Hormones are signaling molecules that reach their target cells via the bloodstream.
The hypothalamus is the key regulator of the endocrine system by releasing hormones directly into the blood and by regulating the anterior pituitary.
The hypothalamic–neurohypophyseal (posterior pituitary) system secretes two peptide hormones directly into the blood, vasopressin and oxytocin.
To regulate the anterior pituitary, hypothalamic neurons synthesize peptide-releasing and inhibitory factors.
The hypothalamic–pituitary–adrenal (HPA) axis comprises corticotropin-releasing factor (CRF), released by the hypothalamus; adrenocorticotropic hormone (ACTH), released by the anterior pituitary; and glucocorticoids, released by the adrenal cortex.
The hypothalamic–pituitary–thyroid axis consists of hypothalamic thyrotropin-releasing hormone (TRH), the anterior pituitary hormone thyroid-stimulating hormone (TSH), and the thyroid hormones triiodothyronine (T3) and tetraiodothyronine or thyroxine (T4).
The hypothalamic–pituitary–gonadal axis comprises hypothalamic gonadotropin-releasing hormone (GnRH), the anterior pituitary luteinizing hormone (LH) and follicle-stimulating hormone (FSH), and the gonadal steroids.
Prolactin is synthesized and released by the anterior pituitary and triggers lactation in females; its release is inhibited by dopamine acting at D2 dopamine receptors.
Growth hormone, also released by the anterior pituitary, is under the stimulatory control of growth hormone–releasing hormone (GHRH) and inhibitory control of somatostatin.
The hypothalamus contains a complex network of peptide-releasing neurons that regulate feeding and energy expenditure.
Leptin, released by adipocytes, regulates the long-term state of the body’s energy reserves largely via its actions on the hypothalamus.
The arcuate nucleus of the hypothalamus plays a key role in feeding and energy expenditure. It contains one set of neurons that express proopiomelanocortin (POMC) that suppresses feeding and another set that express neuropeptide Y (NPY) that stimulates feeding.
The POMC product, melanocortin (also called α-melanocyte-stimulating hormone) and NPY, in turn, regulate several other feeding peptides (eg, melanin-concentrating hormone) to produce their ultimate effects on food intake and energy metabolism.
The hypothalamus responds to inflammatory cytokines by causing fever and sickness behavior.
The brain controls bodily function and behavior through the motor system, the autonomic nervous system (Chapter 9), and hormones released by neuroendocrine neurons. Hormones are signaling molecules that reach their target cells by means of the bloodstream. They comprise three major classes: peptides, neurotransmitter-like small molecules such as epinephrine (adrenalin), and steroid-like molecules. Through its neuroendocrine system, the brain contributes to body homeostasis, regulates growth and maturation, and facilitates the body’s response to environmental stressors, illness, and injury.
The hypothalamus, located at the base of the diencephalon, is in a good position to integrate information about the state of the organism from diverse sources. It receives information about the external environment from forebrain sensory systems and about noxious stimuli from nociceptors ascending in the spinal cord and brainstem (Chapter 11). The hypothalamus is activated by subcortical circuits that process emotional responses such as fear, reward, and disgust (eg, from ingesting noxious food), and receives information about the internal milieu from brainstem nuclei, and directly from the blood in those regions where the blood–brain barrier gives way to fenestrated capillaries, for example, the median eminence at the base of the hypothalamus and near the circumventricular organs (Chapter 2). The hypothalamus also has reciprocal relationships with monoamine and cholinergic nuclei in the brainstem and basal forebrain that regulate states of arousal and attention (Chapter 6). Because they are lipophilic, circulating steroid hormones from the adrenal cortex, gonads, and adipocytes can cross directly into the brain and bind receptors (Chapter 4) in the hypothalamus and in other brain areas (eg, hippocampus and amygdala), many of which send projections to the hypothalamus.
The hippocampus, amygdala, septal nuclei, and nucleus accumbens, each of which responds to salient environmental events (Chapters 15 and 16), all project to the hypothalamus. Such connections enable adaptive responses; for example, they allow the hypothalamus to direct the release of stress hormones and activate the sympathetic nervous system in response to a threat. Communication between these regions and the hypothalamus is largely reciprocal, which permits information about the internal milieu to influence cognition, emotion, and reward. Thus, for example, detection of low glucose levels might contribute to a trip to the refrigerator. In addition to the many types of afferent signals directed toward the hypothalamus, there are extensive intrahypothalamic connections, which are involved in coordinating the many hormonal and autonomic outputs of this brain region.
The output of the neuroendocrine hypothalamus consists of peptide hormones released into the circulation. Hypothalamic neurons can affect bodily functions directly by releasing vasopressin or oxytocin into the systemic circulation via the posterior pituitary or indirectly via releasing hormones 10–1 into the portal hypophyseal circulation and thence to the anterior pituitary. Neuroendocrine neurons are specialized for the purpose of transducing neural signals into hormonal signals; in response to depolarization, they release peptides into the bloodstream by means of axons that terminate on capillaries rather than at synapses 10–1. These peptides are considered hormones because they travel through the bloodstream to reach their receptors. Many of these same peptides also act as neurotransmitters when they are released from other neurons in the brain and act at synapses.
Releasing Factor | Cellular Origin | Function (Hormones Regulated in Anterior Pituitary) |
---|---|---|
Corticotropin-releasing factor (CRF; also called corticotropin-releasing hormone [CRH]) | Parvocellular neurons of paraventricular nucleus | Stimulates ACTH release (in concert with vasopressin) |
Gonadotropin-releasing hormone (GnRH) | Medial preoptic and arcuate nuclei | Stimulates LH and FSH release |
Growth hormone–releasing hormone (GHRH) | Arcuate nucleus | Stimulates GH release |
Thyrotropin-releasing hormone (TRH) | Parvocellular neurons of paraventricular and anterior hypothalamic nuclei | Stimulates TSH release |
Dopamine | Arcuate nucleus | Inhibits prolactin release |
The hypothalamus and pituitary gland can be seen as an interacting unit 10–2. In humans, the pituitary has two divisions: the neurohypophysis or posterior pituitary, which contains the axon terminals of neurons projecting from the hypothalamus, and the adenohypophysis or anterior pituitary, which is composed primarily of endocrine cells specialized to synthesize and release particular hormones. Rodents, but not humans, also possess a pituitary intermediate lobe.
10–2
Relationship of the hypothalamus and the pituitary gland. The anterior pituitary, or adenohypophysis, receives rich blood flow from the capillaries of the portal hypophyseal system. This system delivers factors 10–1 released by hypothalamic neurons into portal capillaries at the median eminence. The figure shows one such projection, from the tuberal (arcuate) nuclei via the tuberoinfundibular tract to the median eminence. The posterior pituitary, or neurohypophysis, contains axon terminals of neurons projecting from the paraventricular and supraoptic nuclei of the hypothalamus.

In the neurohypophyseal system, the peptide hormones vasopressin and oxytocin are synthesized in magnocellular (large) neurons of the supraoptic nucleus (SON) and paraventricular nucleus (PVN) and transported to capillary-associated nerve terminals in the posterior pituitary, where they are released directly into the systemic circulation. In the adenohypophyseal system, peptide-releasing factors 10–1, which are synthesized in hypothalamic neurons, are transported to nerve terminals in the median eminence; when the hypothalamic neurons are stimulated, peptide hormones are released into the hypophyseal–portal circulation, which drains into the blood vessels of the anterior pituitary. Such peptides act to stimulate or inhibit the release of anterior pituitary hormones into the systemic circulation. After reaching their targets, pituitary hormones cause the release of additional hormones, which subsequently act on diverse tissues of the body. Hypothalamic releasing factors that cause pituitary hormone release also have trophic influences on anterior pituitary cells; likewise, hormones of the anterior pituitary gland have trophic influences on their target cells. Thus, hypersecretion of a hormone can lead to hypertrophy or hyperplasia of target endocrine cells, whereas the absence of a hormone can lead to atrophy.
The hypothalamic–neurohypophyseal system secretes two major peptide hormones: vasopressin, also known as antidiuretic hormone (ADH), and oxytocin. Vasopressin and oxytocin are synthesized in distinct magnocellular neurons of the PVN and SON of the hypothalamus, and are carried by axoplasmic flow to nerve endings in the posterior pituitary. Vasopressin also is synthesized in a distinct subset of parvocellular (small) PVN neurons that project to the median eminence. Vasopressin released from these neurons acts together with corticotropin-releasing factor (CRF; also known as corticotropin-releasing hormone [CRH]) to stimulate release of adrenocorticotropic hormone (ACTH; also known as corticotropin). Like most peptide transmitters, vasopressin and oxytocin are derived from large precursor proteins, which are cleaved to form active peptides. Associated cleavage events also produce carrier proteins, or neurophysins, which are coreleased with the peptides. Actions of vasopressin and oxytocin within the brain, where they act as neuropeptide neurotransmitters, are discussed in Chapter 7.
Release of neurohypophyseal vasopressin occurs in response to central and peripheral blood osmolality and blood pressure. Stimuli such as hemorrhage, pain, stress, and strenuous exercise can lead to vasopressin release. The primary systemic action of vasopressin occurs at the distal nephron within the kidney, where it increases reabsorption of water across the collecting duct epithelium. Many drugs can interfere with vasopressin action. Lithium inhibits vasopressin action by inhibiting the renal V2 vasopressin receptor–linked adenylyl cyclase. Lithium thereby causes increased urine flow and, in some individuals, nephrogenic diabetes insipidus (the production of more than 3 L of urine per day). Other drugs, including nicotine when taken in high doses, may cause a syndrome of inappropriate vasopressin (ADH) secretion (SIADH), which results in water retention and can lead to dilutional hyponatremia severe enough to produce seizures. Vasopressin and oxytocin have additional actions in the nervous system, including regulation of social behaviors such as affiliation (Chapter 7).
Oxytocin acts during parturition to cause uterine contractions. Synthetic forms of this hormone (pitocin) are used to induce labor. Oxytocin also mediates milk letdown in lactating mothers. Nipple stimulation causes oxytocin release, which in turn causes the myoepithelial cells of the nipple to contract, resulting in the ejection of milk. The release of oxytocin is subject to classical conditioning; thus, a lactating mother may experience milk letdown in response to the cry of a baby.
The hypothalamic–pituitary–adrenal (HPA) axis 10–3 produces a cascade of hormones that are central to the stress response 10–1. This cascade begins with CRF synthesized and released by parvocellular neurons of the PVN of the hypothalamus. These neurons project to the median eminence, permitting CRF to stimulate the corticotroph cells of the anterior pituitary to synthesize and release ACTH into the systemic circulation. ACTH then acts on the adrenal cortex to release glucocorticoids, cortisol in humans, and corticosterone in rodents. Glucocorticoids function as stress hormones by causing cells to become catabolic, that is, to break down energy stores in order to release glucose. They also increase arousal and inhibit inflammation. (Inflammation has a metabolic cost, and acute inflammation might impede fight-or-flight behaviors. Inflammatory processes, which may include swelling that limits movement, are nevertheless adaptive as a component of the healing processes after an emergency has passed.)
10–3
The hypothalamic–pituitary–adrenal axis. Corticotropin-releasing factor (CRF) and vasopressin are released from parvocellular neurons of the paraventricular nucleus of the hypothalamus into the hypophyseal portal system and act on the corticotrophs of the anterior pituitary to cause release of adrenocorticotropic hormone (ACTH). ACTH reaches the adrenal cortex by means of the bloodstream, where it stimulates the release of glucocorticoids. In addition to their diverse functions, glucocorticoids, including synthetic forms such as dexamethasone, repress CRF and ACTH synthesis and release. In this manner, glucocorticoids inhibit their own synthesis.

10–1 Stress
Cells, organs, and whole organisms must respond adaptively to changes in their environments if they are to function and ultimately survive. Adaptive responses to environmental challenges are described as homeostasis. Environmental challenges that are extreme, harsh, or aversive are described as stressors. Stressors can be both physical (eg, a wound, extreme cold, or lack of water) and psychological (eg, separation from or death of a parent, a consequential competition). There are large numbers of homeostatic mechanisms that act at the level of individual cells, organs, and organ systems. By controlling neuroendocrine hormone release and the autonomic nervous system, the hypothalamus plays a central coordinating role in the stress response of the whole organism. The result is a combination of physiologic and behavioral responses aimed at maintaining or restoring the internal milieu.
The typical response to a significant acute (phasic) stressor is traditionally called the “fight-or-flight” response. This response involves activation of the sympathetic nervous system, leading to release of epinephrine systemically from the adrenal medulla and norepinephrine at synapses with effector organs such as the heart and vascular smooth muscle, leading, for example, to more rapid and stronger cardiac contractions and elevated blood pressure (Chapter 9). In addition, the fight-or-flight response leads to the release of CRF and vasopressin into the portal hypophyseal circulation, resulting ultimately in release of systemic glucocorticoids from the adrenal cortex, with elevated levels observable within a few minutes, peaking at approximately 30 minutes and declining to basal levels after approximately an hour. Glucocorticoids act together with the sympathetic nervous system to mobilize energy stores for the fight-or-flight response. Stressors also activate widely projecting monoamine and orexin systems (Chapter 6) to increase arousal and vigilance. Glucocorticoids appear to work in concert with monoamines to increase arousal and to encode memories of the circumstances under which the stressor was encountered (eg, see Chapter 15 for a description of the role of norepinephrine in the consolidation of fear-related memories).
While transient stress responses mounted in reaction to phasic physical or psychological threats are adaptive, indeed often critical for survival, during chronic stress, or failure to dampen the stress response when the danger has passed, they may produce illness in their own right. Depression and chronic stress have significant similarities (Chapter 15), and many patients with serious depression have elevated levels of CRF and cortisol secretion. Nonetheless, the precise relationship between stress and depression remains to be worked out.
Basal CRF release is controlled partly by the circadian pacemaker, located in the suprachiasmatic nucleus (SCN) of the hypothalamus in mammals (Chapter 13). Maximum secretion of CRF occurs in the morning in humans near the time of waking; maximum secretion in rodents, which are nocturnal, typically occurs in the early evening. Superimposed on normal circadian patterns of circulating CRF levels is CRF release precipitated by stress. (Stressors also stimulate release of vasopressin by PVN neurons. Like CRF, vasopressin is a secretagogue for ACTH.)
PVN neurons receive stimulatory inputs from the amygdala and inhibitory inputs from the hippocampus (see 10–3). They also receive noradrenergic and other monoamine projections from the brainstem that relay information about the salience of stimuli. The effects of CRF and related peptides are mediated by two distinct G protein–coupled receptors, called CRF1 and CRF2. CRF binds selectively to CRF1; the CRF-like peptide, urocortin 1, binds both CRF1 and CRF2; and urocortins 2 and 3 bind CRF2 (Chapter 7). CRF activates release of ACTH via CRF1. Urocortin 1 appears to mediate stress-induced anorexia. Urocortin 2 relaxes coronary arteries and has been proposed as a treatment for congestive heart failure. CRF1 antagonists have failed in clinical trials as antidepressant or anxiolytic drugs; the CRF1 receptors that are most relevant to the control of emotional behavior are likely located in the amygdala and other limbic brain regions rather than in the pituitary (Chapter 15).
CRF and vasopressin promote the synthesis of proopiomelanocortin (POMC) and release of its peptide derivatives. Glucocorticoids can feed back to inhibit POMC transcription. POMC is a large precursor protein (7–4 in Chapter 7) that gives rise to ACTH as well as β-endorphin and α-melanocyte-stimulating hormone (α-MSH; also called melanocortin). ACTH stimulates the adrenal cortex via Gs-coupled ACTH receptors to synthesize and secrete adrenal glucocorticoids. A cAMP-regulated pathway supports adrenal steroidogenesis by controlling transcription of the major synthetic enzymes. ACTH also stimulates the secretion of other adrenocortical steroids, including aldosterone (a major mineralocorticoid) and adrenal androgens.
Dietary cholesterol is the primary starting material for the biosynthesis of glucocorticoids and other adrenal steroids 10–4. Cholesterol enters one of three biosynthetic pathways in the adrenal cortex, which lead to the production of glucocorticoids (cortisol), mineralocorticoids (aldosterone), and adrenal androgens (eg, dehydroepiandrosterone). Cells within different zones of the adrenal cortex express distinct synthetic enzymes that catalyze the production of these specific hormones 10–2.
10–4
Synthetic pathways of the adrenal steroids aldosterone and cortisol. Synthesis of aldosterone and cortisol occurs in different layers of the adrenal cortex (see 10–2).

Location | Layer | Significant Hormones |
---|---|---|
Deepest | Medulla | Epinephrine |
Deepest cortical layer | Zona reticularis | Adrenal androgens, for example, androstenedione |
Middle cortical layer | Zona fasciculata | Glucocorticoids, for example, cortisol |
Superficial cortical layer | Zona glomerulosa | Mineralocorticoids, for example, aldosterone |
The primary mode of action of all steroid hormones is to bind cytoplasmic receptors that, on activation, translocate to the nucleus to regulate gene expression (Chapter 4). Glucocorticoids bind to two different cytosolic receptors: the glucocorticoid receptor (GR) and mineralocorticoid receptor (MR), both of which are members of the large superfamily of steroid hormone (nuclear) receptors (Chapter 4). Despite their names, the MR has a 10-fold higher affinity for glucocorticoids than the GR. Consequently, MR binding sites may be saturated, even with relatively low levels of circulating glucocorticoids, whereas significant GR binding requires high circulating glucocorticoid levels as might occur with a significant stressor or with peak levels of secretion under circadian control. In neurons that express both receptors, such as the hippocampus and amygdala, heterodimers may form with intermediate affinity.
The GR is expressed in essentially every tissue in the body including the brain, but with different levels of expression. Indeed, the lower-affinity GR is anatomically well positioned to exert feedback control when levels of glucocorticoids are very high because it is concentrated in the PVN itself, in the hippocampus, which feeds back negatively on the PVN indirectly by activating GABAergic inputs, and on brainstem monoamine neurons (Chapter 6) that also project to the PVN.
MR expression is more restricted than GR expression; in addition to the brain, it is expressed in the kidney, gut, and heart. Some actions of GR and MR are DNA binding–dependent and others occur independently of DNA binding. After they bind hormone, these receptors are freed from chaperone proteins including heat shock proteins, and are subsequently translocated to the nucleus 10–5. In the nucleus, MR and GR may bind as homodimers or heterodimers to their specific glucocorticoid response elements (GREs) in the regulatory regions of genes; alternatively, they may inhibit or enhance the functions of other transcription factors by means of protein–protein interactions. Thus, GRs may inhibit the actions of AP-1, CREB, NF-κB, and other transcription factors.
10–5
Glucocorticoid receptor (GR) function. After entering a cell, glucocorticoids bind to their receptors, which subsequently are released from their associations with chaperones such as heat shock proteins (HSP90). Ligand-bound GRs translocate to the nucleus, where they bind as dimers to glucocorticoid response elements (GREs) to activate or repress gene expression. Alternatively, a ligand-bound GR can interact with other transcription factors to repress AP-1- or CREB-mediated transcription. Interaction with STAT5 can enhance the activation of target gene expression (Chapter 4).
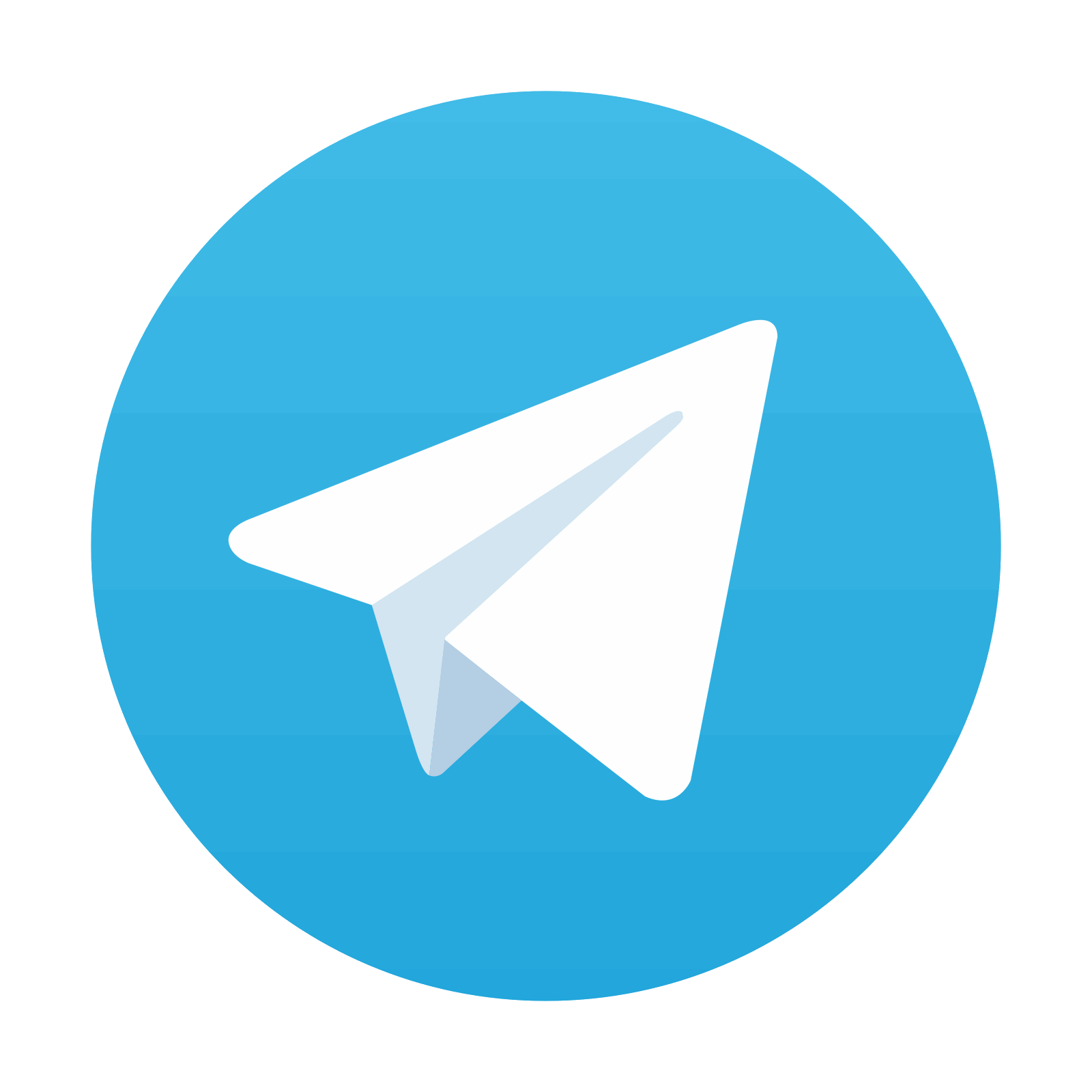
Stay updated, free articles. Join our Telegram channel

Full access? Get Clinical Tree
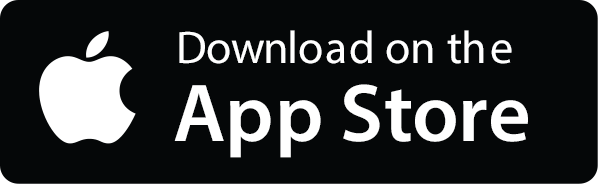
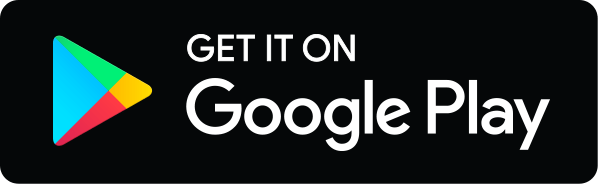
