Abstract
Transcranial alternating current stimulation (tACS) and transcranial random noise stimulation (tRNS) are two noninvasive brain stimulation techniques that use electrical currents to modulate brain activity and change behavior. tACS consists of the application of oscillating electrical current to modulate brain activity and tRNS uses random interstimulus-intervals and amplitudes. More precisely, in tRNS, instead of using a fixed frequency and amplitude, the pulses are delivered across several frequencies and amplitudes, distributed across a certain range of the spectrum.
The rationale behind the use of this type of stimulations is that specific brain oscillations have been associated with cognitive and motor functions in healthy and clinical populations and that it is possible to restore disturbed oscillatory activity by applying alternating current externally to the brain. In this chapter, we will provide an overview regarding the basic mechanisms underlying these techniques and their usefulness for understanding cognition/behavior, as well as their potential impact for clinical rehabilitation.
Keywords
Alternating current, Entrainment, Random noise, Stochastic resonance
Outline
Introduction 1611
Transcranial Alternating Current Stimulation 1612
The Effects of Transcranial Alternating Current Stimulation on Cognition 1613
Random Noise Stimulation 1614
A Special Case of Transcranial Random Noise Stimulation: Transcranial Pulsed Current Stimulation 1614
Mechanism of Action 1615
The Effects of Random Noise Stimulation on Cognition 1615
Clinical Effects of Alternating (tACS) and Random Noise (tRNS) Stimulation 1615
Conclusions 1616
References 1616
Introduction
Electrical currents have been used to modulate brain function for several hundreds of years ( ). Rudimentary forms of electrical brain stimulation were introduced by Luigi Rolando, Gustrav Fritsch, and Eduard Hitzing to study the localization of brain function in animals ( ). From then on, the interest in using electrical stimulation to treat neurological and psychiatric disorders increased rapidly, due to its safety profile and promising results.
Transcranial electrical stimulation (tES) refers to a group of noninvasive brain stimulation techniques in the form of direct current (DC), alternating current (AC), and random noise (RN) stimulation ( ) that uses electrical current to modulate the brain. Direct current stimulation (such as transcranial direct current stimulation (tDCS)) uses a constant electrical field over time, while AC stimulation (such as transcranial alternating current stimulation (tACS)) uses AC at a certain frequency, and RN stimulation (such as transcranial random noise stimulation (tRNS)) uses RN frequency spectrum. This chapter will focus on tACS and tRNS.
Since tACS and tRNS operate according to different mechanisms when compared to tDCS, it requires different thinking when designing an experiment. The rationale behind the use of RN and AC stimulations is that specific brain oscillations are associated with cognitive and motor functions in healthy and clinical populations ( ) and that it is possible to restore disturbed oscillatory activity to the brain by applying AC, externally to the brain. Therefore, before designing an experiment with these techniques, it is necessary to identify: (1) the specific patterns of brain oscillation of a given cognitive process; (2) what parameter(s) of oscillations in the brain are directly responsible for the cognitive process, such as frequency, amplitude and phase of brain oscillations ( Fig. 136.1 ) ( ).

The effects of these techniques have been tested in both animals ( ) and human studies ( ). The outcomes in animal studies are usually neural spike rates or coherence between the signal introduced by the stimulation (e.g., a sine wave in AC) and the neural signal ( ). In human studies, outcomes are usually either behavioral (e.g., performance in specific motor or cognitive tasks, accuracy, response time, reaction times, etc.) and/or physiological (e.g., electromyogram (EMG), electroencephalogram (EEG), etc.). Several studies have tested the effects of these noninvasive brain stimulation (NIBS) techniques by testing the magnitude and time course of is effects on motor cortex excitability using another stimulation technique called transcranial magnetic stimulation (TMS) over primary motor cortex (M1). Single-pulse TMS is able to elicit motor-evoked potentials in EMG recordings and assess changes in cortical excitability after alternating/RN (tACS/tRNS) intervention. EEG allows us to detect abnormalities that are related to electrical activity in the brain, in specific bands (e.g., Delta <4 Hz; Theta 4–7 Hz; Alpha 8–15 Hz, Beta 16–31 Hz, Gamma >32 Hz, Mu 8–12 Hz). The mechanism by which this neurophysiologic assessment is able to reflect changes in cortical activity and connectivity is based on its ability to detect oscillatory electrical activity that takes place in superficial cortical layers.
Transcranial Alternating Current Stimulation
TACS, also known as cranial electrotherapy stimulation is a NIBS technique that consists of the application of oscillating electrical current to modulate cortical excitability and brain activity ( ). This technique is relatively new and has been tested in both healthy ( ) and in several clinical populations suffering from various conditions such as Parkinson’s disease and tinnitus ( ).
The major parameters determining the effects of both tACS and tRNS are frequency or frequency range and intensity. During the half cycle of an AC (tACS) oscillation, one electrode acts as the anode while the other as the cathode. During the other half cycle the pattern reverses, i.e., the former anode now acts as cathode and the former cathode as an anode. The current strength increases and decreases following a half sine wave. Usually currents induced by tACS do not affect cortical membrane excitability over sustained intervals of time; however, its effects during the brief phases of depolarization and hyperpolarization on each half cycle may induce online effects through entrainment. This entrainment process refers to the fact that synchronous activity from several cortical neurons adjust to the periodical signaling of external stimuli, such as repeating lights or sounds.
Although this technique can be administered with different waveforms (such as square waves and sawtooth waves), most studies have tested the effects of alternating sinusoidal waves ( ). A study by used AC consisting of sawtooth waves to compare positive and negative ramping, with simultaneous EEG recordings and found alpha oscillations, both during and after stimulation. Additionally, these authors observed that only the positive ramp, sawtooth waveform significantly enhanced alpha power during tACS, when compared to sham.
TACS is useful in modulating subcortical neural circuits and as a tool for enhancing motor skills and cognitive function ( ). Some of the physiologic mechanisms postulated for these clinical effects are associated with the release of increased amounts of neurotransmitters, specifically serotonin, beta endorphin, and norepinephrine ( ). Considering the safety profile and promising results from previous studies, more studies are needed to optimize the effects and explore new parameters of stimulation.
Studies With Animals
Since tACS uses a very weak electric field, one of the main questions in this field of study is whether the intensity of this stimulation is enough to produce changes in neural activity of humans. This issue is frequently addressed by testing different parameters of stimulation in animal studies. However, the data needs to be handled with caution since it is still not clear if these data can be translated to humans. Testing different parameters of stimulation (intensity, duration, and frequency) allows us to study different effects on behavior; however, it is not enough to estimate the effects on neural populations. The orientation and the geometry of the neurons relative to the applied electrical field will affect the polarization of the neurons differently ( ). Polarization seems to be maximum when neurons are parallel to the electrical field; and minimum or nonexistent when neurons are oriented perpendicularly to the electrical field. When the applied currents follow the direction from axon to dendrites the neuron is hyperpolarized and when the currents follow the direction from dendrites to axon, the neuron is depolarized ( ). Therefore, the relative orientation of the electrical field to the geometry of the neuron determines the effects of stimulation on brain activity, i.e., depolarization (anodal stimulation) or hyperpolarization (cathodal stimulation).
Despite the fact that animal models are not precise enough to estimate the effects of tACS on humans, they are invaluable in determining the minimum electrical AC field that is necessary to induce neural changes. In one study by , AC stimulation of a threshold of 0.2 mV/mm, performed in slices of rat hippocampus, was able to modulate ongoing neural activity. In another study by AC stimulations of a threshold of 0.5 mV/mm were able to modulate the neural activity in ferrets ( Mustela putorius furo ) brain slices.
Animal models can also help determining the amount of externally applied electrical currents that reach the cortex. In one study by , the skull of anesthetized rats was stimulated with AC, while intracranial neural activity was recorded. The data suggested that there was entrainment of ongoing activity at frequencies between 0.8 and 1.7 Hz in several cortical areas. The authors concluded that AC stimulation may be able to alter membrane potentials and unit activity and that voltage gradients of 1 mV/mm in the extracellular space affects the discharge probability of neurons.
Mechanism of Action
Transcranial alternating current stimulation (tACS) induces AC fields in the brain ( ), and if applied within the EEG range, it is thought to entrain with or synchronize neuronal networks, thus inducing specific changes in ongoing, oscillatory brain activity. Quantification of such changes can be performed through different methods that include neuroimaging and quantitative electroencephalography (EEG). tACS is expected to synchronize if a single frequency is used or to desynchronize, instead, if several frequencies are used ( ). Although some studies have explored quantitative EEG changes before and after tACS application ( ), minimal literature exists on quantitative EEG changes during the application of tACS ( ). The mechanism by which this neurophysiologic assessment is able to reflect changes in cortical activity and connectivity is based on its ability to detect oscillatory electrical activity that takes place in superficial cortical layers.
On the other hand, the activity of neuronal networks in the mammalian forebrain demonstrates several oscillatory bands, covering frequencies from approximately 0.05–500 Hz. The mean frequencies of the experimentally observed oscillator categories form a linear progression on a natural logarithmic scale with a constant ratio between neighboring frequencies, leading to the separation of frequency bands. Neighboring frequency bands within the same neuronal network are typically associated with different brain states and compete with each other ( ). On the other hand, several rhythms can temporally coexist in the same or different structures and interact with each other ( ). The power density of EEG or local field potential is inversely proportional to frequency ( f ) in the mammalian cortex. This 1/f power relationship implies that perturbations occurring at slow frequencies can cause a cascade of energy dissipation at higher frequencies ( ) and those widespread slow oscillations modulate faster local events. These properties of neuronal oscillators are the result of the physical architecture of neuronal networks and the limited speed of neuronal communication, due to axon conduction and synaptic delays ( ). Because most neuronal connections are local ( ), the period of oscillation is constrained by the size of the neuronal pool engaged in a given cycle.
The Effects of Transcranial Alternating Current Stimulation on Cognition
As mentioned earlier, the mechanisms of action by which tACS operates is rather different from those that operate in transcranial direct current stimulation (tDCS). Different cognitive processes are associated with variations in amplitude, frequency, and phase of brain oscillations. For instance, studies have identified that different amplitudes of EEG alpha oscillations are closely related to visual perceptions and determine whether or not a visual stimulus at near-threshold is processed and perceived by human subjects ( ).
Specific, cortical, synchronous oscillations, in the firing rates of large populations of neurons, have been associated with some cognitive functions ( ). These oscillations and fluctuations in electrical field potentials are recorded during EEG and represent forms of organization and synchronization of brain activity ( ). Hence, several studies have determined that specific oscillatory frequencies are associated with specific tasks, areas, and states, representing specific structural and functional organizations of the neuronal networks ( ).
Alpha oscillations (8–13 Hz) have been associated with pulsed inhibition ( ) and hypothesized as reflecting reduced processing capacities (i.e., inhibition) of a given brain area ( ). For instance, over the occipitoparietal areas, alpha oscillations seem to facilitate functional inhibition of task-irrelevant visual processing ( ). Different attention processes (such as holding and shifting attention) have been associated with synchronization of different frequencies ( ). For instance, increased oscillatory synchronization between frontal and parietal areas seem to be involved in orienting attention in multiple frequency bands ( ) and manipulation of information in working memory ( ).
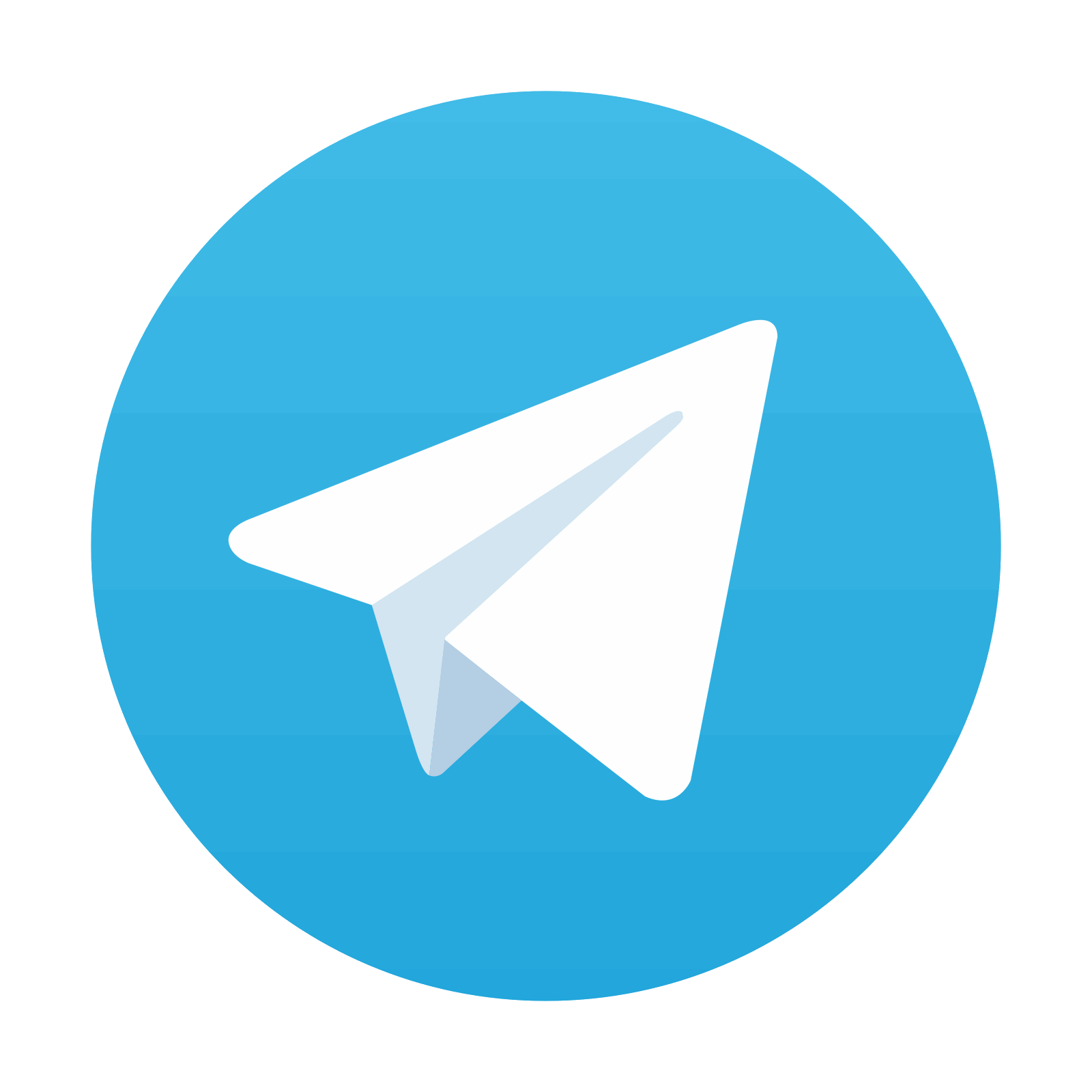
Stay updated, free articles. Join our Telegram channel

Full access? Get Clinical Tree
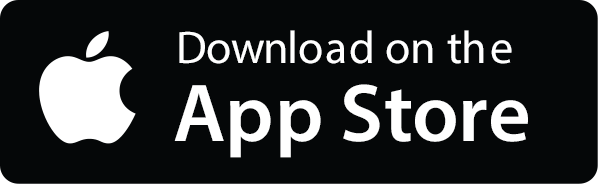
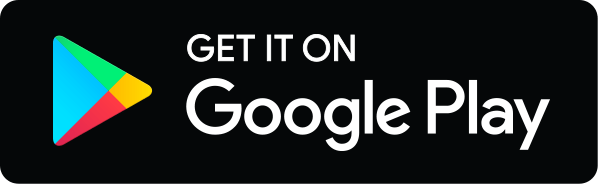