Chapter 4 Meningeal Coverings of the Brain and Spinal Cord
Living brain is on the soft and mushy side, despite the network of cytoskeletal proteins contained in neurons and glial cells. Without support of some kind, the central nervous system (CNS) would be unable to maintain its shape, particularly as we walk and run around and occasionally bump our heads. The brain and spinal cord are protected from outside forces by their encasement in the skull and vertebral column, respectively. In addition, the CNS is suspended within a series of three membranous coverings, the meninges (from the Greek word meninx, meaning “membrane”), that stabilize the shape and position of the CNS in two different ways during head and body movements. First, the brain is mechanically suspended within the meninges, which in turn are anchored to the skull, so that the brain is constrained to move with the head. Second, there is a layer of cerebrospinal fluid (CSF) within the meninges; the buoyant effect of this fluid environment greatly decreases the tendency of various forces (such as gravity) to distort the brain. Thus a brain weighing 1500 g in air effectively weighs less than 50 g in its normal CSF environment, where it is easily able to maintain its shape. In contrast, an isolated fresh brain, unsupported by its usual surroundings, becomes seriously distorted and may even tear under the influence of gravity (Fig. 4-1).
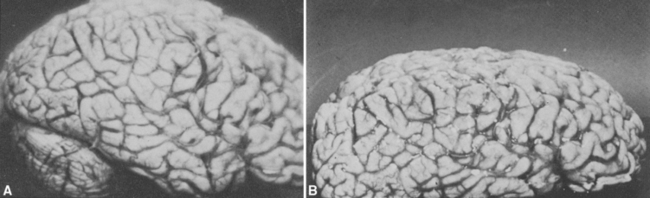
Figure 4-1 Effects of gravity and of partial flotation on the brain. A, An unfixed human brain in a vat of isotonic saline; normal shape is maintained. B, The same brain in air, obviously distorted by its own weight.
(From Oldendorf W: The quest for an image of brain, New York, 1980, Raven Press.)
There Are Three Meningeal Layers: The Dura Mater, Arachnoid, and Pia Mater
The three meninges, from the outermost layer inward, are the dura mater, the arachnoid mater, and the pia mater (Fig. 4-2). In common usage the mater is often dropped, and the three are referred to simply as the dura, arachnoid, and pia. The dura mater is by far the most substantial of the meninges, and for this reason it is also called the pachymeninx (from the Greek word pachy, meaning “thick,” as in thick-skinned pachyderms). The arachnoid and pia mater, in contrast, are thin and delicate. They are similar to and continuous with each other and so are sometimes referred to to-gether as the pia-arachnoid or the leptomeninges (from the Greek word lepto, meaning “thin” or “fine”). The dura mater is attached to the inner surface of the skull, and the arachnoid adheres to the inner surface of the dura mater. The pia mater is attached to the brain, following all its contours, and the space between the arachnoid and pia mater is filled with CSF.
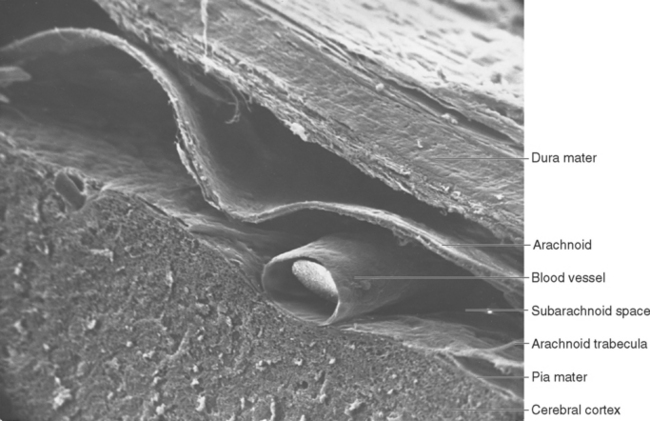
Figure 4-2 Scanning electron micrograph of the cranial meninges of a young dog. The apparent space between the dura mater and the arachnoid is an artifact of processing and would not normally be present.
(Courtesy Dr. Delmas J. Allen, Medical College of Ohio.)
The same three meningeal layers continue around the spinal cord but have a slightly different arrangement there, so spinal meninges are described separately toward the end of this chapter.
The Dura Mater Provides Mechanical Strength
The cranial dura is a thick, tough, collagenous membrane that adheres firmly to the inner surface of the skull (dura is the Latin word for “hard,” as in durable). It is often described as consisting of two layers: an outer layer that serves as the periosteum of the inner surface of the skull and an inner layer, the meningeal dura. Because these two layers are tightly fused, with no sharp histological boundary between them, the entire complex is ordinarily referred to as dura mater.
With few exceptions, no space exists on either side of the dura under normal circumstances because one side is attached to the skull and the other side adheres to the arachnoid.* However, two potential spaces, the epidural and subdural spaces, are associated with the dura (Table 4-1; see Fig. 4-14). Epidural (or extradural) space refers to the potential space between the cranium and the periosteal layer. Subdural space is commonly described as the potential space between dura and arachnoid and is sometimes said to contain a thin film of fluid. However, electron microscopic evidence indicates that the dura and arachnoid are normally attached to each other, and when they appear to separate, the splitting actually occurs within the innermost cellular layers of the dura. Parts of these potential spaces can become actual fluid-filled cavities in certain pathological conditions, most often as a result of hemorrhage (see Fig. 4-14).
Table 4-1 Spaces in the Cranial Meninges
Space | Location |
---|---|
Epidural | Potential space between dura and calvaria |
Subdural | Potential space in innermost dural layer, near dura-arachnoid interface |
Subarachnoid | Normally present, CSF-filled space; enlarged in cisterns |
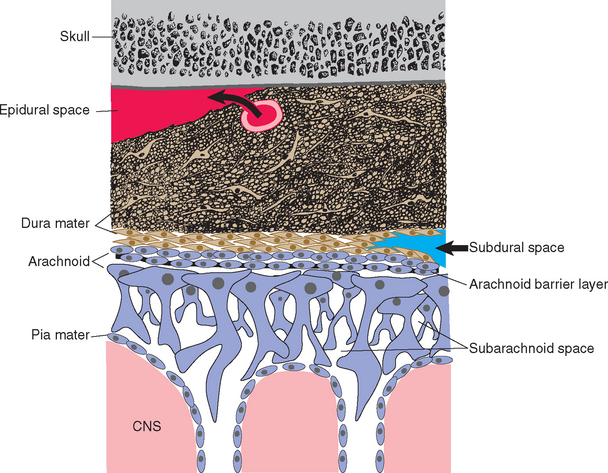
Figure 4-14 Actual spaces and potential spaces in and around the cranial meninges. Epidural space (not normally present) between the dura and skull may be opened up by blood from a ruptured meningeal artery or, less commonly, a torn dural venous sinus. Subdural space (not normally present), typically within the dura near the latter’s junction with the arachnoid, may be opened up by blood from a vein that tears as it crosses the arachnoid to enter a dural sinus. Dark bars joining superficial arachnoid cells represent the tight junctions that are the basis of the barrier properties of this portion of the arachnoid.
Dural Septa Partially Separate Different Intracranial Compartments
There are several places where the inner dural layer folds in on itself as a sheetlike protrusion into the cranial cavity, each called a dural reflection or dural septum. The principal dural reflections are the falx cerebri between the two cerebral hemispheres and the tentorium cerebelli between the cerebral hemispheres and the cerebellum (Fig. 4-3). The falx cerebelli is a small reflection that partially separates the two cerebellar hemispheres. The diaphragma sellae, another small reflection, covers the pituitary fossa, admitting the infundibulum through a small perforation.
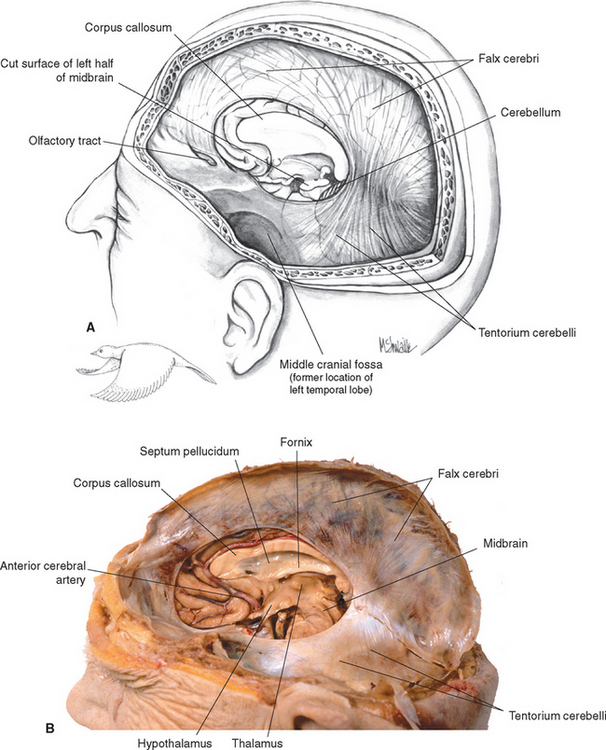
Figure 4-3 Shape and spatial relationships of the major dural reflections. A, The cerebellum and the right cerebral hemisphere are drawn in on the other side of the tentorium cerebelli and falx cerebri. The small bird in the lower left reminds certain individuals of the shape of the tentorium cerebelli. B, A prosection similar to the drawing in A, showing the midbrain passing through the tentorial notch.
(A, drawn from a dissection by Gary Jenison, University of Colorado Health Sciences Center. B, courtesy Dr. John W. Sundsten, University of Washington School of Medicine.)
The falx cerebri (from the Latin word falx, meaning “sickle”) is a long, arched, vertical dural sheet (Figs. 4-3 and 4-4A) that occupies the longitudinal fissure and separates the two cerebral hemispheres. Anteriorly it is attached to the crista galli of the ethmoid bone. The falx curves posteriorly and fuses with the middle of the tentorium cerebelli, ending posteriorly at the internal occipital protuberance. The inferior, free edge of the falx generally parallels the corpus callosum, but the falx is somewhat broader posteriorly than it is anteriorly, so the free edge comes closer to the splenium of the corpus callosum than to the genu. The anterior portion of the falx frequently contains a number of perforations.
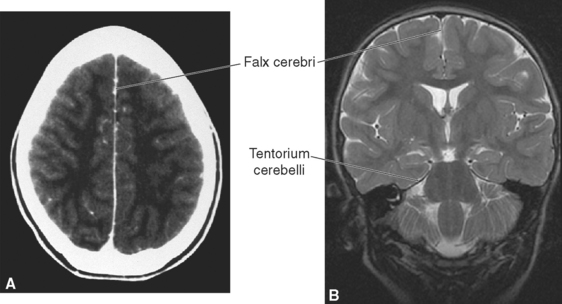
Figure 4-4 Major dural septa as seen in clinical images. A, Contrast-enhanced computed tomography scan (see Chapter 6) showing the falx cerebri between the two cerebral hemispheres. B, Coronal magnetic resonance image (see Chapter 5) showing the falx cerebri and tentorium cerebelli.
(Courtesy Drs. Raymond F. Carmody [A] and Joachim F. Seeger [B], University of Arizona College of Medicine.)
The tentorium cerebelli separates the superior surface of the cerebellum from the occipital and temporal lobes, defining supratentorial and infratentorial compartments. The supratentorial compartment contains the cerebrum, and the infratentorial compartment (or posterior fossa) contains the brainstem and cerebellum. Because the cleft between the cerebrum and cerebellum is not horizontal or flat, neither is the tentorium. Rather, it is roughly the shape of a bird with its wings extended in front of it; the bird’s body corresponds to the midline region where the falx joins the tentorium, and its wings correspond to the rest of the tentorium, which extends anteriorly (Fig. 4-3). Posteriorly the tentorium is attached mainly to the occipital bone. This line of attachment continues anteriorly and inferiorly along the petrous temporal bone. The free edge of the tentorium also curves anteriorly on each side, almost encircling the midbrain (Fig. 4-5). This space in the tentorium, through which the brainstem passes, is called the tentorial notch (or tentorial incisure). It is of great clinical significance, as discussed later in this chapter.
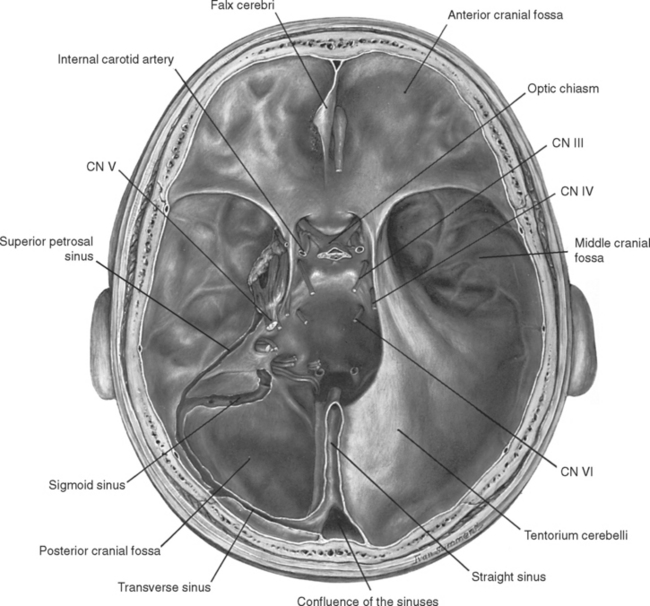
Figure 4-5 Dural lining of the base of the skull. The falx has been removed except for a small anterior portion. The left half of the tentorium has also been removed, exposing the posterior fossa (where the cerebellum was). CN, cranial nerve.
(From Mettler FA: Neuroanatomy, ed 2, St Louis, 1948, Mosby.)
The Dura Mater Contains Venous Sinuses That Drain the Brain
As noted previously, the two layers of the cranial dura are tightly fused, and there are no pathological conditions in which an intradural space (i.e., a space between the two layers) develops. However, at some edges of dural reflections (most often attached edges), the two layers are normally separated to form venous channels, called dural venous sinuses, into which the cerebral veins empty. These sinuses are roughly triangular in cross section and are lined with endothelium (Fig. 4-6). The locations of the major sinuses can be inferred by considering the lines of attachment of the falx and the tentorium. The superior sagittal sinus is found along the attached edge of the falx, the left and right transverse sinuses along the posterior line of attachment of the tentorium, and the straight sinus along the line of attachment of the falx and tentorium to each other (Figs. 4-5 and 4-7). All four of these sinuses meet in the confluence of the sinuses (also called the torcular, or torcular Herophili—“winepress of Herophilus”) near the internal occipital protuberance. Venous blood flows posteriorly in the superior sagittal and straight sinuses into the confluence, and from there through the transverse sinuses. Each transverse sinus continues, from the point where it leaves the tentorium, as the sigmoid sinus, which proceeds anteriorly and inferiorly through an S-shaped course and empties into the internal jugular vein (Figs. 4-5 and 4-7; see also Fig. 6-32).
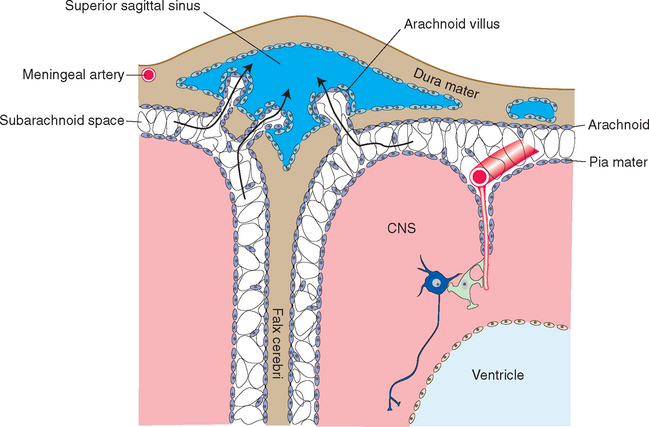
Figure 4-6 Section through the superior sagittal sinus showing the movement of CSF from the subarachnoid space, through the arachnoid villi, and into the sinus.
(Modified from Hamilton WJ: Textbook of human anatomy, ed 2, St Louis, 1976, Mosby.)
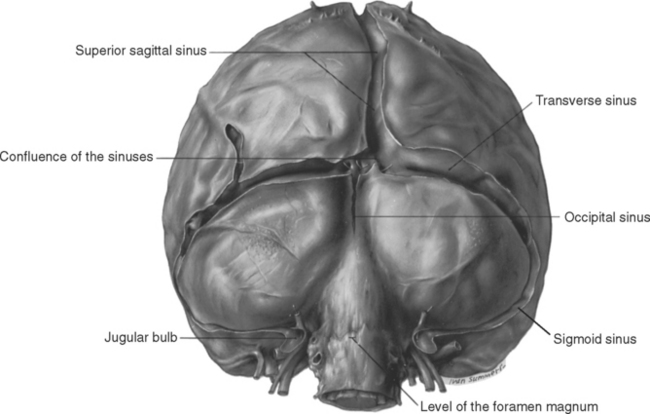
Figure 4-7 The brain, still encased in dura, viewed from behind. Note the asymmetry of the confluence of the sinuses. Note also the jagged line at the foramen magnum level, corresponding to the cut edge of the periosteal layer of the cranial dura. Below this line, a single-layered dural sheath continues around the spinal cord.
(From Mettler FA: Neuroanatomy, ed 2, St Louis, 1948, Mosby.)
The confluence of the sinuses is generally not a symmetrical structure. Usually most of the blood from the superior sagittal sinus flows into the right transverse sinus, whereas blood from the straight sinus flows into the left transverse sinus (Figs. 4-5 and 4-7; see also Fig. 6-31). Occasionally, the two transverse sinuses are not interconnected at all.
In addition to receiving cerebral veins, the major dural sinuses are connected with several smaller sinuses (see Fig. 6-31). The inferior sagittal sinus, in the free edge of the falx cerebri, empties into the straight sinus. The small occipital sinus, in the attached edge of the falx cerebelli, empties into the confluence of the sinuses (Fig. 4-7). The superior petrosal sinus, in the edge of the tentorium attached to the petrous temporal bone, carries blood from the cavernous sinus to the transverse sinus at the point where the latter leaves the tentorium to become the sigmoid sinus (Fig. 4-5). The inferior petrosal sinus follows a groove between the temporal and occipital bones, carrying blood from the cavernous sinus to the internal jugular vein.
The Dura Mater Has Its Own Blood Supply
The arterial supply of the dura comes from a collection of meningeal arteries. These are somewhat misnamed because they travel in the periosteal layer of the dura and function mainly in supplying the bones of the skull; however, many small arterial branches penetrate the dura itself. The largest of the meningeal arteries is the middle meningeal artery, a branch of the maxillary artery, which ramifies over most of the lateral surface of the cerebral dura. Anteriorly the dura is supplied by branches of the ophthalmic artery, and posteriorly it is supplied by branches of the occipital and vertebral arteries. Meningeal veins, also located in the periosteal layer, generally parallel the arteries.
The Dura Mater Is Pain Sensitive
Remarkably, the brain itself, as well as the arachnoid and pia mater, is not sensitive to pain (in the sense that physical stimulation of these structures is not painful). As a consequence, some neurosurgical procedures can be carried out without general anesthesia. The principal pain-sensitive intracranial structures are the dura mater and proximal portions of blood vessels at the base of the brain.
Most of the cranial dura, except for that of the posterior fossa, receives sensory innervation from the trigeminal nerve. Dural nerves follow the meningeal arteries and end near either the arteries or the dural sinuses. Except in the floor of the anterior cranial fossa, areas of dura between branches of meningeal arteries are innervated sparsely, if at all. Deformation of these endings is painful and is the cause of certain types of headache (Fig. 4-8). Interestingly, the way the pain is perceived depends on whether endings near meningeal arteries or endings near dural sinuses are stimulated. In the former case, the pain is fairly accurately localized to the area of stimulation. In the latter case, as in the case of stimulating the dura in the floor of the anterior cranial fossa, the pain is referred to portions of the peripheral distribution of the trigeminal nerve, such as the eye, temple, or forehead.
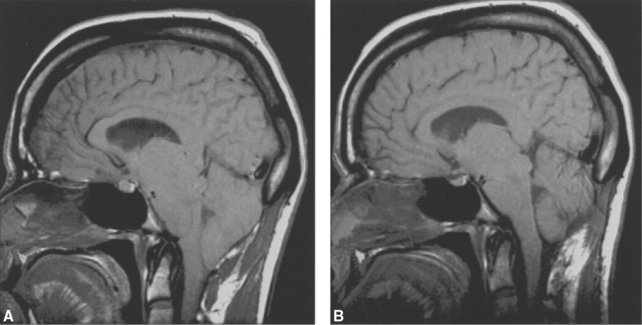
Figure 4-8 Headache caused by mechanical deformation of the meninges. This 40-year-old man had a several-year history of debilitating headaches that were relieved by lying down. He was found to have a meningeal diverticulum at the level of the second lumbar vertebra, through which CSF presumably drained. This interfered with the normal flotation effect of CSF, causing “sagging” of the brain (A) and consequent traction on the meninges. After ligation of the diverticulum, the brain assumed a nearly normal shape (B).
(From Schievink WI et al: J Neurosurg 84:598, 1996.)
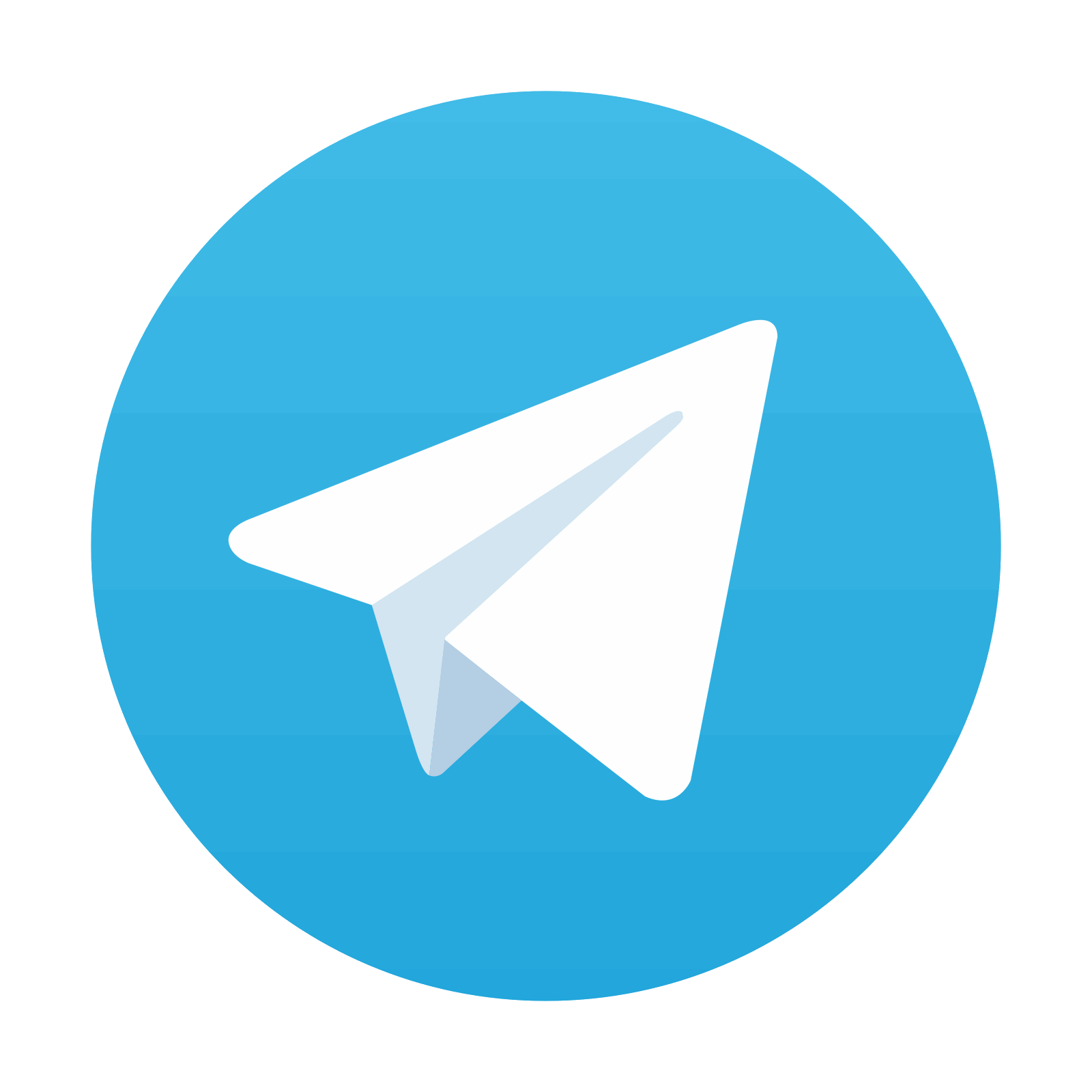
Stay updated, free articles. Join our Telegram channel

Full access? Get Clinical Tree
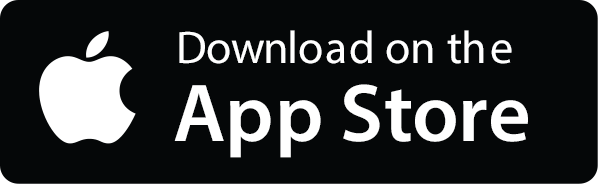
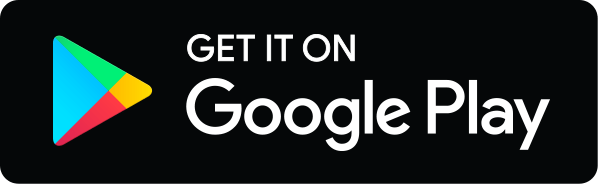