Abstract
The immediate and dramatic improvements seen seconds after deep brain stimulation (DBS) in a patient with essential tremor or Parkinson disease, combined with the rapid return of symptoms following DBS shutoff, suggest that its mechanism of action involves modulation of existing neuronal circuitry as opposed to the formation of new permanent neuronal connections. Thus, the term neuronal plasticity , traditionally referring to changes in neuronal structure and function that outlast the stimulation period, would not be applicable. Changes in the computational network state, such as disinhibition, rather than sprouting of new axons, can best explain the time course of such changes. In contrast, the delay in symptomatic improvement seen in other forms of neuromodulation therapy is more consistent with other mechanisms of action, namely subacute to chronic formation of new neural networks via mechanisms other than simple computational state changes, which then could be characterized as neuronal plasticity. It is likely, however, that multiple mechanisms of neuromodulation are at play in states of chronic therapy. These mechanisms include representational plasticity, which can be defined as the modification of central nervous system topographic maps (for example, the homuncular mapping in primary somatosensory and motor cortices) owing to changes in neuronal input. This chapter will review what is known about neuronal plasticity, discuss the evidence of plasticity in a number of disease states in which neuromodulation is a potential therapy, and review the evidence of the connection between neuromodulation therapy and neuronal plasticity.
Keywords
Central nervous system, Chronic pain, Cortical plasticity, Movement disorders, Neuromodulation, Neuronal plasticity
Introduction
The immediate and dramatic improvements seen seconds after deep brain stimulation (DBS) in a patient with essential tremor or Parkinson disease, combined with the rapid return of symptoms following DBS shutoff, suggest that its mechanism of action involves modulation of existing neuronal circuitry as opposed to the formation of new permanent neuronal connections. Thus, the term neuronal plasticity , traditionally referring to changes in neuronal structure and function that outlast the stimulation period, would not be applicable ( ). Changes in the computational network state, such as disinhibition, rather than sprouting of new axons, can best explain the time course of such changes. In contrast, the delay in symptomatic improvement seen in other forms of neuromodulation therapy ( ) is more consistent with other mechanisms of action, namely subacute to chronic formation of new neural networks via mechanisms other than simple computational state changes, which then could be characterized as neuronal plasticity. It is likely, however, that multiple mechanisms of neuromodulation are at play in states of chronic therapy. These mechanisms include representational plasticity, which can be defined as the modification of central nervous system topographic maps (for example, the homuncular mapping in primary somatosensory and motor cortices) owing to changes in neuronal input. This chapter will review what is known about neuronal plasticity, discuss the evidence of plasticity in a number of disease states in which neuromodulation is a potential therapy, and review the evidence of the connection between neuromodulation therapy and neuronal plasticity.
Topographic Organization of the Central Nervous System: Historical Overview
In , Penfield and Boldrey reported data obtained via electrical stimulation of the frontoparietal cortex in neurosurgical patients, and described orderly maps of the body present in the precentral motor cortex as well as the postcentral sensory cortex. The well-known homunculi of the precentral and postcentral motor and sensory cortices were further elaborated upon by Penfield and Rasmussen 13 years after the initial reports ( ) ( Fig. 9.1 ).

For two decades subsequently, the consensus was that these maps were static, determined at birth or perhaps during an early critical period of development, and did not significantly change during an individual’s lifetime. In the early 1980s, pioneering work by Merzenich and others demonstrated that, in contrast to previous belief, these maps maintain the ability to reorganize in response to a variety of both peripheral and central perturbations, termed cortical plasticity . Following the transection of the median nerve in owl and squirrel monkeys, the somatosensory cortical territory previously responsive to median nerve inputs became almost immediately responsive to inputs from the uninjured radial and ulnar nerve afferents ( ). Ultimately, over a period of months, an entirely new topographic map emerged, with extensive representations of the radial and ulnar nerve territory of the hand appearing in areas previously responsive to median nerve inputs. Similarly, following amputation of one or two digits in monkeys, the cortical territory originally responsive to these digits became completely occupied by representations of the adjacent skin territories, including the adjacent digits, palmar pads, and digit stumps ( ). Similarly, when digits of monkeys were surgically fused, mapping of the somatosensory cortical area in the subsequent months revealed that the normal sharp discontinuity between the individual digit representations was abolished ( ).
Following upper extremity deafferentation, the upper limb area of the cortex ultimately became responsive to stimulation of the lower part of the face, an intracortical distance of approximately 10 mm ( ). The spatial extent of such large-scale reorganization suggested that multiple mechanisms account for these changes, including:
- 1.
simple computational changes in the relative weights of excitatory and inhibitory inputs to a predefined neural matrix,
- 2.
axonal sprouting,
- 3.
changes in synaptic size, number, and morphology,
- 4
.reorganization at the subcortical level ( ).
The first direct evidence of similar plasticity occurring in humans was demonstrated in 1993 by the author and colleagues, via magnetoencephalography (MEG), a noninvasive method of brain mapping utilizing recordings and localizations of the weak magnetic fields produced by neural activity ( ) ( Fig. 9.2 ). The primary somatosensory cortex representation of the hand was mapped in two adults with syndactyly, both prior to and following surgical separation of the fused digits. Prior to surgery, cortical maps were abnormal, demonstrating shrunken hand representations without the usual somatotopy. Within weeks after digit separation, cortical reorganization was noted to occur, spanning distances of approximately 1 cm, and resulting in appropriate somatotopic representations ( Fig. 9.3 ).


Subsequent functional imaging studies utilizing MEG, and later functional MRI (fMRI), demonstrated evidence of plasticity in patients with a variety of both central and peripheral nervous system injuries, including amputation, spinal cord injury, peripheral nerve injury, and stroke ( ). Reorganization has been demonstrated to occur during the rehabilitation period following neurologic injury ( ). Analogous plastic changes have been demonstrated to occur following short-term motor skill learning ( ), and trained musicians show enlarged representations both of the hand used to play the instrument and in the tonotopic map of the auditory cortex compared with controls ( ).
Neuronal Plasticity in Disease States
A variety of chronic conditions treated with neuromodulation are associated with neuronal plasticity at multiple levels along the neuraxis. Whether this plasticity represents the generator of the pathology or is merely an epiphenomenon remains unclear; nonetheless these findings must be taken into account when postulating mechanisms of action of neuromodulation therapies.
Chronic Pain
demonstrated evidence of cortical reorganization in the primary somatosensory cortex of patients with chronic lower back pain via MEG. Cortical localization of stimulation of the painful area in primary somatosensory cortex S1 was shifted medially in comparison with control subjects. Ramachandran reported psychophysical evidence of cortical reorganization in amputees, with complete somatotopic representations of the amputated upper limb found on adjacent body sides, including the face, chest, and axilla ( ). Using MEG, Flor et al. demonstrated a direct positive correlation between the degree of cortical plasticity and the magnitude of phantom limb pain in patients with upper extremity amputations. Higher pain scores were associated with an elevated shift in the focus of cortical responsivity to tactile stimulation of the face ( ). These results suggest that the phenomenon of phantom limb pain might be a result of maladaptive cortical reorganization, and further suggest that a possible mechanism for neuromodulation’s efficacy may be via adjustment of these already-modified cortical and subcortical sensory and motor representations. Supporting evidence for cortical reorganization’s presence in chronic pain syndromes was reported by in a longitudinal study in patients with complex region pain syndrome. During the acute pain phase, the hemisphere contralateral to the affected upper extremity demonstrated an altered somatotopic organization compared with the contralateral hemisphere. Specifically, the overall cortical representation of the hand was shrunken compared with the contralateral hand. One year later, in patients with decreased pain, the cortical representation of the affected hand had regained its normal spatial extent.
Microelectrode recordings and microstimulation performed in patients undergoing stereotactic neurosurgery have confirmed aberrant thalamic functional organization in patients with chronic pain syndromes ( ). Mapping of the sensory thalamus (ventralis caudalis) nucleus was performed in patients with chronic pain as well as in patients with movement disorders. In nonpain patients, the location of the “projected field,” i.e., that part of the body activated by thalamic stimulation, matched the receptive field of the sensory neurons located at that site. In contrast, there was a high incidence of projected field–receptive field mismatches noted in the chronic pain patients. In an earlier study by the same group, the thalamic representation of the trunk was noted to be significantly larger in patients with deafferentation pain in the leg and foot compared to patients without pain ( ).
This hypothesis, namely that abnormal reorganization is associated with increased pain, has been challenged by studies that demonstrate that improvement in motor function may actually increase phantom pain in phantom limb patients ( ). In this model, a brain–machine interface (BMI) using MEG signals was used to train amputees to move a robotic hand using two different paradigms. Interestingly, although the BMI training with phantom hand task increased the discriminability of the cortical activity, it was associated with an increase , rather than a decrease, in pain. In contrast, BMI training that dissociated the prosthetic and phantom limbs reduced pain.
Movement Disorders
A number of studies have demonstrated abnormalities of the standard cortical and subcortical somatotopic maps in patients with dystonia. Microelectrode recording studies in the globus pallidus and thalamus of patients with dystonia undergoing stereotactic surgery have shown an enlarged sensory representation of the affected limb, with receptive fields that were widened and less specific than reported in normal primates ( ). An fMRI study in patients with focal right-hand dystonia demonstrated an altered somatotopic organization in the left putamen. Furthermore, there was a decreased distance in three-dimensional space between the representations of the hand and the lip in the dystonic patients, which correlated with duration of illness.
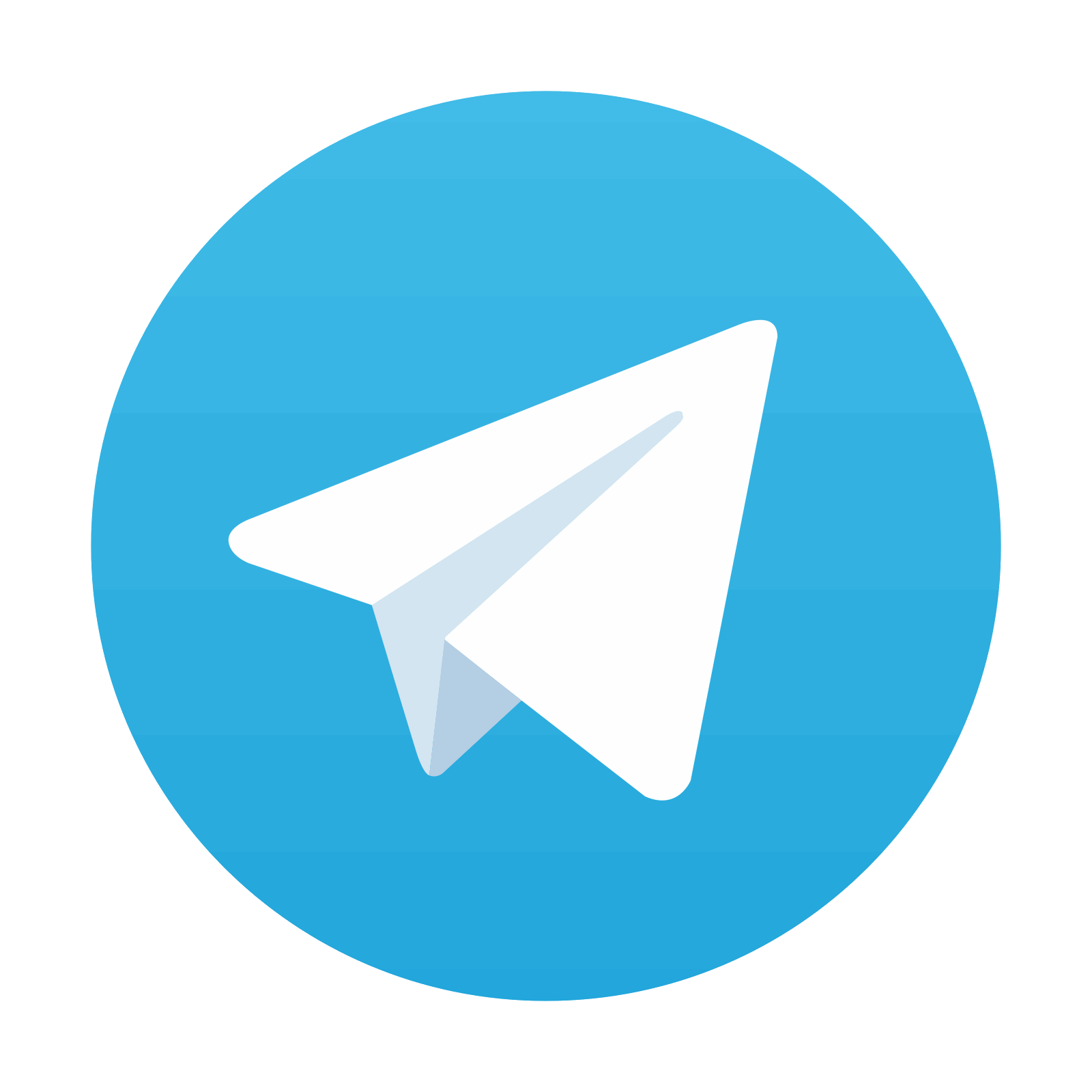
Stay updated, free articles. Join our Telegram channel

Full access? Get Clinical Tree
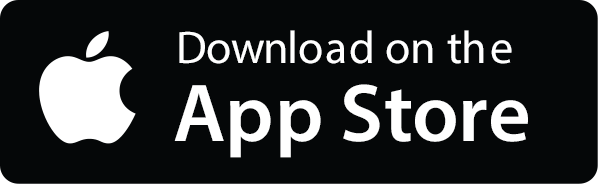
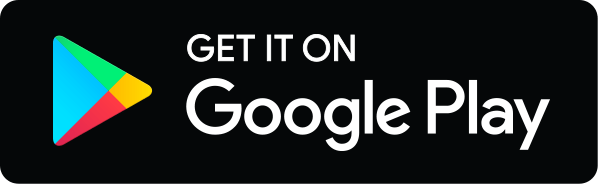