers,75–77 with encouraging results.50 However, exposing people to light therapy at night can have unwanted effects by chronically inhibiting melatonin secretion, and administration of exogenous melatonin to night or shift workers has not been of proven benefit in real-life conditions, most probably due to nonoptimal timing and duration of the treatment.
Increased Incidence of Hormone-Dependent Cancer
The Nurses’ Health Study (NHS), involving a cohort of 78,562 nurses, showed a significantly increased incidence of breast cancer among those who worked on a rotating night shift schedule for 30 years or more.78 An increased risk of breast cancer has also been observed in women exposed to light during the night, either at home79 or at work, including nurses, airline flight attendants, and radio operators.80–83 This increased incidence in breast cancer appears to be related to the altered circadian regulation of melatonin that results from exposure to light during night hours. Indeed, melatonin has immunomodulatory and antioncogenic properties84 and has been shown to suppress growth of murine mammary tumors.85 A lower urinary excretion of 6-sulfatoxymelatonin was found in nurses working the night shift compared with those working the day shift. The plasma level of melatonin is decreased in night workers, and nurses who exhibited the lowest levels of melatonin were especially at risk for developing breast cancer.86
Metabolic Dysregulation
Spiegel and colleagues87 evaluated the impact of sleep duration on the circadian profiles of plasma leptin, cortisol, thyroid-stimulating hormone (TSH), glucose, and insulin concentrations and on the sympathovagal balance. Sleep restriction (4 hours of sleep) resulted in a decreased nocturnal peak of leptin and TSH, concomitant with an elevated sympathovagal balance and an evening increase in cortisol level, together with higher plasma glucose levels during the first 90 minutes following breakfast despite a slight increase in plasma insulin, as compared with conditions of normal (8 hours) or extended (12 hours) sleep time. The same group reported that sleep restriction is associated with decreased leptin and increased ghrelin levels, concomitant with enhanced hunger and appetite.88
Thus, alterations in the neuroendocrine mechanisms involved in food regulation in sleep-deprived subjects concur to enhance appetite and favor the development of obesity, and epidemiologic studies have found an association between shift work and overweight.89–92 Moreover, sleep deprivation and shift work result in insulin resistance93–96 and thus favor the development of the metabolic syndrome and type 2 diabetes.97,98 Sleep restriction in young healthy subjects to 4 hours per night for 5 consecutive nights resulted in symptoms of early-stage diabetes.99 Experimental sleep restriction has been associated with an increase in risk factors for cardiovascular disease such as high levels of C-reactive protein.100 Interestingly, a genetic variant near MTNR1B has been associated with increased fasting plasma glucose levels and risk for type 2 diabetes, representing a possible link between circadian rhythm and glucose homeostasis.101
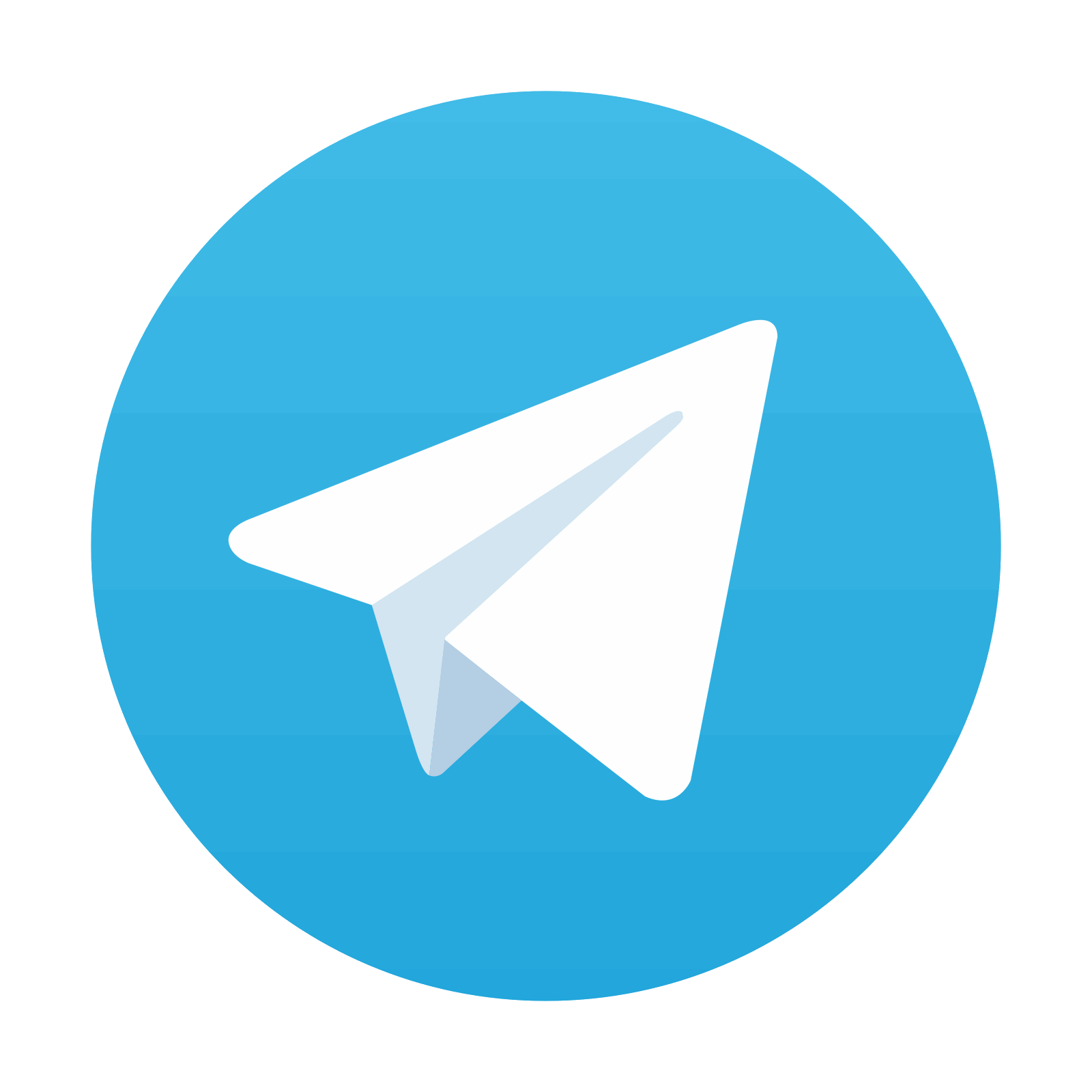
Stay updated, free articles. Join our Telegram channel

Full access? Get Clinical Tree
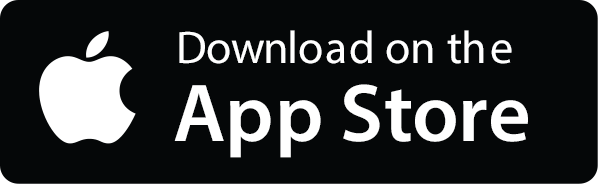
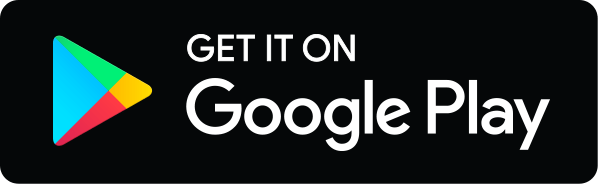