Pharmacol. type
Metabotropic
Ionotropica
Common denomination
Muscarinic
Nicotinic
Subtype
M2, M4
M1 (50–60% of the total mAChRs), M3, M5
Hetero-pentameric (α4 and β2 subunits)
Homo-pentameric receptor (only α7 subunits)b
Hetero-pentameric (α6 and β2 subunits)
Second messengers
Gi/o protein, inhibition of AC
Gq protein, metabolic cascades
Increased Na+ and K+ permeability
Increased Ca2+, Na+ and K+ permeability
Increased Na+ and K+ permeability
Localization
Presynaptic (M2/4). hippocampus and cerebral cortex, pedunculopontine and laterodorsal tegmental nuclei of the mesopontine tegmentum (M2), striatum (M4), co-localized with dopamine receptors
Predominantly extrasynaptic in forebrain, hippocampus, cerebral cortex, striatum, thalamus (M1). Hypothalamus and various other brain regions (M3). Pars compacta of the substantia nigra, ventral tegmental area (M5)
90% of the high-affinity nAChR in brain: localized in cortex, hippocampus, striatum, thalamus, superior colliculus and mesencephalon
Involved in classic excitatory neurotransmission in some brain regions where the release of neurotransmitters, neurite outgrowth and neuronal survival is also modulated
Mesostriatal pathway, substantia nigra, ventral tegmental area, nucleus accumbens, caudate–putamen, visual pathways
The metabotropic mAChRs are coupled to different types of G proteins, e.g., Gi/o type (M2 and M4 subtypes of mAChRs) of G proteins that negatively couple to adenylate cyclase or Gq proteins (M1, M3 and M5 subtypes of mAChRs), which convert the cholinergic signal into metabolic cascades [20]. Presynaptic mAChRs (M2, M4 subtypes) are largely inhibitory, and perform this function partly as inhibitory autoreceptors on cholinergic terminals [27]. The M2 subtype is the predominant autoreceptor in the hippocampus and cerebral cortex, whereas M4 is the main subtype in the striatum [15, 20, 21]. Postsynaptic mAChRs can be either inhibitory (M2, M4) or excitatory (M1, M3, M5) [15, 21].
The ionotropic, fast-signaling nAChRs are composed of five polypeptide subunits organized pseudo-symmetrically around a central pore [22]. Each subunit contains an extracellular domain, four hydrophobic transmembrane segments arranged in the form of three concentric rings around the pore, and a short extracellular carboxy-terminal domain. nAChRs are characteristically involved in the rapid “phasic” effects of ACh under conditions of brief release/high local concentration of the neurotransmitter, but they also operate under the low, tonic ACh release or mimicking systemically applied cholinergic drugs [16], a condition which may be particularly relevant to cholinergic neurotransmission — and/or its modulation — in the striatum.
Muscle-type nAChRs are expressed in the PNS and neuronal-type nAChRs in both PNS and the CNS as well as in other non-neural tissues such as immune cells, lymphocytes, lung epithelium, and other tissues [28]. In the CNS, the nAChR is present in various combinations of subunits (α4, α5, α6, α7, α9, α10, and β2 [29]), the two most abundant ones being the hetero-pentameric receptor formed by α4 and β2 subunits and the homo-pentameric receptor formed exclusively by α7 subunits. The deficit of some of the nAChR subunits in PD has been explicitly explored [30]. The two predominant forms of the nAChR, the α4β2 and the α7 oligomers, are also strongly expressed in the striatum, accompanied by the α6β2 form [31]. It is not clear whether other subunits are present in the heteromeric nAChRs. The α4β2 and α6β2 nAChRs in the striatum are localized at the dopaminergic terminal, the predominant target undergoing degeneration in PD. The α4β2 nAChR is also found in striatal GABAergic inhibitory interneurons [32].
The α7nAChR exhibits certain functional properties that distinguish it from other nicotinic receptors: (a) fast desensitization kinetics, (b) unusually high Ca2+ permeability, and (c) high affinity for binding α-bungarotoxin [29, 33]. In most regions of the brain, the α7 nAChR is found presynaptically, where it modulates enhanced neurotransmitter release of various other neurotransmitters, including DA, 5-HT, glutamate, and GABA; and postsynaptically, where it generates postsynaptic currents [23, 24]. In addition, the perisynaptic presence of the receptor has also been demonstrated, where it modulates neuronal activity, presumably by an unconventional mechanism involving diffusion of the natural neurotransmitter and binding to non-synaptic sites [16]. In the striatum, the α7 nAChRs are found in cortical glutamatergic excitatory afferents [23].
Cholinergic mechanisms are intimately linked to cognitive functions associated with cortical and hippocampal brain anatomical regions. Working memory, spatial and episodic memory acquisition, storage, maintenance and retrieval, attention, and other neurophysiological processes at the root of neural information and cognitive functions remit to ACh neurotransmission at synaptic, pathway, and circuital levels.
Neuropsychiatric Symptoms Resulting from Cholinergic Degeneration in PD
A summary of the brain cholinergic nuclei exhibiting signs of denervation in PD and their neuropsychiatric correlates is presented in Table 27.2. In this section, the contributions of cholinergic degeneration to the physiopathology of cognitive impairment, psychosis, and sleep disturbances in PD will be summarized.
Table 27.2
Sources of cholinergic dysfunction in PD and its main clinical correlates
PD feature | Pathological basis |
---|---|
Cognitive impairment | Degeneration of the NBM |
REM sleep behavior disorder | Degeneration of the PPN |
Psychosis | Reduced cholinergic tone (maybe PPN) |
Cognitive Impairment and Mood Disorders
The physiopathology of PD dementia (PDD) is complex and involves severe dopaminergic and cholinergic deficits, the main pathological drivers of cognitive decline being a synergistic effect between α-synuclein and AD pathology [34]. Only cholinergic deficits will be reviewed in this section.
Alteration in Cholinergic Receptors Expression or Function in Cognitive Impairment in PD
Because of their distribution in brain anatomical regions associated with cognitive processes, various subtypes of nAChR have been invoked as being associated with abnormal cognitive processes. The α7 nAChR is highly expressed in the hippocampus, a region particularly affected in cognitive disorders [29, 35–37], as recently reviewed in [38], whereas a massive loss in cerebral cortex of the other most abundant type of CNS nAChRs, the α4β2-type, accompanies the cognitive decline observed in AD [39, 40]. Not surprisingly, alterations in memory and cognition associated with nAChRs have also been reported in pathological states other than AD, such as schizophrenia [41]. The various functions afflicted in PD have been associated with nAChR dysfunction of different brain nAChR oligomeric forms [42–44]. α7 nAChR ligands are a subject of intense research in diseases affecting cognitive functions, especially the subclass of ligands termed positive allosteric modulators (PAMs, see reviews in [45]). This is a group of compounds that enhance recognition memory and cognitive improvement in animal models (e.g., [46, 47]).
Muscarinic receptors are also implicated in cognitive disturbances. Antagonists such as scopolamine perturb the performance of cognitive tasks in animal models [48] and even lead to extreme cognitive disturbances with delirium at higher doses [49]. This condition has also been reported in children after application of postsurgical transdermal patches to ameliorate nausea and motion sickness [50], and in the elderly, who are particularly vulnerable to even modest levels of antimuscarinic drugs due to their cumulative effects [51].
Several lines of evidence link brain nicotinic nAChRs, the α7 in particular, with the development of neurodegenerative disease with cognitive impairments, such as AD [45]. The greater the depletion of cholinergic neurons and associated cholinergic pathways in cognitive-associated brain areas such as the neocortex and hippocampus, the more severe the associated dementia, suggesting a relationship between the clinical manifestations and the level of cholinergic decline [52, 53]. Cholinergic pathways are associated with the processes of learning and memory, and nicotinic agonists and cholinomimetics in general have been used as therapeutic agents, providing symptomatic improvements in cognitive impairment [54–57]. This constitutes the basis of therapeutic approaches aiming at α7 AChR activation with selective agonists.
Data from Studies in PD Patients
The involvement of ACh pathways in PD is further exemplified by the results of a recent trial by Park and colleagues [58]. White matter hyperintensities in the cholinergic pathways were assessed by means of the Cholinergic Pathways Hyperintensities Scale (CHIPS) using 3.0 Tesla magnetic resonance. Patients with AD (n = 20), PDD (n = 21) and dementia with Lewy bodies (DLB, n = 17) were compared with a group of 20 healthy controls. Results showed that the CHIPS score was correlated with MMSE, SOB scores of the Clinical Dementia Rating, and verbal and visuospatial memory domains in demented patients.
Degeneration of the NBM appears to be highly correlated with PDD [59]. A recent study showed that PD patients with mild cognitive impairments (PD-MCI) who would develop PDD during follow-up had greater degeneration of the substantia innominata, where the NBM is located [60]. In this study, 51 PD-MCI were followed for a minimum of 2 years, during which PDD was diagnosed in 15 cases. Greater grey matter loss in the prefrontal area was also observed in subjects developing PDD. Loss of neurons in the substantia innominata was observed in early stages of the disease, and was further accentuated in PDD.
Recent results in post-mortem analyses of brains from demented and non-demented PD patients confirmed these results. In the study by Hall and colleagues, stereological analyses of the A9 and A10 dopaminergic neurons and Ch1, Ch2 and Ch4 cholinergic neurons located in the basal forebrain, along with an assessment of α-synuclein pathology in these regions and in the hippocampus, were performed in six demented and five non-demented PD patients and five age-matched control individuals with no signs of neurological disease [61]. Choline acetyltransferase (ChAT) activity in the hippocampus and frontal cortex was also measured in a different set of eight demented and eight non-demented PD patients, as well as in the same areas of eight age-matched controls. Stereological analyses showed a significant 54% reduction in the NMB of PDD compared to controls and a non-significant reduction of 30% in non-demented PD. No differences were observed in other cholinergic regions. Furthermore, the density of ACh neurons in the NBM correlated inversely with the severity of dementia. ChAT activity, a measure of the presence of cholinergic terminals in a given brain region, was reduced in the hippocampus of PD with dementia compared to non-demented patients and controls. Interestingly, neocortical ChAT activity was reduced in the neocortex of both demented and non-demented PD compared to controls. Finally, α-synuclein pathology and Lewy-body deposition in the basal forebrain of patients with PDD were more severe than in non-demented patients, thus suggesting the possible role of α-synuclein aggregation in the development of cortical and hippocampal cholinergic dysfunction.
The diminution in the density of the α4β2 nAChR in the CNS has been recently correlated with cognitive impairments in non-demented PD patients [62]. Previous studies had revealed reduced binding to these receptors in PD brains, and some preliminary findings suggest that the lower density of these receptors might correlate with cognitive impairments. In this study, 25 non-demented PD patients underwent a 5-[123I]iodo-3-[2(S)-2-azetidinylmethoxy] pyridine (5-I-A-85380) SPECT to visualize α4β2 nAChRs, and cognitive testing with the CERAD (Consortium to Establish a Registry for Alzheimer’s Disease) battery to identify domains of cognitive dysfunction [62]. Results showed significant correlations between performance of the CERAD subtests Boston Naming Test (a specific test for visual perception and for detection of word-finding difficulties) and Word List Intrusions (a specific test for learning capacity and memory for language information) with the density of α4β2 nAChRs at the right superior parietal lobe cortex and the left thalamus, and left and right posterior subcortical regions.
An interesting question is whether the alteration of the NBM is the same as that found in AD. NBM degeneration is comparable or even more intense in PD compared to the latter, yet the clinical characteristics of the two dementias differ significantly. Some authors have suggested that the divergence may be connected to possible differences in the degree to which subsections of the NBM are affected [63], but this hypothesis remains to be studied.
Depression can precede dementia, or at least depressed patients are at greater risk of developing PDD [64]. In a recent study, neocortical cholinergic innervation was assessed in 12 non-demented PD patients, six PDD and ten normal control patients [65] by means of dynamic PET scanning of previously injected [11C]methyl-4-piperidinyl propionate radioligand, a selective substrate for the enzyme AChE. Pooled analyses demonstrated a significant inverse correlation between cortical AChE activity and Cornell Scale for Depression in Dementia scores (r = 0.5, p = 0.007). The correlation remained significant when only PD patients were assessed, in whom AChE activity also correlated with the MMSE score. Recent evidence suggests that the early involvement of the posterior neocortex and visuoperceptual impairment may be risk factors for the rapid symptomatic progression and dementia in PD [66].
Cognitive Dysfunction as a Side-Effect of Cholinergic Drugs
In the light of the evidence reviewed above, it is not surprising that drugs interfering with cholinergic function have profound effects on cognitive function in PD. Muscarinic receptor blockers can cause acute confusion, dementia, and chronic intellectual impairment [67]. In a study with trihexyphenidyl, an oral anticholinergic agent, clinical disability, cognitive assessment, and measurements of cerebral blood flow (rCBF) and oxygen metabolic rate (rCMRO) were performed in six PD patients before and after administration of the drug for 7 weeks at 6 mg/day [68]. Results showed improvements in motor symptoms without evident changes in cognitive function. Cortical and striatal rCBF and rCMRO2 were significantly decreased, a typical finding in PDD [69].
Psychosis and Delirium
Visual hallucinations (VHs) are frequently reported by PD patients [2]. Besides the effect of dopaminergic medication, anticholinergics are associated with VHs even in patients without PD.
Data from Studies in PD Patients
In a recent study, inhibitory cholinergic activity in the CNS was measured by means of the short-latency afferent inhibition (SAI) technique in ten non-demented PD patients with VHs, in 12 non-demented PD patients without VHs, and in 11 age-matched healthy controls [70]. Results showed reduced SAI in patients with VHs, which was otherwise normal in patients without hallucinations. In addition, patients with VHs showed more frequent MCI and had reduced values in some cognitive function tests. The authors speculated that these results might be related to diminished neocortical cholinergic input from the NBM.
Delirium
Characterized by an acute and fluctuating disturbance in attention and awareness accompanied by an additional disturbance in cognition, delirium is more frequent in PD than in the general population [71]. Cholinergic deficiency is one of the most frequently found abnormalities in delirium. In a recent study, the association between exposure to anticholinergic drugs and delirium was studied in a database of more than 16,000 PD patients [72]. Results showed that 57.8% of PD patients were prescribed non-PD medications with moderate to very strong anticholinergic potential. Subjects exposed to anticholinergic polypharmacy had increased risk of delirium (adjusted OR: 1.61, 95% CI: 1.08–2.40).
Sleep Disturbances
Sleep disturbances are common disabling non-motor features of PD that have a detrimental effect on health-related quality of life [2]. Activation of the PPN is capable of inducing REM sleep [73] and degeneration of cholinergic neurons in the basal forebrain and brainstem is one of the factors resulting in a reduction in REM sleep and REM-sleep behavior disorder (RBD) [74]. RBD is characterized by a loss of normal muscle atonia during REM sleep and dream-enacting behavior. RBD occurs in 0.5% of the general population, but is considered to be a risk factor for synucleinopathies and even as a pre-motor sign of PD [75].
Data from Studies on Animal Models
The effects on REM sleep of drugs acting on different monoaminergic systems have been explored in the MPTP mouse model of PD [76]. The objective of the study was to assess the effects of these drugs on sleep/wakefulness patterns, measuring the amount of REM sleep (or paradoxical sleep -PS-). Arecoline, a muscarinic agonist, increased the amount of PS in the MPTP-treated mice but not in the controls, probably reflecting supersensitivity in the former.
Data from Studies in PD Patients
Cholinergic function has been recently evaluated in PD patients with or without RBD by means of short-latency afferent inhibition (SAI), a transcranial magnetic stimulation protocol able to test an inhibitory cholinergic circuit in the human brain [77]. In this study, ten PD patients with RBD diagnosis by polysomnography, 13 patients without the disorder, and ten healthy controls were enrolled. In addition to SAI, neuropsychological examination was also performed. SAI was reduced in PD patients with RBD compared to unaffected PD and healthy controls. Interestingly, MCI was more frequent in the former, and cognitive parameters correlated with SAI. These findings indicate that cholinergic dysfunction may play an important role in RBD in PD.
Symptomatic Treatment of Cholinergic Deficits in PD
The idea that enhancing cholinergic tone might be a first-line therapeutic strategy for cholinergic symptoms is logical and appealing. In this section, clinical use of cholinergic tone manipulation at the brain level by pharmacological or neurosurgical approaches will be explored. Other non-cholinergic treatments will be (briefly) mentioned for the sake of completeness.
Cognitive Impairment
A recent systematic review and meta-analysis suggested that inhibitors of cholinesterase are effective in the treatment of cognitive impairment in patients with PD [78]. The systematic search yielded three studies involving donepezil and one involving rivastigmine. The EXPRESS study included 541 patients with PDD who were randomly administered 12 mg rivastigmine or placebo and followed up for a mean of 24 weeks [79]. The study by Dubois et al. included 355 patients with PDD receiving 5 mg donepezil, 10 mg donepezil, or placebo, followed up for a mean duration of 24 weeks [80]. The study by Ravina et al. was a crossover study in which 22 patients with PDD were randomized to receive either 10 mg/day donepezil followed by placebo, or placebo followed by 10 mg/day donepezil for a mean follow-up of 10 weeks [81]. Results showed that these drugs significantly slowed MMSE decline (MD = −1.123, 95% CI = −1.638 to −0.608; p = 0.001; I2 = 44.6%), and ADAS-cog (SMD = −0.266, 95% CI −0.399 to −0.133; p < 0.0001; I2 = 0%). Interestingly, the death rate was lower in treated patients than in those receiving a placebo (OR = 0.295, 95% CI 0.108 to 0.806; p = 0.017; I2 = 0%). Tremor and adverse drug reactions in general were more frequent with cholinesterase inhibitors. A summary of these studies is presented in Table 27.3.
Table 27.3
Studies with cholinesterase inhibitors for cognitive impairment in PD
Author & Year | Drug/ Procedure | Design | Sample | Main results |
---|---|---|---|---|
Emre 2004 [79] | Rivastigmine | Randomized, double-blind, placebo-controlled trial | 541 demented PD patients | Improvement of 2.1 points in the ADAS-cog with rivastigmine vs 0.7-point worsening with placebo (p < 0.001) |
Ravina 2005 [81] | Donepezil | Randomized, double-blind, placebo-controlled trial | 22 demented PD | There was a 1.9 point trend toward better scores on the ADAS-cog on donepezil vs placebo (p = NS) |
Dubois 2012 [80] | Donepezil | Randomized, double-blind, placebo-controlled trial | 355 demented PD | In a post-hoc analysis, donepezil was better than placebo |
Mamikonyan 2015 [82] | Rivastigmine | Randomized, double-blind, placebo-controlled trial | 28 PD patients with minimal cognitive impairment | Non-significant benefits with rivastigmine |
Long-term safety of rivastigmine was studied in a 76-week, prospective, open-label, randomized study of 583 PD patients aged 50–85 years old [83]. Patients were randomly assigned rivastigmine 12 mg/d capsules or 9.5 mg/24 h patches. Primary outcomes included incidence of, and discontinuation due to, predefined adverse events (AEs) potentially arising from worsening of PD. Incidence of predefined AEs was 36.1% for capsules vs 31.9% for patch. Discontinuation due to worsening of motor symptoms was observed in 4.4% and 2.4% for capsule/patch respectively, and tremor in 24.5% vs 9.7%. Authors argued that these figures were in the range expected due to the natural progression of Parkinson’s disease. Rivastigmine is considered to be “Clinically Useful” for the treatment of dementia in PD according to the latest review of the Movement Disorder Society Evidence-Based Medicine Task Force [84]. Use of donepezil is considered “Investigational” [84].
The efficacy and safety of rivastigmine for the treatment of minimal cognitive impairment in PD (PD-MCI) have been explored in a recent study [82]. Patients with PD-MCI (n = 28) were enrolled in a 24-week, randomized, double-blind, placebo-controlled, crossover, single-site study of the rivastigmine transdermal patch. The primary outcome measure was the Alzheimer’s Disease Cooperative Study — Clinical Global Impression of Change (ADCS-CGIC). Twenty-six participants (92.9%) completed both study phase assessments, and 23 (82.1%) completed both phases on study medication. The CGIC response rate demonstrated a non-significant difference favoring rivastigmine.
In a recent study, patients with PDD or PD-MCI were compared to controls and studied before and after a 3-month therapy with rivastigmine patch [85]. At baseline, patients showed reduced spontaneous brain activity in regions important for motor control (e.g., caudate, supplementary motor area, precentral gyrus, thalamus), attention and executive functions (e.g., lateral prefrontal cortex), and episodic memory (e.g., precuneus, angular gyrus, hippocampus). Spontaneous brain activity deficits in the left premotor cortex, inferior frontal gyrus, and supplementary motor area were restored such that the activity was increased post-treatment compared with baseline and was no longer different from controls. These results are in line with a potential restoration of cortical cholinergic tone from the NBM induced by the drug.
Antimuscarinic drugs have a well-known deleterious effect on cognitive function [67], which has been confirmed in PD by a study measuring cerebral blood flow and oxygen metabolic rate after treatment with trihexyphenidyl [68]. These parameters are markers for dementia in PD [69]. Therefore, antimuscarinic drugs should be avoided in patients with cognitive impairments.
Memantine, a channel blocker of the N-methyl-d-aspartate (NMDA) type of glutamate receptors, has been prescribed for Alzheimer’s disease patients, and could possibly be used for the treatment of PDD who cannot tolerate cholinesterase inhibitors, but its efficacy has been poorly documented [86].
Psychosis, Delirium and Sleep Disturbances
The management of psychosis should start by withdrawal of potential offending drugs, anticholinergics and tricyclic antidepressant in particular [86]. Quetiapine in small doses might also be used if drug withdrawal fails [86]. Anecdotal evidence suggests interesting clinical effects with donepezil [87], and there is an ongoing randomized, double-blind, placebo-controlled trial with donepezil for this indication [88].
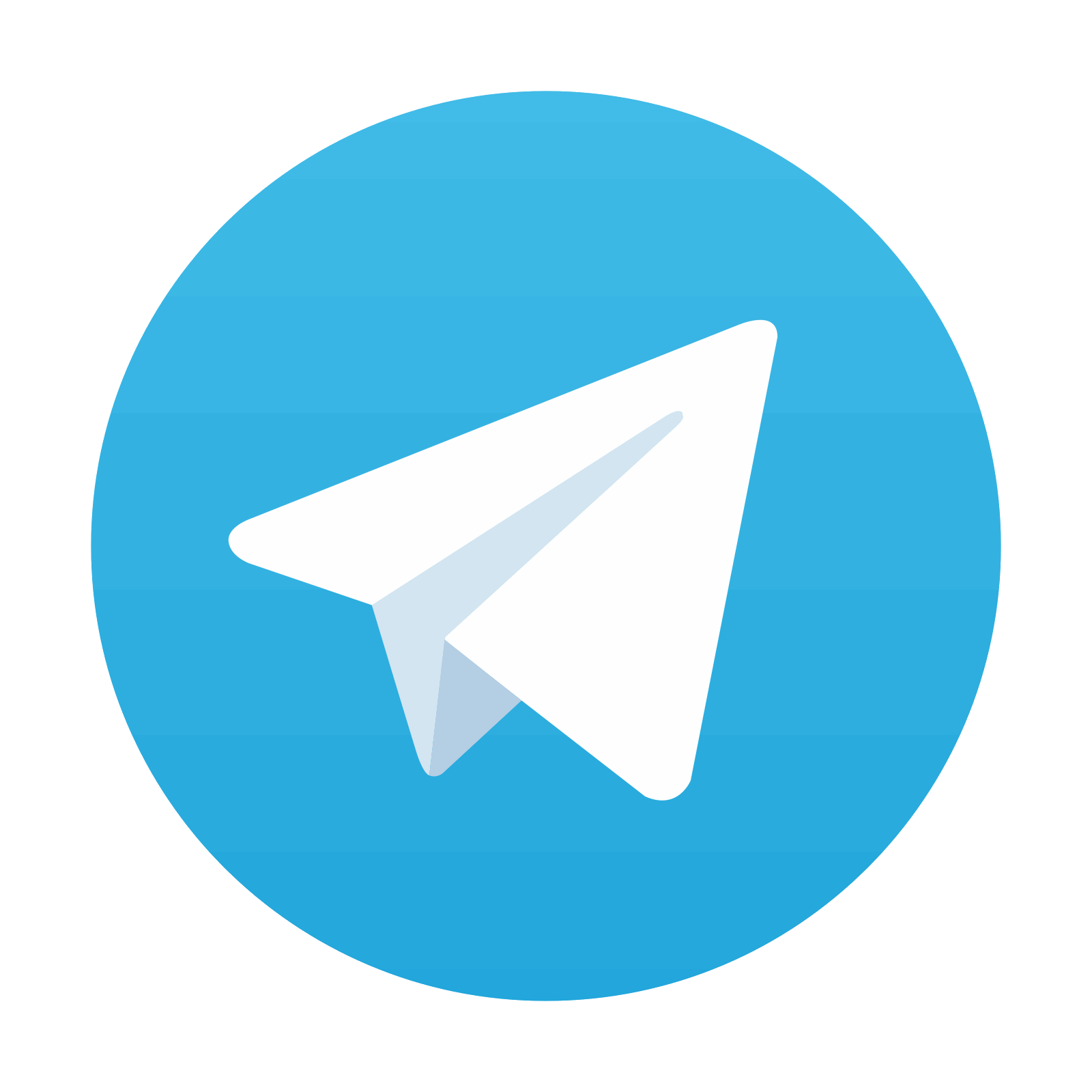
Stay updated, free articles. Join our Telegram channel

Full access? Get Clinical Tree
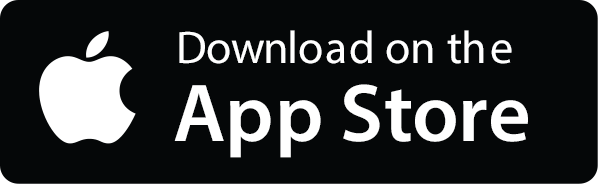
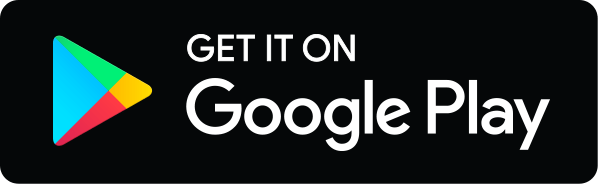