I. NCS and EMG A. Basic neurophysiology 1. Action potential (AP) generation a. Definition: a self-propagating regenerative change in membrane potential b. Originally found to be the result of sodium/potassium channels via voltage clamp experiments on axons of the giant squid; involves maintaining membrane potential at a fixed value and then using channel blockers to test current changes i. Tetrodotoxin blocks Na+ channels ii. Tetraethylammonium blocks K+ channels c. Three phases: resting, depolarizing, repolarizing d. Myelinated are faster than unmyelinated nerves i. Myelin decreases membrane capacitance and conductance and the time constant. ii. Increases the space constant of the segment of axon between the nodes of Ranvier AP propagation in myelinated fibers is known as saltatory conduction. iii. Velocity is proportional to axon radius. 2. Neuromuscular junction a. Presynaptic components i. Motor neuron body (soma) ii. Axon iii. Terminal bouton: synaptic vesicles contain 5,000 to 10,000 molecules (1 quanta) of acetylcholine; release based on voltage-gated calcium channels. b. Synaptic cleft: 200 to 500 µm c. Positive-synaptic components i. Motor end plate ii. Acetylcholine receptors iii. Voltage-gated sodium channels 3. Volume conduction a. Definition: spread of current from the potential source through a conducting medium b. May cause considerable differences in waveforms when a potential travels from one medium to a different medium c. The interstitial fluid and body tissues possess a finite resistance capable of attenuating the magnitude of a potential at a distance from the current source. This diminution in potential magnitude is directly proportional to the square of the distance from the current source and falls approximately 20% in 6.25 cell diameters in neural tissue. d. The amplitude of the recorded potential is dependent on the following: i. Membrane’s charge density ii. Orientation of the recording electrode and active portion of the membrane iii. Distance between the membrane and recording electrode iv. Type of recording electrode used e. The amplitude is also directly proportional to a defined portion of the membrane’s surface area and indirectly proportional to the square of the distance between the membrane and the electrode. i. The amplitude increases if the membrane’s surface area increases or the distance to the membrane decreases. ii. The amplitude of sensory nerve AP (SNAP) or compound muscle AP (CMAP) is the composite of the amplitudes of the individual nerve fibers, some faster or slower than others, resulting in a final amplitude that is less than would be seen if all were summated together in phase. f. The triphasic waveform results from the cathode (negative lead) receiving a wave of depolarization that changes in polarity as it passes and subsequent repolarization occurring later. 4. Far-field recording: recording electrical activity of biologic origin generated at a considerable distance from the recording electrodes a. Usually implies stationary rather than propagating signals recorded at a distance b. Tissue of different density can distort the potential into a complex waveform, with latencies that are different from the actual latencies measured near the generator. c. Changes in extracellular resistance caused by anatomic “inhomogeneities” and/or by conductivity changes give rise to far-field components. 5. Filters and gain a. High and low frequencies i. High-frequency (low pass) filters (HFFs) exclude high frequencies. ii. Low-frequency (high pass) filters (LFFs) exclude low frequencies. b. Gain: recorded in microvolts per centimeter 6. Artifacts a. Physiologic i. Temperature: most negative factor; conduction velocity slows 1.5 to 2.5 m per second for every 1°C drop in temperature, and distal latency prolongs by approximately 0.2 millisecond per degree. ii. Age: newborns have 50% of normal adult nerve conduction velocity (NCV); age 1 year: 75% of normal adult NCV; normal velocities by age 3 to 5 years when complete myelination occurs; upper limb SNAP amplitude drops by up to 50% by age 70 years (lower limb SNAP amplitude may be severely reduced or unevokable by age 60 years); motor unit AP duration is longer in older patients. iii. Height: taller individuals have slower NCVs due to longer (and less thickly myelinated) nerves (adjust to normative data). iv. Proximal versus distal nerve segments: distal segments have slower NCVs due to smaller nerve diameter (including reduced myelin thickness). b. Nonphysiologic: electrode impedance mismatch and 60-Hz interference i. Electrode impedance: minimize by using same electrodes, cleaning skin, using conducting jelly/paste ii. 60 Hz (power-line artifact): if problematic, may be faulty ground; reduced by using a 60-Hz notch filter c. Stimulus artifact i. Cathode position: may not stimulate directly over the nerve (producing submaximal stimulation); may also inadvertently stimulate other nerves ii. Supramaximal stimulation: all nerve fibers must be depolarized (suboptimal stimulation will give lower amplitude) iii. Co-stimulation of adjacent nerves brings in other nerves as artifacts superimposed on desired nerve response iv. Electrode placement: if too distant, may result in distortion from far-field effect v. Antidromic versus orthodromic: antidromic advantage is higher-amplitude potentials; disadvantage is potential for volume-conducting motor potential after the SNAP vi. The distance between recording and referencing electrodes must be greater than 4 cm. vii. Verify accurate distance. B. Equipment 1. Principle a. The clinical EMG is recorded extracellularly from muscle fibers embedded in tissue (conducting medium). b. The ratio of muscle fibers per motor neuron (innervation ratio) is variable—3:1 in extraocular muscles, 1,000+:1 in limb muscles (e.g. 1,000:1 to 2,000:1 for the gastrocnemius). 2. Sources of generators a. Fibrillation and positive sharp waves are from the spontaneous depolarization of a muscle fiber. b. Fasciculation, doublets, multiplets, cramps, and myokymic discharges are from the motor neuron and its axon. c. Complex repetitive discharges (CRDs): result from depolarization of a single muscle fiber followed by nonsynaptic spread to nearby denervated fibers; the depolarization spreads in a “circus” movement back to the original pacemaker muscle fiber (this may be seen with denervation). 3. Technique of needle EMG a. Four steps: insertional and spontaneous activity evaluation, minimal contraction to assess different motor unit potentials (MUPs), and maximal contraction to assess recruitment and interference pattern b. Sensitivity: 50 to 100 µV/cm for insertional and spontaneous activity; 200 µV/cm to 1 mV/cm to assess voluntary activity c. Filter: low, 10 to 20 Hz; high, 10 kHz d. Muscles typically assessed in upper and lower extremities i. Upper extremities (A) First dorsal interosseus (ulnar nerve, C8–T1) (B) Abductor pollicis brevis (median nerve, T1 > C8) (C) Extensor indicis proprius (radial nerve, mostly C8) (D) Flexor pollicis longus (median nerve, C8 > T1) (E) Pronator teres (median nerve, C6–C7) (F) Biceps brachii (musculocutaneous nerve, C5–C6) (G) Triceps (radial nerve, mostly C7) (H) Deltoid (axillary nerve, C5 > C6) (I) Cervical paraspinal muscles ii. Lower extremities (A) Abductor hallucis (tibial nerve, mostly S1) (B) Extensor digiti brevis (deep peroneal nerve, L5 > S1) (C) Tibialis anterior (deep peroneal nerve, mostly L5) and tibialis posterior (tibial nerve, mostly L5) (D) Vastus lateralis or rectus femoris (femoral nerve, mostly L3–L4) (E) Gluteus maximus (inferior gluteal nerve, S1 > L5) and gluteus medius (superior gluteal nerve, L5 > S1) (F) Lumbar and sacral paraspinals 4. Single-fiber EMG a. To determine fiber density and jitter b. Records 300-µm radius c. Amplifier: higher impedance greater than or equal to 100 megaohms d. Sweep faster, higher gain, high-frequency allowed filter C. Needle EMG of normal muscle 1. EMG of normal muscle a. Insertional activity i. Produced by mechanic stimulation of the muscle fibers by the penetrating electrode with muscle at rest ii. Persists for a few hundred milliseconds (typically, <300 ms) iii. Duration slightly exceeds the movement of the electrode. iv. Divided into normal, increased, and decreased insertional activity v. An isolated positive wave may be present at the end of insertional activity in normal muscle. vi. Prolonged insertional activity occurs in two types of normal variants and in denervated muscle and myotonic discharges. (A) Normal variants: short trains of regularly firing positive waves—may be familial or subclinical myotonia; short recurrent bursts of irregularly firing potentials—most often seen in muscular individuals, especially in calf muscles (B) Reduced insertional activity: occurs in periodic paralysis (during paralysis) and with replacement of muscle by connective tissue or fat in myopathies and neurogenic disorders (C) Increased insertional activity: needle movement resulting in any waveform that lasts longer than 300 ms; needle movement may provoke positive waves; may be seen in neuropathic and myopathic conditions: denervated muscle, myotonic disorders, inflammatory myopathies b. Motor end-plate activity i. The end-plate region is the usual place normal resting muscle shows electrical activity when the needle is held in a stationary position. ii. Consists of end-plate noise and end-plate spikes (A) End-plate noise (1) Monophasic, irregular negative potentials with low amplitude (10–50 µV) and 1 to 2 milliseconds in duration. (2) “Ocean”/“sea shell” sound due to depolarization caused by spontaneous release of acetylcholine (3) Biphasic potentials with a negative onset are also a constituent of end-plate noise and have duration of 3 to 5 milliseconds and amplitude of 100 to 200 µV. (4) Biphasic potentials represent muscle fiber APs arising sporadically at the neuromuscular junction or intramuscular nerve fibers. (B) End-plate spikes (1) Amplitude: 100 to 200 µV (2) Duration: 3 to 4 milliseconds (3) Frequency: 5 to 50 Hz irregularly firing (4) Initially negative amplitude (as opposed to fibrillations) (5) Possibly originate in intrafusal muscle fibers c. MUP i. Waveform (A) Composed of a group of muscle fibers innervated by a single anterior horn cell (B) The spatial relation between the needle and the individual muscle fibers plays the greatest role in determining the waveform of the MUP. (C) Composite of the compound potential of the sum of individual APs generated in the few muscle fibers of the unit that are in the range of the EMG needle (D) Cooling of the muscle (from 37°C to 30°C): increase in the duration; decrease in the amplitude; marked increase in the percentage of polyphasic potentials (E) The MUP is made up of less than 20 muscle fibers lying within a 1-mm radius from the electrode tip. (F) MUP waveform (1) Amplitude: typically 200 µV to 3 mV; determined largely by the distance between the recording electrode and the active fibers that are closest to it; computer simulations have suggested that MUP is determined by less than eight fibers situated within 0.5 mm of the electrode. (2) Rise time (a) Time lag from the initial positive peak to the subsequent negative peak (b) Should be less than 500 microseconds (c) Area of negative spike depends on number and diameter of muscle fibers closest to electrode and their temporal dispersion. (d) A crisp sound is associated with minimized rise time (optimal needle electrode position). (e) Distant units have a slower rise time. (3) Duration (a) Relates to anatomic scatter of end plates of the muscle fibers in the units studied (b) Measured from the initial takeoff to the return to the baseline (c) Indicates the degree of synchrony among many individual muscle fibers (d) Varies from 2 to 15 milliseconds depending on the muscle, temperature, and age: decreased temperature causes increased duration and number of polyphasic potentials; increases with age due to increased width of territory of end plates that are scattered (e) Duration is a more reliable parameter in assessing MUP size, and it reflects more accurately all the muscle fibers within the motor unit. (f) Pathologic findings (i) Long duration: seen in lower motor neuron disorders and chronic myositis (ii) Short duration: seen in all myopathies; occasionally in neuromuscular junction disorders and early phases of reinnervation (iii) Polyphasia: five or more phases, seen in myopathic and neurogenic disorders (4) Phases (a) Determined by counting the negative and positive peaks to and from the baseline (b) Normal: less than four (c) More than four suggests desynchronization of discharges or drop out of fibers. (d) May see polyphasic potential in normal muscles, but should not exceed 5% to 15% (e) Desynchronization may be suggested by complex or pseudopolyphasic potentials (potentials that have several turns but do not cross the baseline). d. Recruitment patterns of MUPs i. Recruitment pattern: the relationship between the number of MUPs firing and their firing rate varies between muscles but is constant for a particular muscle. ii. Recruitment frequency: frequency at which a particular unit must fire before another is recruited; 5 to 20 Hz; the ratio of the number of active motor units to the firing frequency of individual units is generally less than 5 and is relatively constant for individual muscles. iii. Motor units are activated according to Henneman’s size principle: early recruited units are usually small type I; larger type 2 fibers are activated later during strong voluntary contractions. iv. Increase in muscle force results in recruitment of previously inactive units; increased firing rate of already active units v. Number of active units less than 10 is indicative of loss of motor unit. vi. Interference pattern (A) Simultaneous activation of multiple motor units precludes the identification of individual motor units—interference pattern. (B) The spike density and the average amplitude are determined by several factors, including descending input, number of motor units capable of firing, firing frequency, waveforms, and phase cancellation. (C) Provides a simple quantitative means of evaluating the relationship between the number of firing units and the muscle force exerted with maximal effort (D) Decreased recruitment (interference) pattern (1) Characterized by a rapid rate of firing of MUPs disproportionate to the number of units firing (2) Caused by any disorder that destroys motor axons or neurons, blocks conduction along motor axons, or devastates (or blocks) a large number of muscle fibers so that many motor units are practically lost (3) Can be seen in acute neuropathic conditions (trauma, infarction, Guillain-Barré syndrome [GBS]), acute demyelinating and axonal loss lesions, Kugelberg-Welander disease; in uncooperative patients, the interference pattern may be reduced during maximal voluntary effort (but CMAPs are normal in configuration). e. Clinical applications i. Denervation (A) Conditions: acquired neuropathy, hereditary neuropathy, plexopathy, radiculopathy (B) Typically long duration, large amplitude (reinnervation), decreased recruitment, ± polyphasia ii. Myopathy (A) Conditions: acquired myopathies, hereditary myopathies (B) Typically short duration, small amplitude, “early” or normal recruitment, polyphasic potentials iii. Spontaneous discharges (A) Fibrillation potentials and positive waves: APs of single muscle fibers that are discharging spontaneously in the absence of innervation (1) Fibrillations (a) Triphasic or biphasic, 1 to 5 milliseconds in duration, and 20 to 200 µV in amplitude; firing rate, 2 to 20 Hz; high pitched, bi- or triphasic; first phase is positive except when recorded in end plate. (b) Muscle fibers that show fibrillation potentials: denervated muscle fibers 3 to 5 weeks after acute lesions (may persist for months or years until muscle fibers are reinnervated or are degenerated) (c) Grading (variable methodologies used) (i) Fibrillations that are not persistent (ii) One or more fibrillations persistent in at least two areas (iii) Two or more persistent fibrillations of moderate numbers in three or more areas (iv) Three or more persistent fibrillations of large numbers but not obscuring the baseline (v) Four or more persistent fibrillations of large numbers that obscure the baseline (2) Positive waves (a) Biphasic: 10 to 30 milliseconds in duration and 20 to 200 µV in amplitude (b) Arising from single fibers that are injured (c) Same significance as fibrillation potentials (B) Myotonia (1) APs of the muscle fibers that are firing spontaneously in a prolonged fashion after external excitation (2) Regular in rhythm but vary in frequency between 40 and 100 per second (3) Occur as brief spikes or positive waveforms (4) Sounds like a dive-bomber (5) Conditions: myotonic disorders (e.g., myotonic dystrophy), acid maltase deficiency (C) Myokymia (1) Spontaneous muscle potentials associated with the fine, worm-like motoric movement (2) Appears as normal MUPs that fire with a fixed pattern and rhythm (3) Burst of 2 to 10 potentials (4) Rate of 40 to 60 Hz (5) Bursts that recur at regular intervals of 0.1 to 10.0 seconds (6) The firing pattern of one potential is unrelated to other potentials. (7) Hyperexcitability of lower motor neuron/peripheral nerve, ephaptic excitation (8) Unaffected by voluntary activity (9) In tetany, similar findings are seen but under voluntary control. (10) Conditions: radiation-induced plexopathy or myelopathy, multiple sclerosis (MS), acute inflammatory demyelinating polyradiculopathy, chronic radiculopathy, entrapment neuropathy, gold intoxication, facial myokymia (MS, brainstem tumor) (D) Complex repetitive discharges (1) APs of groups of muscle fibers discharging spontaneously in near synchrony (2) May be the result of ephaptic activation of groups of adjacent muscle fibers (3) Characterized by abrupt onset and cessation (4) Uniform frequency from 3 to 40 Hz (5) Typically polyphasic with 3 to 10 spike components with amplitudes from 50 to 500 µV and durations up to 50 milliseconds (6) Conditions: typically, those with chronic denervation (E) Cramp potentials (1) Distinguished from other potentials by their firing pattern (2) Fire rapidly from 40 to 60 Hz, usually with abrupt onset and cessation (3) May fire in a sputtering pattern, but typically appear as increasing numbers of potentials that fire at similar rates as the cramp develops and then drop out as the cramp subsides (4) Common in normal patients and usually occur when a shortened muscle is strongly activated (F) Neuromyotonia (1) Manifestation of peripheral nerve hyperexcitability (2) Fire at frequencies of 10 to 300 Hz (3) May decrease in amplitude because of the inability of muscle fibers to maintain discharges at rates lower than 100 Hz (4) May be continuous or recur in bursts (5) Unaffected by voluntary activity and are commonly seen in neurogenic disorders (6) Conditions: hereditary and acquired; may be seen with peripheral neuropathies or after radiation treatment; classically caused by an autoimmune etiology (with potassium channel antibodies, e.g., Isaacs’ syndrome). (G) Fasciculations (1) APs of a group of muscle fibers innervated by an anterior horn cell that discharges in a random fashion (2) Conditions: normal patient, chronic partial denervation, including motor neuron disease D. Pathologic conditions 1. Myopathic disorders a. Similar findings are that of reinnervation after severe axon loss nerve damage (these “nascent” motor units are myopathic-appearing) b. Assess proximal muscles (e.g., iliacus, glutei, spinati, and paraspinous muscle) and midlimb muscles (brachioradialis and tibialis anterior) 2. Muscular dystrophies (MDs) a. Decreased insertional activity when muscle replaced by fatty tissue b. Increased insertional activity, positive waves, fibrillations, and complex repetitive discharges may occur as a result of segmental necrosis of muscle fiber or regeneration of fibers. c. Conditions: Duchenne’s MD, Becker’s MD, Limb-girdle MD, fascioscapulohumeral MD, Emery-Dreifuss MD 3. Inflammatory myopathy a. EMG findings may be patchy. b. EMG myopathic findings especially common in proximal limb +/– paraspinal muscles c. Conditions: polymyositis, dermatomyositis, inclusion body myositis 4. Endocrine and metabolic myopathies a. Hypokalemic periodic paralysis i. Typically normal between attacks ii. During attacks: no abnormal spontaneous activity; decreased duration and number of MUPs, decreased interference pattern, complete electrical silence in severe cases b. Hyperkalemic or normokalemic periodic paralysis i. Increased insertional activity; decreased duration and number of MUPs ii. Myotonic discharges +/– CRDs 5. Drug-related myopathy a. Both myopathic and neuropathic findings i. Cimetidine ii. D-Penicillamine iii. Colchicine iv. Chloroquine b. Myopathic findings only i. Clofibrate ii. Lovastatin iii. Gemfibrozil iv. Niacin c. Acute rhabdomyolysis i. Lovastatin ii. Gemfibrozil 6. Critical illness myopathy a. Classic myopathic-appearing MUPs b. ± Fibrillations (“necrotizing” feature) c. Decreased CMAP 7. Neuropathic disorders a. Immediately after acute nerve injury i. Decreased CMAP under voluntary control ii. No complete interference pattern iii. Increased firing rate of individual units iv. No electrical activity in severe cases b. Reinnervation i. Decreased spontaneous activity and amplitude of CMAP ii. Variable in size and configuration of CMAP iii. Increased duration of CMAP and polyphasia RECRUITMENT MUP APPEARANCE DISORDERS Normal or “early” Short duration, low amplitude, polyphasic Primary myopathies Severe myasthenia gravis Normal Mixed short duration and long duration Chronic myositis Inclusion body myositis Rapidly progressing neurogenic disorder (e.g., amyotrophic lateral sclerosis) Reduced Normal Acute neurogenic lesion Reduced Long duration, polyphasic Chronic neurogenic lesion Reduced Short duration, polyphasic Severe myopathy (end-stage) Early reinnervation after severe nerve damage (with “nascent” units) E. Clinical NCS studies 1. Miscellaneous: maximum difference of NCS latencies between right and left a. Motor: 0.7 millisecond b. Sensory: 0.5 millisecond 2. Neuropathy a. Axonal: decreased amplitude; mild slowing; reduced recruitment; giant MUPs; fibrillation +/− positive wave potentials. Motor NCS Amplitude Decreased Duration Normal or increased Morphology Normal, may include polyphasia Velocity Normal or decreased (>60% normal) Sensory NCS Amplitude Decreased Duration Normal Morphology Normal Velocity Normal or decreased (>60% normal) H-reflex Increased (<150% normal) or absent F-wave Increased (<150% normal) or absent b. Demyelinating: NCV less than 60% of normal; conduction block∗; temporal dispersion∗; distal latency prolongation (∗ = seen in acquired causes) Motor NCS Amplitude Variable Duration Dispersion Shape Normal or multiphasic Velocity Decreased (<60% normal) Sensory NCS Amplitude Variable Duration Increased Shape Decreased Velocity Decreased (<60% normal) H-reflex Increased (>150% normal) F-wave Increased (>150% normal) 3. Reinnervation a. May begin as early as 1 to 2 weeks after injury b. Reinnervation progresses at 1 mm per day 4. Motor nerves typically degenerate at faster rates than sensory nerves. 5. Sensory NCSs are typically better preserved than motor NCVs. 6. Lesion proximal to dorsal root ganglion will produce sensory loss but preservation of sensory NCS. F. H-reflex studies 1. Technique a. Stimulating cathode proximal to avoid anodal block b. Stimulus pulse with long duration (1 millisecond) c. Submaximal stimulus d. Frequency = 0.2 Hz to allow full recovery before next stimulus e. Late response must be larger than the preceding direct motor response. f. Lower extremity H-reflex: posterior tibial nerve at popliteal fossa (PF) recorded on soleus muscle g. Upper extremity H-reflex: flexor carpi radialis muscle via median nerve stimulated at cubital fossa 2. Neurophysiologic significance a. H-reflex involves fast-conducting afferent (Ia) fibers via monosynaptic reflex. b. The tibial H-reflex and Achilles’ reflex (ankle jerk) are interchangeable c. Uses i. It is a standard test available within the routine EMG study to evaluate the preganglionic segment of the sensory fibers of the S1 root. ii. Upper limit of normal latency: soleus—35 milliseconds; flexor carpi radialis— 21 milliseconds iii. Side-to-side difference of latency: 2 milliseconds between lower extremities; 1.5 milliseconds between upper extremities iv. Side-to-side difference of amplitude: less than or equal to 3 milliseconds for both upper and lower extremities v. Note: may be normally absent in the elderly d. Disorders of peripheral nervous system: absence early in GBS; important in plexopathies and radiculopathies; important in C6, C7, or S1 radiculopathies; useful in radiculopathies—showing the injury to the anterior rami even when EMG is unrevealing owing to sparing the ventral roots e. Disorders of central nervous system (CNS): important in CNS lesion with upper motor neuron signs f. Other uses of H-reflex: decreased in cataplexy and acute spinal cord lesion G. F-response 1. Physiology: F-waves are produced by antidromic activation (reflected impulse) of motor neuron; useful to estimate conduction in proximal motor nerves by testing length of entire motor nerve; no synapse is tested. 2. Technique a. Cathodes—proximal b. Supramaximal stimulation c. No need of long duration d. Rate less than 0.5 Hz e. Gain amplifier, 200 to 500 µV; sweep, 5 to 10 milliseconds 3. Clinical application of F-wave a. Normal range i. Upper limits of F-wave latency (A) Hand: 31 milliseconds (B) Calf: 36 milliseconds (C) Foot: 61 milliseconds ii. Maximal side-to-side difference (A) Hand: 2 milliseconds (B) Calf: 3 milliseconds (C) Foot: 4 milliseconds iii. Less than 70% of normal patients do not have peroneal nerve F-wave, but most should have normal tibial nerve F-waves. iv. Disorders of peripheral nervous system (A) Prolonged F-wave latencies (1) Polyneuropathies (2) Amyotrophic lateral sclerosis (3) Myotonic dystrophy (4) GBS and chronic inflammatory demyelinating polyradiculoneuropathy: prominent F-wave slowing compared to distal motor NCV (5) Syringomyelia (6) S1 radiculopathy v. Disorders of CNS (A) Absent in spinal shock, and possibly in other disorders of upper motor neurons
NB:
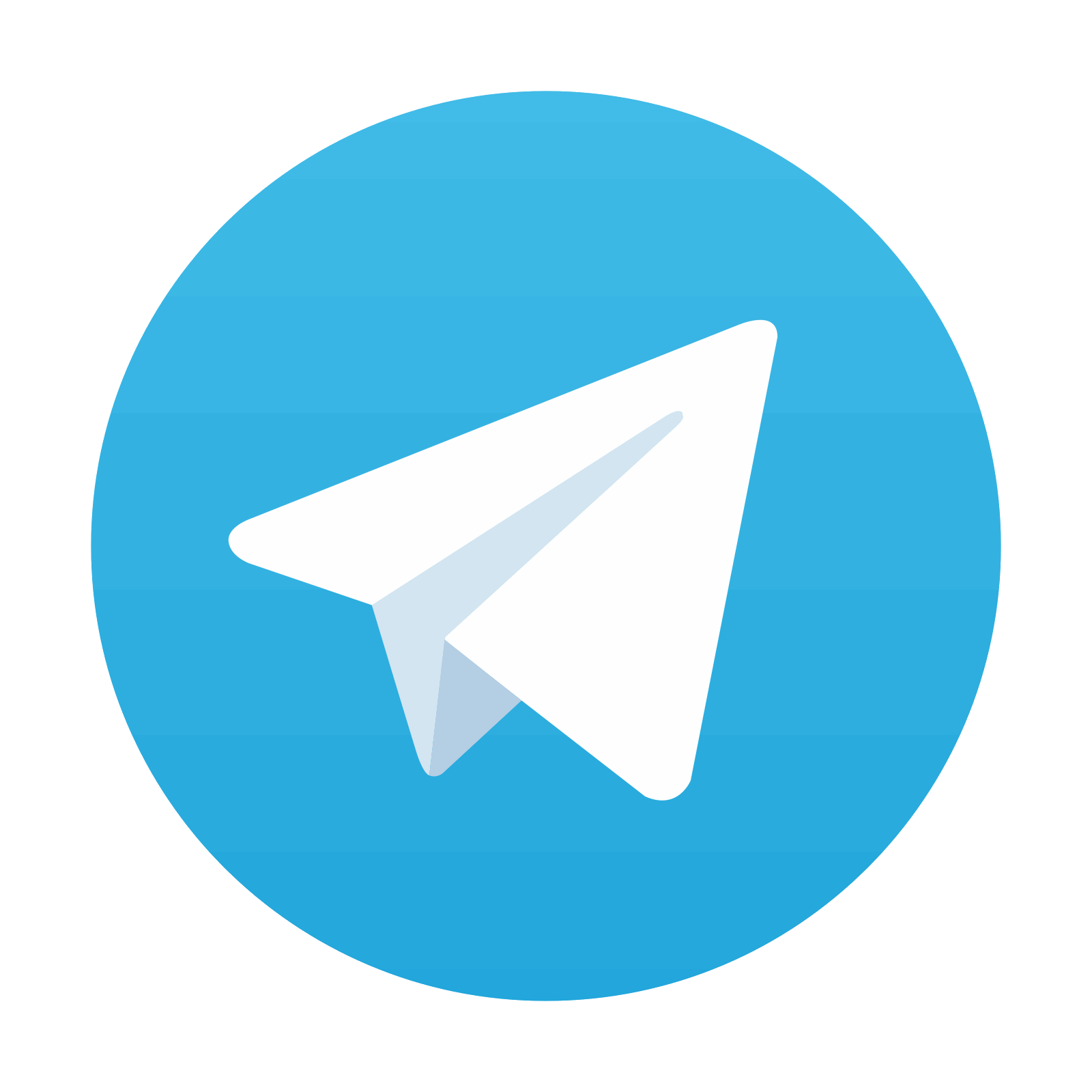
Stay updated, free articles. Join our Telegram channel

Full access? Get Clinical Tree
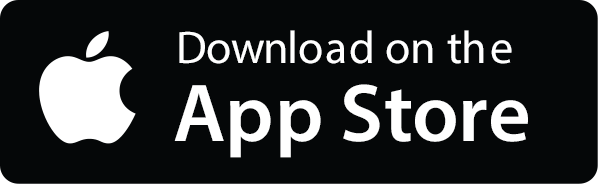
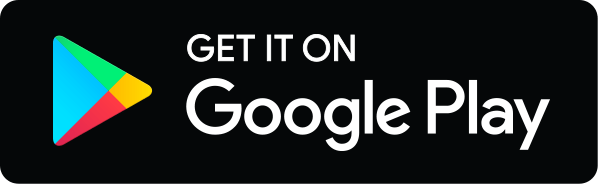