15 Neuroplasticity and Recovery This chapter surveys the research in neuroplasticity that seeks to explain how structures and functions of the brain change in persons developing typically and persons with neuropathology. This body of research shows that the brain can reorganize in response to experience, training, and environmental demands. Neuroplastic changes in the brain may be optimized when practice and specific goal-directed activities are combined in a meaningful environment. The findings in neuroplasticity differ between adults with mature brains and infants and children with developing brains. However, this research points out that in a model of neurorecovery, such as Neuro-Developmental Treatment (NDT), the timing and intensity of intervention during critical periods of recovery can influence the brain of any age, albeit in different ways. Various studies have demonstrated that therapeutic intervention influences neural reorganization. Constraint-induced movement therapy (CIMT) is one protocol that has been used to show a link between specific intervention strategies and intensity of intervention to neuroplasticity. Examples from that body of literature are presented in this chapter. However, the information from these studies supports many of the assumptions that are the foundations of NDT and are currently part of the NDT Practice Model. Specifically, that well-directed, intense, and well-timed precise intervention strategies can produce permanent changes in the brain, which are reflected in changes in function. The authors state that therapists need to become acquainted with the neuroplasticity research to consider how plasticity may be enhanced or restricted as they plan interventions for their clients. With this in mind, the reader is referred to the case reports in Unit V to link this body of research to the current NDT Practice Model when considering new directions in NDT intervention. Learning Objectives Upon completing this chapter the reader will be able to do the following: • Describe how neuroplasticity is linked to brain development and changes across the life span. • Differentiate between concepts of neuroplasticity in adults with mature brains and infants and children with developing brains. • Describe factors that can enhance neuroplasticity (e.g., repetition, intensity, and environment) and how they can be incorporated in NDT interventions for adults and children. • Describe how the concepts of neuroplasticity can influence the design of NDT intervention. One of the most exciting research topics of the past few decades is the study of brain plasticity and recovery. Plasticity is the ability of the central nervous system to reorganize its structure, connections, and function and its response to intrinsic or extrinsic stimuli throughout life.1 Therefore, the brain and its function change dynamically in response to experience, practice, and the environment. Emerging knowledge regarding neuroplasticity has enormous implications for the rehabilitation of infants, children, and adults who have sustained injuries to the brain. Impaired movement is a major consequence of neuropathology in adults and children.2,3 As described in the International Classification of Functioning, Disability and Health (ICF) model, impaired movement affects a person’s ability to perform functional activities and to participate fully in the home, school, community, and workplace.4 In addition, there are direct and indirect costs associated with conditions such as stroke and cerebral palsy.5,6,7,8 And, as medical care improves, the numbers of infants, children, and adults surviving with long-term neurological sequelae are increasing. Therefore, it is logical that individuals with neurological injury look to habilitation and rehabilitation options, in the form of physical, occupational, and speech therapies to address the effects of their injury and to aid them in achieving a full and productive life. During therapeutic intervention delivery, the variables that practitioners manipulate may impact neuroplasticity positively as well as negatively, and influence functional gains for people with neurological conditions.1,9 Therapeutic interventions, however, are diverse and vary among countries and regions. In the past, the philosophy of therapy has been one of either compensation or functional neurorecovery.10,11 In the compensatory approach to intervention, the therapist minimally incorporates the involved side in the functional activity, teaching the client to rely on the less affected extremity for function. As a result, compensatory movement patterns are developed to take the place of those lost as a result of the injury. One example might be teaching an adult or child with hemiplegia how to eat using only the less involved extremity. In a functional neurorecovery model, such as NDT, the client is guided to use the affected extremities as part of the movement and to recover or relearn movements that were interrupted or lost as a result of the injury. Our understanding of molecular, cellular, synaptic, and network neuroplasticity is currently more advanced with respect to animal models. However, research is in the decisive stages of identifying the mechanisms that lead to neuroplastic changes in the human brain and the application of this knowledge to the intervention for adults and children who have sustained brain lesions or injuries.12,13 For example, the neurorecovery model has been incorporated into activity-based interventions, such as constraint-induced movement therapy (CIMT) and treadmill training, that are specifically designed to enhance function in a specific part of the body. These intensive interventions demonstrate preliminary positive outcomes supported by pre–and post–brain imaging data. As our understanding of brain plasticity grows, we will acquire additional knowledge to modify and create rationales for intervention. In the not too distant future it may be possible to augment neural repair and maximize functional outcomes after brain injuries using pharmacological, rehabilitative, experiential, and environmental paradigms.9 Our understanding of the developing brain and the possible mechanisms of brain recovery, adaptation, and/or compensation secondary to the effects of disease or injury supports and provides insights for the development of NDT interventions for individuals with neurological conditions. There are numerous technologies available to facilitate the study of brain plasticity and recovery in adults and children. The gold standard for visualization of brain structures or the extent of a brain lesion is structural magnetic resonance imaging (MRI). In the infant. it is most often used very early before myelination is fully developed, with follow-up at 18 to 24 months when myelination is complete and the brain structures can be fully visualized. When used in conjunction with diffusion tensor imaging (DTI), the white matter integrity or damage can be better visualized.14 Additional technologies that are employed to study the brain include functional MRI (fMRI), which measures changes in blood flow to detect and monitor brain activation during functional tasks (e.g., motor, language, and somatosensory stimulation) and transcranial magnetic stimulation (TMS) and evoked potentials (EPs) to quantify cortical responses to somatosensory or visual stimuli. However, because active cooperation is required, these technologies cannot be used in newborns, infants, and young children.15 Prior to discussing current research associated with plasticity as it relates to the neurological conditions commonly treated using NDT, it is important to describe models of plasticity associated with recovery in adults and children. This information will aid in understanding key mechanisms that underlie the brain’s ability to respond to injury. To address the broad nature of this topic, we divided this chapter into two neuroplasticity categories—adaptive and developmental plasticity. Adaptive plasticity is an area of neuroplasticity research that addresses how the central nervous system (CNS) maximizes remaining functions and compensates for lost function following brain injury. Research on adaptive plasticity is most advanced with regard to adults with stroke and is reviewed here. More recently, research on adaptive plasticity has expanded our understanding of this process in adults with spinal cord injury,16,17 amputations,18,19 and those with chronic pain.20 Stroke is caused by an occlusion to the blood vessel or a hemorrhage in the brain, resulting in ischemia of the brain tissues (see Chapter 11 on stroke in unit II for additional information). Ischemia sets off a cascade of biochemical reactions leading to inflammatory processes and/or cell death and disintegration of supporting structures within 2 minutes of brain injury.21 Following a stroke, treatments are targeted toward neuroprotection to decrease lesion size, neural survival, motor deficits, and, in turn, disability.22 When neurological structures of the brain are injured, the brain may demonstrate spontaneous recovery, defined as the ability to recover function without intervention. This type of recovery occurs predominantly during the first 3 months following the onset of stroke.23 Spontaneous recovery from stroke is often attributed to resolution of edema and return of circulation in the area surrounding the stroke, called the ischemic penumbra—brain tissue that surrounds the main brain injury site area and is salvageable if intervention is provided within a certain time window to reestablish blood flow to the area.24 In addition, resolution of diaschisis, a depression of blood flow and metabolism of remote sites that are connected to the site of injury, is also believed to play a role in spontaneous recovery.25 See “Time-Sensitive Critical Period” later in this section for a discussion on using the spontaneous recovery period for NDT intervention. Another critical contributor to recovery is neurological reorganization, which occurs at multiple levels of the brain—physiological, anatomical, and functional. Examples of neurological reorganization include changes in excitatory response and efficacy of spared cells, axonal sprouting, dendritic branching, and synaptogenesis. Any of these mechanisms may have the possibility of forming new connections in the cortical areas adjacent to or far from the site of the injury and rerouting and/or unmasking alternative neurological pathways. The process of neural organization known as vicariation may access other structures of the brain to substitute for the function of injured areas.26 There are several patterns of vicariation: (1) functional map extension accesses an area adjacent to the injury by reorganizing it to take over parts of the function of the injured site; (2) homologous region adaptation recruits the contralesional hemisphere to take part of the function of the injured site; and (3) cross-modal reassignment uses structures previously devoted to processing a particular mode of sensory input to accept input from a new sensory modality. This process is reflected in the individual with a visual impairment who learns to read using tactile information. It is widely held that the neural structures which are functionally related to the injured areas can be a key to brain reorganization and support the recovery of function.27 In addition, optimally administered rehabilitation intervention is believed to augment neurological reorganization and enhance the functional outcomes.27,28 Neuroscience research in animal models has contributed to our understanding of the consequences of brain injuries and the possible mechanisms of recovery in humans. However, we are also left with many questions and with the awareness that not all rehabilitation processes result in improved functional outcomes, particularly in the developing brain.9 NDT therapists accept that functional outcome can be altered, for good or bad, through internal and external (i.e., environment, experience) dynamics. These variable dynamics are why NDT strategies are tailored to the individual client and reexamination is part of the NDT Practice Model. In the developing brain, injuries can result from hypoxic, vascular, traumatic, or other etiologies, and the result of the injury is also influenced by location, age, and severity. In almost every case, the injury results in damage or disturbance to the neural networks causing glial and neuronal death, damage to axonal pathways, and disruption of the neurotransmitter and vascular systems.29 Reponses to neural insult, in the form of recovery, include restitution and substitution.30 Restitution proposes that the damaged brain heals and function is restored. Substitution describes the reorganization of the brain through the transfer or reorganization of control to healthy areas of the brain. Diaschisis, one form of restitution, was discussed earlier with respect to recovery in adults. Diaschisis is that period of rapid recovery that immediately follows a brain insult and allows for stabilization of undamaged areas and recovery of functions that have not been destroyed. However, there are also traumatic processes that accompany a brain insult, which may not be well tolerated by the developing brain. Studies of hypoxic ischemic insults in animal models during the perinatal period have shown that cell death can affect the neurotransmitter systems and disturb the balance of the developing brain. Therefore, injuries to the developing brain can result in poorer recovery than described in adults and have caused neuroscientists to consider the fetal and infant brain as structures that are more vulnerable to early injury.14,29 That is, early injury may not result in the same recovery processes described in adults, and the brain’s attempt to recover from injury may cause further damage. With respect to other recovery processes, regeneration, sprouting, and other mechanisms, there is little evidence to support the effect of these processes on the developing brain. With respect to interventions to minimize further brain damage (i.e., brain cooling and pharmacological adjuncts), additional research is needed to determine if these interventions can successfully minimize the effect of early brain injuries and support improved brain plasticity.31 In a review of eight randomized, controlled trials, the findings support the use and effectiveness of therapeutic cooling following hypoxic-ischemic events in newborns.32 However, additional studies are needed to develop specific guidelines for the use of this intervention protocol. Pharmacological interventions show several promising options designed to minimize the effects of injury to the developing brain. For example, antenatal magnesium sulfate administered during preterm deliveries has been shown to reduce mortality and long-term neurological sequelae, but the results are not consistent. Divergent results may be related to the gestational age of the infant, when the drug is administered, and/or the dosage.33 Further studies are needed before this intervention can be considered beneficial in preventing the long-term effects of early brain injury. Finally, plasticity and recovery may also depend on factors such as age at the time of the insult and environmental factors. Animal and human studies show that neural plasticity is present in the adult and infant brain.15,34 Studies of brain insults in infants reveal that structural pathology depends more on the age of the fetus or infant and the developmental stage of the CNS at the time of the injury rather than on the precise nature of the injury itself.35,36 Injuries to the brain that occur in the first and second trimester of the pregnancy influence neuronal proliferation, migration, and cortical organization of the developing brain and result in malformations of the brain structure.14 In the third trimester of pregnancy (i.e., after 24–28 weeks of gestation), brain insults result in gliotic or cystic lesions of the brain, which predominantly manifest as damage to the periventricular white matter and cortical, subcortical, and deep gray matter of the brain.14 Infants are particularly vulnerable to white matter injury between 24 and 36 weeks of gestation, whereas gray matter injuries more commonly occur in the late trimester, after 36 weeks of gestation. In each type of injury, the resultant effect on function is related to which brain structures are disrupted and to the extent of the injury. Corticospinal projections sprout in the motor cortex and migrate away from the brain, creating pathways that will eventually connect the cortex with the brainstem and spinal cord. By 20 weeks of gestation, the corticospinal axons arrive at the level of the cervical spinal cord segments and through synaptogenesis make connections with target cells, predominantly α motor neurons, at each level of the spinal cord.37 During the developmental period of the corticospinal tract (CST), each hemisphere sends ipsilateral and contralateral corticospinal projections to innervate α motor neurons in both the ipsilateral and contralateral segments of the spinal cord. As development continues, this competition resolves, and the contralateral projections are strengthened while ipsilateral projections are withdrawn.38 This process continues in the last trimester and throughout the first year of life. In the case of a brain insult in gray matter, ipsilateral projections from the noninjured hemisphere can play a role in innervating spinal motor neurons on the contralateral side of the injured hemisphere, thus ensuring that corticospinal connections can be achieved even in the absence of projections from the contralateral hemisphere. The presence of these ipsilateral projections may be significant in the reorganization process following a brain injury, particularly in the pre- and perinatal periods, which coincide with the development of ipsilateral and contralateral projections.39 Magnetic resonance imaging (MRI) can be used in children with brain insults to assess the presence of ipsilateral projections arising from the noninvolved cortical hemisphere. Functional hand movements during imaging can reveal whether the movement is associated with activity in the injured or the noninjured hemisphere. This finding would indicate that both of the child’s hands share input from the same hemisphere.15 Another mechanism that can significantly disrupt the CST occurs when the brain injury causes significant white matter damage to the internal capsule (e.g., a periventricular injury). This can damage the crossed corticospinal projections from the motor cortex, as they pass through the internal capsule on their way to the brain stem and spinal cord. In this scenario, the CST projections from the noninjured hemisphere, often detected with MRI, can innervate the spinal cord on the involved side. With regard to hand function and ipsilateral CST projections from the noninjured hemisphere, many children who demonstrate minimal to moderate control of the involved hand and fingers have MRI findings that have traced movement ability back to the ipsilateral CST projections. However, there have been no reports of near-normal hand function supported by ipsilateral CST projections alone.40 However, there are children with ipsilateral projections who have no functional use of the involved hand. This may be in part related to the age at which the injury is sustained. It seems that earlier injuries to the brain result in a prognosis of greater functional use of the hand.41 As a result, despite the presence of ipsilateral projections, there will be some children who will not develop functional use of the hand if the injury to the brain occurs at or around term age. If this information is available to the NDT therapist, it can be useful for program planning and setting realistic outcome goals. The age of the infant at the time of the brain insult influences an infant’s ability to receive afferent sensory information, including tactile and proprioceptive information. Information travels via the posterior column–medial lemniscus pathway to the thalamus and connects via thalamocortical projections through the internal capsule to the sensorimotor cortex. These thalamocortical fibers reach their destination during the third trimester of pregnancy (28–40 weeks of gestation). If a periventricular injury causing damage to the internal capsule is sustained before the onset of or in the early weeks of the third trimester, the thalamocortical projections can bypass the injured area and reach the primary sensorimotor cortex.42 When damage to the internal capsule occurs later in the third trimester or during the perinatal period, the path of the thalamocortical projections through the internal capsule has been completed and those neuronal projections will likely be damaged together with the internal capsule, thus interfering with their ability to reach the sensorimotor cortex.43 Confirmation of such reorganization of the sensorimotor system gives rise to an additional hypothesis regarding restructuring of the brain following early-gestation-stage injury.42,43 This information is important to therapists because it implies that the delivery of sensory information may be perceived and processed differently depending on both the type and the age of the injury. The evidence also implies that some infants who sustain brain insults at the end of the third trimester or during the perinatal and postnatal periods may have significant impairments to the sensory system, which should be considered when planning intervention. Therapists might consider using additional sensory information (e.g., visual and/or verbal cues) to augment or replace the feedback normally acquired through the tactile sensory input. Another form of the reorganization of the sensorimotor cortex has been studied with respect to middle cerebral artery (MCA) injuries, which affect adjacent corticosubcortical areas of the brain, particularly those injuries that cause damage to the postcentral gyrus where the sensorimotor cortex resides. To date, there has been no evidence to support cortical reorganization in these areas following brain lesions resulting from damage to the MCA.42,44
15.1 Introduction
15.2 How Is Plasticity Studied?
15.3 Plasticity: Adaptive and Developmental
15.3.1 Adaptive Plasticity
Neural Recovery and Neuroplasticity Process: Poststroke
15.3.2 Developmental Plasticity
15.4 Neurophysiology of Brain Injuries in Infants
15.4.1 Injuries to the Developing Motor System—Corticospinal Projections
15.4.2 Injuries to the Somatosensory System
15.5 Applying Concepts of Neuroplasticity to Adults and Children
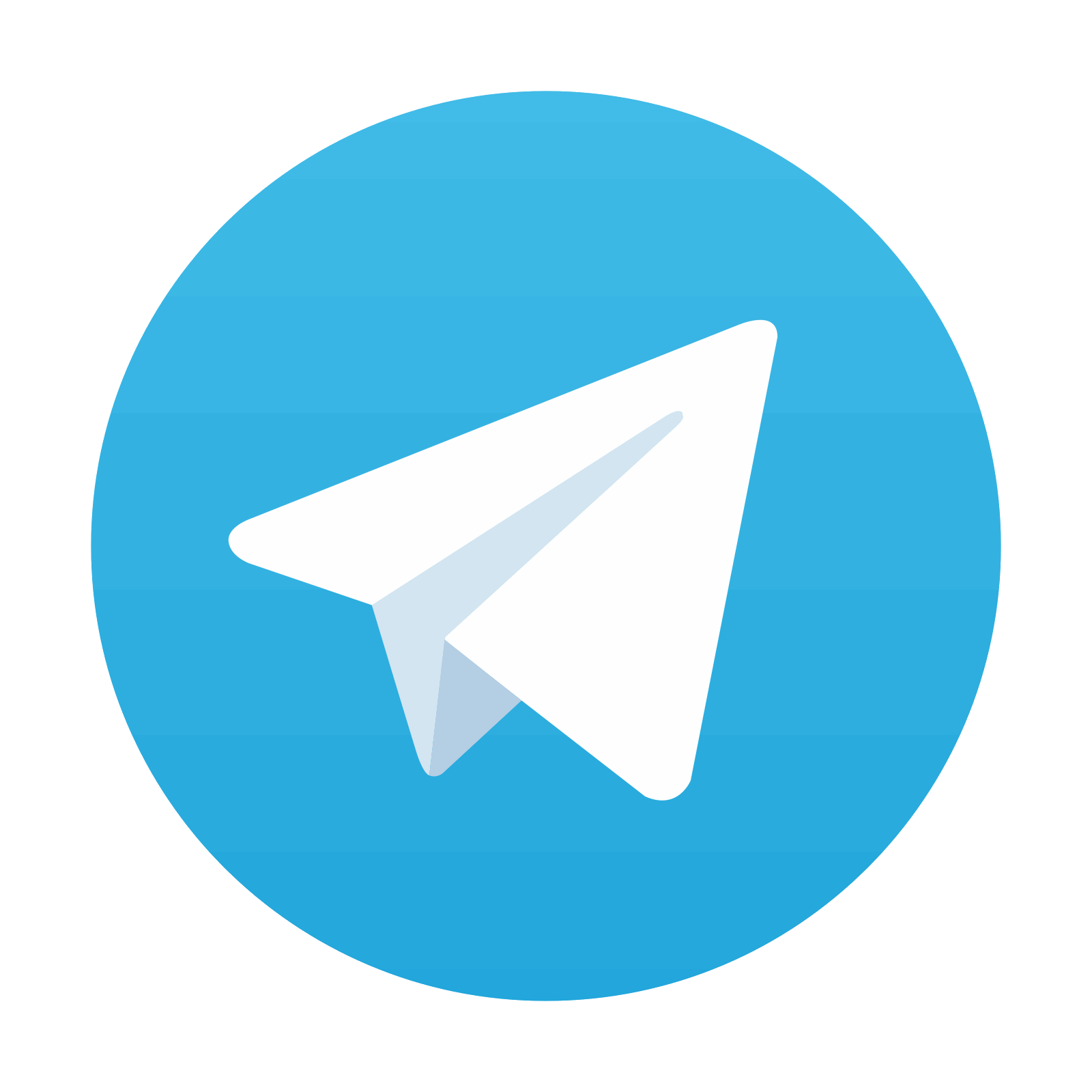
Stay updated, free articles. Join our Telegram channel

Full access? Get Clinical Tree
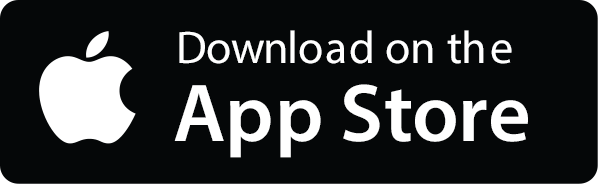
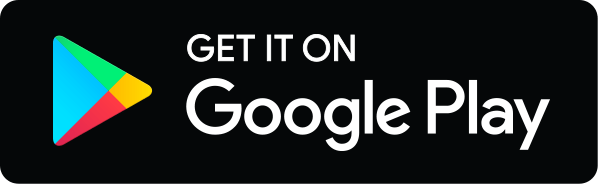