Abstract
Epilepsy is one of the top three most prevalent neurological disorders worldwide, together with headache and cerebrovascular diseases. Epilepsy is defined as a disease of the brain characterized by an enduring predisposition to generate epileptic seizures, and by the neurobiological, cognitive, psychological, and social consequences of this condition. An epileptic seizure is defined as a transient occurrence of signs and/or symptoms due to abnormal excessive or synchronous neuronal activity of the brain. This chapter describes the diagnosis, pathophysiology, and variability of epilepsy, seizures, and seizure syndromes. Temporal lobe epilepsy is one of the most frequent forms of epilepsy and is discussed in more detail. The prognosis and treatment of epilepsy are briefly described, as well as the occurrence of drug-resistant epilepsy and the application of novel therapeutic approaches such as neurostimulation.
Keywords
Diagnosis, Drug-resistant epilepsy, Electroencephalography, Epilepsy, Neurostimulation, Pathophysiology, Treatment
Introduction
The first description of an epileptic seizure was written in Akkadian, the oldest written language, which dates from about 3000 years ago in Mesopotamia. The seizure was attributed to the god of the moon. Further anecdotal cases of epilepsy were found in Egyptian, Chinese, Indian, and Babylonic writings ( ). In ancient history epilepsy was believed to be caused by spirits or gods. In 400 BC Hippocrates (460–375 BC), the father of medicine, wrote his book “On the sacred disease” in which he rejected this theory. He was the first to describe epilepsy as a brain disease, and stated ( ): It is thus with regard to the disease called Sacred: it appears to me to be nowise more divine nor more sacred than other diseases, but has a natural cause from the originates like other affections.
However, his theory of the etiology of epilepsy as a superfluity of phlegm leading to abnormal brain consistency was incorrect. Hippocrates’ book was initially not translated into Latin, and after the fall of the Roman Empire epileptic seizures were again wrapped in a veil of superstition and mysticism. Translation of classical Greek and Arabic texts into Latin and the establishment of medical studies influenced the beliefs of medieval scholastic medicine. Physicians became familiar with the different types of epileptic seizures, but the causative theories for these seizures remained focused on supernatural powers. In the 18th century a growing interest in neuropathology led to the theory that “epileptic seizures arose from structural abnormalities of the brain such as hardening or infection, capable of diverting ‘animal spirits’ from their normal pathways through the brain substance or released an irritant that acted on these spirits to produce seizures.” Outside the medical circuit, beliefs of supernatural influences still persisted ( ).
Thanks to the work of Luigi Galvani (1737–1798), the founder of electrophysiology, further progress in the understanding of the pathophysiology of epilepsy was possible. In 1914 the first photos of an electroencephalogram (EEG) during an epileptic seizure were published. Pioneering work in the field of electrophysiology by Hans Berger (1873–1941) led to the standard use of EEG in diagnosis and treatment of epilepsy. Interaction during the 20th century between clinical neurology and experimental research led to the current understanding of epilepsy ( ).
Today epilepsy is defined by the International League Against Epilepsy (ILAE) as a disease of the brain characterized by an enduring predisposition to generate epileptic seizures, and by the neurobiological, cognitive, psychological, and social consequences of this condition ( ). An epileptic seizure is defined as a transient occurrence of signs and/or symptoms due to abnormal excessive or synchronous neuronal activity of the brain ( ). Because the conceptual definition of epilepsy can be difficult to apply in everyday clinical practice, the ILAE has now proposed a practical definition for epilepsy which requires that any of the following conditions are met: at least two unprovoked (or reflex) seizures occurring more than 24 h apart; one unprovoked (or reflex) seizure and a probability of further seizures similar to the general recurrence risk after two unprovoked seizures (at least 60%) occurring over the next 10 years; and/or diagnosis of an epilepsy syndrome ( ).
Worldwide, the lifetime prevalence of epilepsy is estimated to be about 65 million people ( ). In high-income countries the annual incidence of epilepsy is 46 per 100,000, whereas the prevalence approaches 7 per 1000 people a year ( ). Higher incidence rates occur in low-income countries, rural areas, and among infants and elderly people ( ). About 50% of new epilepsy cases start in those two extremes of life ( Fig. 81.1 ) ( ). In children the majority of newly diagnosed patients are younger than 1 year. In the elderly the major cause is postlesional epilepsy after a cerebrovascular disease ( ).

Between 5% and 10% of the population may experience one single spontaneous seizure during their lifetime. As with epilepsy, single unprovoked seizures predominate in men and in patients less than 12 months and older than 65 years ( ). Patients with a single unprovoked seizure have about a 35% risk of seizure recurrence in the subsequent 5 years. However, about 75% of patients with two or three unprovoked seizures will have further seizures within 4 years ( ). Therefore the diagnosis of epilepsy is made when a second nonprovoked seizure occurs, which is the case in 0.5%–1% of the population ( ). Treatment with antiepileptic drugs (AEDs) is then started.
Diagnosis of Epilepsy
The diagnosis of epilepsy is primarily based on history taking. Anamnesis of the patient is important, but preferably also a description of the seizure from a witness is of great value, since most patients have no recall of the seizure due to an alteration of consciousness. However, even with witness information, it remains a challenge to obtain a correct description of the seizures. The neurologist asks detailed questions about the specific circumstances (occupation at the time of the seizure), provoking factors (e.g., sleep deprivation, alcohol abuse, stress factors), time pattern of the seizures, hormonal influences (menstruation), personal risk factors (e.g., febrile seizures), etc. Also, a detailed family history may contribute to the diagnosis and prognosis.
During physical examination special attention is paid to (sometimes subtle) anomalies indicative of central nervous system (CNS) abnormalities (e.g., asymmetries of fine motor skills and coordination, dysmorphic features for chromosome disorders, and careful examination of the skin for neurocutaneous disorders) ( ).
Besides history taking and standard neurological examination, complementary investigations are performed, such as an EEG and an electrocardiogram (ECG) to rule out cardiac abnormalities ( ). An EEG may reveal typical epileptiformic discharges, which may help to classify the seizure type.
The EEG in patients with epilepsy is characterized by the occurrence of epileptiform activity, i.e., spikes, polyspikes, sharp waves, and spike–wave discharges that can be recorded during (ictal) and in between (interictal) seizures ( Fig. 81.2 ). Recording of habitual seizures during video EEG monitoring is the gold standard for confirmation of the diagnosis of epilepsy and classification of seizure type.

A brain magnetic resonance imaging (MRI) to detect structural epileptogenic lesions is required, except for patients presenting with typical syndromes such as juvenile absence epilepsy or juvenile myoclonic epilepsy ( ). If necessary, further specialized investigations such as positron emission tomography, single photon emission computed tomography, and magnetoencephalography can be performed.
Epilepsy is not a straightforward entity. The term “epilepsy” comprises many syndromes and there may be different underlying causes. Correct diagnosis and classification of the seizure type or epileptic syndrome are indispensable for appropriate treatment and prognosis. The classification is based on the clinical presentation of the seizures combined with the interictal and/or ictal abnormalities on the EEG.
Epileptic seizures are classified into two subtypes according to the networks in which they originate: focal epileptic seizures originate within networks limited to one hemisphere, while generalized epileptic seizures arise within and rapidly engage bilaterally distributed networks. Generalized seizures can be further classified into absence, myoclonic, atonic, clonic, tonic, and tonic–clonic seizures. Focal seizures used to be classified into simple or complex seizures on the basis of presumed changes in the level of consciousness. However, the ILAE recommends abandoning these imprecise terms and instead describing focal seizures according to their clinical manifestations using the “Glossary of Ictal Semiology” (e.g., aura, focal motor, autonomic events, retained/altered responsiveness or awareness) ( ).
The elements that cause epilepsy are multiple and heterogeneous, but they can be used to subcategorize it into epilepsy syndromes, as this aids in the choice of antiepileptic treatments. An epilepsy syndrome is a unique epilepsy condition defined by a typical age of onset, seizure type, and EEG features ( ). Previously the subdivisions of “symptomatic, idiopathic, and cryptogenic” epilepsy syndromes were used, but these terms were poorly delineated and led to confusion. With the current understanding it has been possible to categorize epilepsy syndromes by their specific etiologies, e.g., genetic, structural, metabolic, immune, infectious, and epilepsy syndromes that have no known cause ( ). Genetic epilepsy syndromes are a direct result of a known or presumed genetic defect(s) in which seizures are the core symptom of the disorder (e.g., Angelman syndrome). A small number of inherited epilepsies are “pure” idiopathic epilepsy syndromes ( Table 81.1 ). Almost all mutation occurs in genes that encode voltage-gated or ligand-gated ion channels. It must be clear that a single mutation can give rise to a variety of epilepsy types and a single seizure phenotype can be associated with different mutations. Seizures in structural epilepsy syndromes are attributed to distinct structural brain abnormalities that can be developmental (e.g., tuberous sclerosis) or acquired (e.g., trauma). Metabolic abnormalities are responsible for the increased brain excitability in metabolic epilepsy syndromes, such as in mitochondrial disorders. The increased risk of developing epilepsy in immune epilepsy syndromes is driven by CNS inflammation (e.g., Rasmussen syndrome). The most common epilepsy syndrome worldwide, however, is the infectious syndrome, where infections of the CNS (e.g., bacterial meningitis) can cause both acute symptomatic seizures and epilepsy.
Syndrome | Gene | Gene Product |
---|---|---|
Benign familial neonatal convulsions | KCNQ 2 KCNQ 3 | Voltage-gated potassium channel subunits |
Benign familial neonatal infantile seizures | SCN 2 A | Voltage-gated sodium channel, α subunit, type 2 |
Autosomal-dominant nocturnal frontal lobe epilepsy | CHRNA 4 CHRNB 2 CHRNA 2 | Nicotinic acetylcholine receptor subunits |
Autosomal-dominant lateral temporal lobe epilepsy | LGI 1 | Leucine-rich glioma-inactivated protein |
Generalized epilepsy with febrile seizures plus (GEFS+) | SCN 1 A, SCN 1 B, SCN 2 A GABRG 2 | Voltage-gated sodium channel subunits GABA A receptor, γ subunit, type 2 |
Severe myoclonic epilepsy of infancy | SCN 1 A | Voltage-gated sodium channel, α subunit, type 1 |
Pathophysiology
In the normal brain there is a constant equilibrium between excitatory and inhibitory stimuli. Studies in experimental animal models suggest that the initiation of a seizure is characterized by two concurrent events in an aggregate of neurons: high-frequency bursts of action potentials and hypersynchronization ( ). During an epileptic seizure the normal asynchronous activity of cerebral neurons becomes abnormally synchronized, and the firing characteristics of individual cells take on various stereotypic features, especially bursting patterns. When restricted to only a small portion of cerebral gray matter, this pathologic electrical activity may not lead to clinical manifestations and is considered as an interictal epileptic abnormality. Such asymptomatic localized disturbances may spread to recruit anatomically contiguous gray matter, creating a larger abnormality leading to the clinical manifestations of a seizure with changes in consciousness, motor control, sensory perception, behavior, and/or autonomic dysfunction ( ).
The detailed pathophysiology of epilepsy is incompletely understood and a single mechanism is unlikely, given the diversity of seizure types and causes. Initial steps to investigate the mechanism of epilepsy were based on the study of pathological and surgical specimens, particularly with regard to seizures with a temporal lobe onset, which are often accompanied by hippocampal sclerosis (HS). Studies of morphological abnormalities associated with epilepsy do not explain the development or propagation of seizures. The process of epileptogenesis involves a wide range of changes at the cellular and network levels. In a normal brain the spread of bursting activity is prevented by intact hyperpolarization and a region of surrounding inhibition, created by inhibitory neurons. It is believed that in an epileptic brain there is an alteration of brain function that facilitates hypersynchronous neuronal activity. This may be based on changes in intrinsic mechanisms regulating excitability, e.g. changes in conductance of ion channels, second messenger systems, or protein expression, or altered synaptic function, such as changes in neurotransmitter release or expression of neurotransmitter receptors. Besides neuron-specific alterations, changes in glial function caused by aberrant activation and proliferation of astrocytes and microglia can promote increased excitability and seizures ( ). Similarly, the blood–brain barrier (BBB) is pivotal for neuronal functioning by maintaining brain homeostasis. In that regard BBB damage and subsequent leakage can enhance excitability by stimulating brain inflammation ( ). Moreover, inflammation and oxidative stress are able to increase the excitability of the network ( ). Examples of structural epileptogenic changes are neurodegeneration, astrogliosis, and network reorganization. Together, these changes then pathologically rewire the network, as is particularly evident in HS (see Temporal lobe epilepsy) ( ).
Pathophysiology of Temporal Lobe Epilepsy
Temporal lobe epilepsy (TLE) comprises 30%–35% of all epilepsies and is the most common form of refractory symptomatic epilepsy ( ). Patients suffering from TLE can have simple partial seizures in which they remain conscious. The partial seizures can be divided into different subgroups: focal seizures with changes in muscle activity (simple partial motor seizures), changes in sensation (simple partial sensory seizures), autonomic changes, and psychic changes. More common are complex partial seizures (CPSs) in which the patients lose consciousness and have sensory, mental, visceral, and somatomotor symptoms. A CPS is often preceded by an aura. Although temporal lobe seizures are always partial, they can become secondarily generalized and result into tonic–clonic seizures ( ).
TLE can be categorized in two groups: those with seizures originating in the mesial temporal lobe structures (mesial temporal lobe epilepsy, MTLE) and those with seizures beginning elsewhere in the temporal lobe (e.g., lateral temporal lobe epilepsy, LTLE). There is very little information about LTLE and distinguishing seizure characteristics do not exist ( ). Sufferers from LTLE comprise less than 10% of patients with TLE. Unlike patients with MTLE, there are no reported large series of patients with well-documented lateral temporal lobe seizure origin. This partly explains why LTLE is not well described ( ). Thus in this chapter we refer to MTLE when we describe TLE, unless otherwise stated.
The hypothesis on TLE development covers three phases: an initial precipitating insult of the brain, such as head trauma, status epilepticus, stroke, inflammation, or febrile seizures, initiates a cascade of events; a period of epileptogenesis during which several processes (either molecular or structural) occur; and chronic epilepsy characterized by the occurrence of recurrent seizures.
There is still a lot of debate about the mechanisms underlying development and intractability of TLE, but it is generally believed that limbic structures such as the hippocampus, the amygdala, and the temporal neocortex play crucial roles. One of the reasons for this belief is the fact that surgical removal of these temporal lobe structures eliminates seizures in up to 80% of the cases ( ). Histological studies on tissue obtained surgically from patients with intractable and unilateral TLE reveal specific changes which could underlie hyperexcitability of the removed structures. Typical alterations that are described in temporal lobe regions involve neuronal loss ( ), gliosis, synaptic plasticity ( ), neurogenesis ( ), inflammation ( ), and molecular reorganization in cellular membranes and the extracellular matrix ( ).
HS is the most common lesion observed in patients with refractory TLE and is found in 60%–70% of the cases referred for surgical evaluation ( ). HS is frequently the result of a previous status epilepticus, complicated febrile convulsions, encephalitis, or an ischemic insult ( ). However, seizures themselves can cause or aggravate HS ( ). The hallmark of HS is extensive gliosis combined with a rather selective loss of neurons in the hilus of the dentate gyrus and areas CA1 and CA3 of the hippocampus proper ( Fig. 81.3 ). Granule cells of the dentate gyrus and pyramidal neurons of the area CA2 and the subiculum are relatively spared. Neuronal cell loss involves both glutamatergic excitatory neurons and GABAergic inhibitory interneurons in the dentate gyrus and the hippocampus proper. Sclerosis can extend to other mesiotemporal regions such as the amygdala and the entorhinal, perirhinal, and temporopolar cortex ( ).

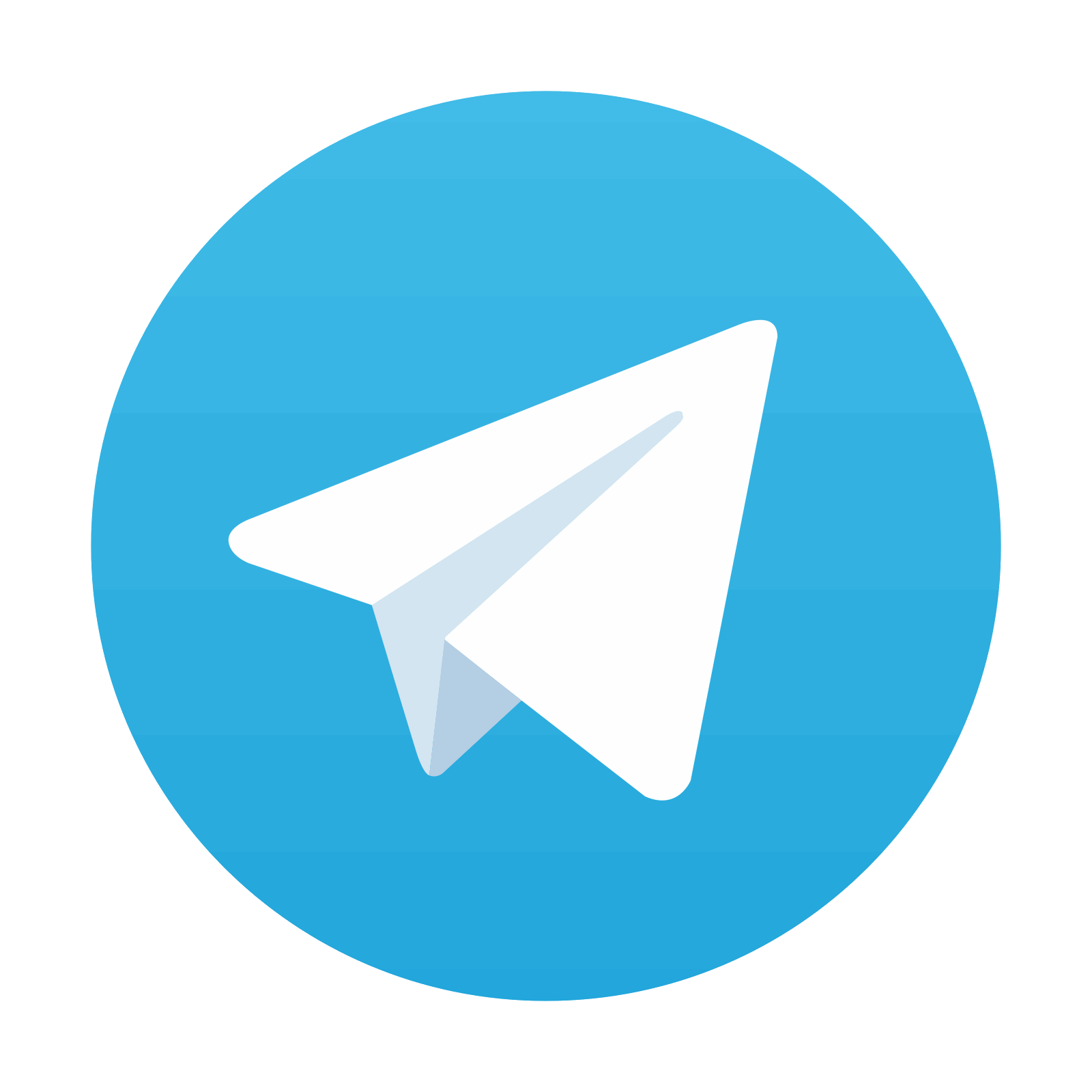
Stay updated, free articles. Join our Telegram channel

Full access? Get Clinical Tree
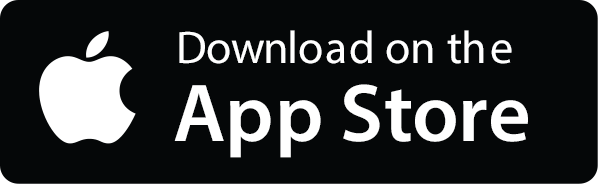
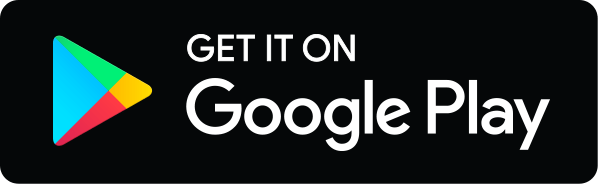
