Chapter 18 Overview of Motor Systems
Each of us has fewer than a million motor neurons with which to control muscles. Without them, we would be completely unable to interact with the outside world. With them, however, we are capable of an enormous range of complex activities, from automatic and semiautomatic movements such as postural adjustments to the characteristically human movements involved in speaking and writing. The way in which a wide variety of neural structures interact to make these activities possible is the topic of Chapter 18Chapters 19 to 20.
Each Lower Motor Neuron Innervates a Group of Muscle Fibers, Forming a Motor Unit
Lower motor neurons, the target of central nervous system (CNS) pathways and connections involved in motor control, are arranged in the spinal cord and brainstem in groups corresponding to individual muscles (see Fig. 10-10). The axons of lower motor neurons leave the CNS in ventral roots (or in motor roots of cranial nerves) and divide into terminal branches widely distributed in their target muscles. Each branch ends at the single neuromuscular junction of a muscle fiber (see Figs. 8-1 and 8-11). The combination of one motor neuron and all the muscle fibers it innervates is referred to as a motor unit. Motor units vary tremendously in size, in a way that makes functional sense: the size of the motor units in a given muscle is related to the degree of fine control involved in the use of that muscle. As examples, there may be only 2 or 3 muscle fibers in a motor unit in the stapedius, 10 in an extraocular muscle, 100 in a hand muscle, and 1000 in a large antigravity muscle such as the gastrocnemius (Fig. 18-1). Even within a single muscle, however, motor units vary in size and functional properties, as described shortly.
Lower Motor Neurons Are Arranged Systematically
Just as there are systematic maps in sensory pathways (see Fig. 10-22) and in cortical areas (see Figs. 3-30 and 17-29), so too is there a systematic arrangement of clusters of motor neurons. In the anterior horn of the spinal cord, for example, motor neurons for axial muscles are located medial to those for more distal muscles, and those for flexors are dorsal to those for extensors (Fig. 18-2). This axial-distal mapping corresponds to the arrangement of descending pathways, some of which are important for postural adjustments of axial muscles, and others for the control of distal muscles (see Fig. 18-8).
There Are Three Kinds of Muscle Fibers and Three Kinds of Motor Units
Invertebrates have both excitatory and inhibitory motor neurons, but in vertebrates, all lower motor neurons release acetylcholine onto nicotinic receptors of skeletal muscle. A single action potential in the axon of a lower motor neuron causes the release of acetylcholine at hundreds of active zones, resulting in a single action potential in the postjunctional muscle fiber (see Fig. 8-10). This in turn causes a twitch of the muscle fiber. Hence force production by vertebrate muscle fibers is related to the rate of firing of lower motor neurons: successive twitches sum temporally, much the way excitatory postsynaptic potentials do (Fig. 18-3).
Most muscles are called on to contract for different purposes. The gastrocnemius, for example, must contract weakly but for long periods when we stand upright, more strongly while running (which most of us cannot do for nearly as long as we can stand), and very strongly but very briefly during a jump. Corresponding to these requirements, there are three kinds of skeletal muscle fibers (Fig. 18-4), each populating one of three different types of motor unit (Fig. 18-5). Red fibers (type I) are thin, contain abundant mitochondria, and contract weakly and slowly but are able to sustain contractions for long periods. White fibers (types IIa and IIb) are larger, contain relatively few mitochondria, and contract in briefer, more powerful twitches. Type IIb fibers use glycolysis almost exclusively to fuel their contractions and fatigue very rapidly; type IIa fibers use a combination of oxidative metabolism and glycolysis and fatigue at intermediate rates. Most muscles contain all three fiber types randomly intermingled with one another, but in proportions that vary depending on the principal function of a given muscle.*
All the muscle fibers in a motor unit are of a single type, with the result that there are three types of motor unit (Fig. 18-5). The smallest lower motor neurons innervate type I fibers, the largest innervate type IIb fibers, and motor neurons of intermediate size innervate type IIa fibers. The properties of each motor unit type can be predicted from the properties of the muscle fibers: type S (slow-twitch) motor units produce small amounts of force for prolonged periods, type FF (fast-twitch, fatigable) units produce large amounts of force for brief periods, and type FR (fast-twitch, fatigue-resistant) produce moderate amounts of force that can be sustained for moderate amounts of time (Fig. 18-5).
Motor Units Are Recruited in Order of Size
The association of different muscle fiber types with motor neurons of different sizes is the basis of an elegantly simple mechanism for grading the force of muscle contraction. If two neurons have the same density of channels in their surface membranes, the smaller of the two neurons will have fewer total channels and a greater resistance to transmembrane current flow. Hence a given amount of synaptic current will cause a greater membrane potential change in the smaller neuron, making the smaller neuron more easily excitable. As the synaptic drive reaching the anterior horn increases, motor neurons reach threshold in order of increasing size (the size principle). S units are recruited first, and as they fire faster and faster, FR units are added. As the FR units increase their firing rate, FF units are added. This sequence is required to smoothly increase the force of muscle contraction, beginning with small increases from the background level of tone and ending with brief maximal contractions (Fig. 18-6). The elegant part is that it happens automatically, in all movements, simply by virtue of the increasing size of the motor neurons in the three types of motor unit.
Motor Control Systems Involve Both Hierarchical and Parallel Connections
The inputs that determine the level of activity of lower motor neurons can be divided very broadly into three overlapping classes (Fig. 18-7):
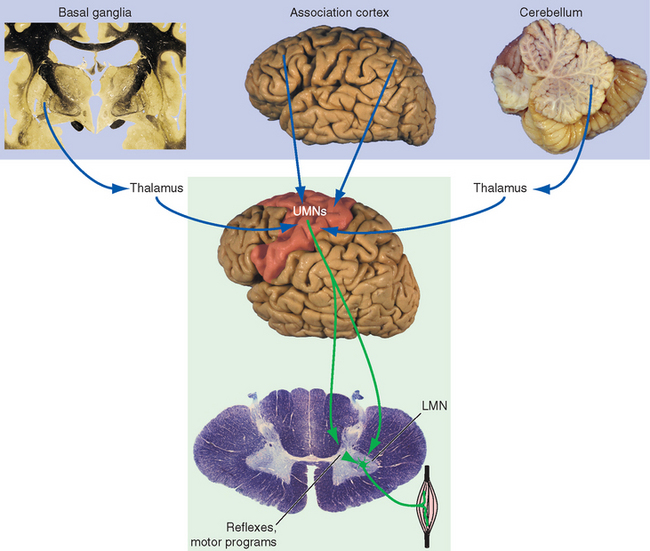
Figure 18-7 Major components and schematic connections involved in motor control. The cerebellum and basal ganglia participate in motor control primarily by influencing the output from cerebral cortex to the brainstem and spinal cord. (These connections are discussed in greater detail in Chapters 19 and 20.) Each also has additional outputs to brainstem nuclei (relatively minor for the basal ganglia, more substantial for the cerebellum). The association cortex, basal ganglia, and cerebellum play vital roles in the choice, design, and monitoring of movement but have no direct effect on lower motor neurons (LMN). For this reason, damage to structures in the lower box but not the upper box causes movement disorders in which weakness is prominent. (As indicated in Fig. 18-8, not all upper motor neurons [UMNs] live in the cerebral cortex.)
Reflex and Motor Program Connections Provide Some of the Inputs to Lower Motor Neurons
The stretch reflex is an obvious and simple example of a built-in pattern of neural connections that controls, to some extent, the activity of motor neurons. Stretching a muscle stimulates its muscle spindles, whose afferent fibers end on motor neurons, which in turn causes the muscle to contract (see Fig. 10-11). Other reflexes, such as the flexor reflex (see Figs. 10-13 and 10-15), are more complex and involve a number of muscles and spinal segments. Finally, there are networks of interneurons in the brainstem and spinal cord that can act as pattern generators for rhythmic movements such as breathing and walking. Although some of the same interneurons involved in reflexes may also be part of the circuitry of these motor programs or central pattern generators, these programs are more than simply a stringing together of reflexes, each one triggering the next. One indication of this is the observation that the principal features of central pattern generators can persist in the absence of afferent input. As an extreme example, the spinal cord of a lamprey (a primitive, jawless fish) can be kept alive in a dish for several days. Such a spinal cord, completely isolated from the rest of the lamprey, can exhibit in its ventral roots oscillating bursts of action potentials that in an intact animal would produce rhythmic, coordinated swimming movements. Upper motor neurons and higher centers harness the basic elements of these central pattern generators and adapt them as necessary—for example, modifying a stepping cycle to avoid an obstacle.
Upper Motor Neurons Control Lower Motor Neurons Both Directly and Indirectly
Upper motor neurons, whose axons descend to the spinal cord (and to cranial nerve motor nuclei) to affect the activity of lower motor neurons, are located in both the cerebral cortex and the brainstem. The descending pathways involved, most of which have already been mentioned, are summarized in Figure 18-8. The vestibulospinal tracts (see Fig. 14-29) are important mediators of postural adjustments and head movements. The corticospinal tract (see Fig. 10-24) has traditionally been considered the principal mediator of voluntary movement, although, as discussed in this chapter, its real role is currently not entirely clear. The reticulospinal tracts (see Fig. 11-19) and, to a lesser extent, the rubrospinal tract are the principal alternative routes for the mediation of voluntary movement. The rubrospinal tract originates in the red nucleus, crosses to the other side of the midbrain, descends in the lateral part of the brainstem tegmentum, and travels through the lateral funiculus of the spinal cord along with the lateral corticospinal tract. The rubrospinal tract of humans is very small, and the reticulospinal tracts are the major alternative routes to the spinal cord. A tectospinal tract has also been described, descending from the superior colliculus through the contralateral anterior funiculus to cervical levels of the spinal cord. It is assumed to be important in reflex turning of the head in response to visual and perhaps other stimuli, but little is actually known about its function in humans.
Association Cortex, the Cerebellum, and the Basal Ganglia Modulate Motor Cortex
Even though corticospinal, rubrospinal, reticulospinal, and vestibulospinal fibers are able to influence motor neurons and their local connections, this still does not explain how a voluntary movement is made. At the present time, the nature of the “little person” within the CNS who pulls the strings when we decide to move is not entirely clear. We can, however, specify some of the structures and connections that must be involved, because damage to these structures and connections results in defective movements (Fig. 18-7). In addition to the portions of the CNS already mentioned, these structures include the basal ganglia, the cerebellum, some areas of association cortex, and portions of the thalamus. The basal ganglia and the cerebellum are the subjects of Chapters 19 and 20, respectively, but the general way in which the various components of the motor system are interconnected is discussed here briefly. Cortical association areas are discussed in Chapter 22.
In one sense, the components of the motor system are organized hierarchically, as though association areas of cortex “decide” that a movement is called for; premotor areas of the cortex devise a plan for the movement and pass this information on to the motor cortex, which then issues commands to motor neurons either directly or indirectly, by way of nuclei and interneurons of the brainstem and spinal cord. In another sense, the components of the motor system are organized in parallel, much like the sensory pathways: messages are conveyed to the spinal cord not only from motor cortex but also from premotor areas themselves (see Fig. 18-11). The basal ganglia and cerebellum are involved in various aspects of planning and monitoring movements but have few or no outputs of their own to the spinal cord. Rather, they act primarily by affecting motor and premotor cortex.
< div class='tao-gold-member'>
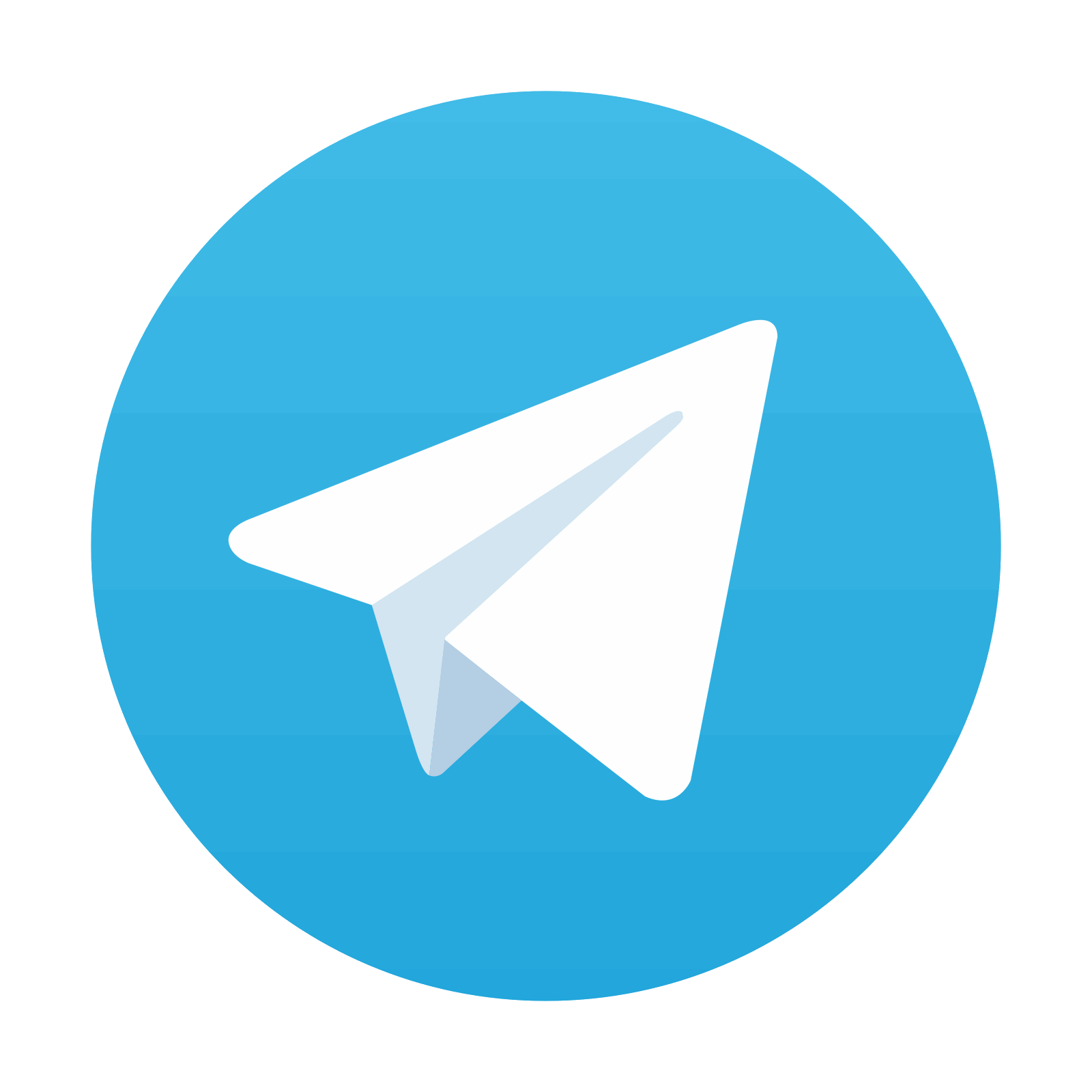
Stay updated, free articles. Join our Telegram channel

Full access? Get Clinical Tree
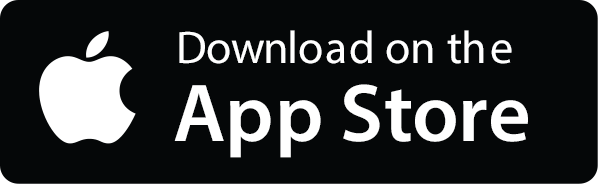
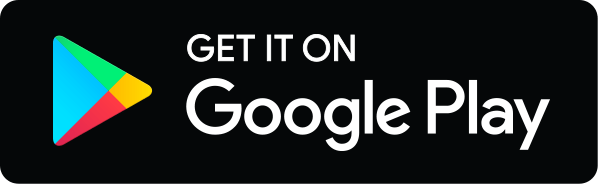