Odontoid Fractures
Richard J. Bransford
Joshua Charles Patt
Jens R. Chapman
INTRODUCTION: THE SCOPE OF THE PROBLEM
There is probably not another osseous structure within the human body that relative to its size elicits more controversy and uncertainty than the anterior rostral osseous protuberance of the axis. The lack of clarity extends to its preferred name, with a number of options such as dens axis, odontoid peg, and odontoid process having been used concurrently by authors over time and cultures. For purposes of this text, we will join the increasing number of authors who prefer the simple term “odontoid.” This bony prominence represents the focal landmark of the upper cervical spine as anchoring entity between the cranium and the cervical spine. Failure through injury makes it a focal point of concern due to its limited innate capacity toward healing in all but the most harmless of cases. This smallish bone, with its usual fully grown size approximating the two distal phalanges of an adult small finger, is subjected to considerable mechanical forces and a variety of age-related physiologic changes. Its construct affords the upper cervical spine its tremendous range of motion, while it supplies a modicum of stability to this region when healthy. Lack of appreciation to detail and an ignored or insufficiently treated odontoid injury can lead to poor or even devastating outcomes. Despite a relatively straightforward osseous architecture and an anatomic location, which allows for relatively safe manipulation and closed reduction, odontoid fracture management cannot be readily expressed as a simplistic algorithm derived from its two major nonoperative “conservative” options of collar or halo-vest immobilization with or without traction—or surgery with its two major options of anterior odontoid screw fixation or posterior atlantoaxial arthrodesis. This is due to a large number of variables both on the patient side and on the part of the treating physician (Fig. 47.1).
The contradictions and lack of agreement surrounding odontoid fracture treatment is not the result of disagreement over treatment goals. Universally, the goal of treatment is to return a patient with odontoid fracture to best possible preinjury function while exposing the patient to the least amount of complications and pain. Conversely, nontreatment of all but the most trivial odontoid fractures in functionally active patients can be expected to result in nonhealing of the fracture, secondary displacement, pain, dysfunction, and even serious neurologic injury or death. It appears that the majority of odontoid fracture patients in fact benefit from a decisive and insightfully applied surgical or nonoperative approach from relatively early on in the treatment process with little room for error in judgment or conclusion of the task. An improved awareness of treatment options in case of unsatisfactory care development is highly desirable. Unfortunately, none of the available treatments—and these include both surgical and nonsurgical care options—come without some sacrifice of function, and all have their inherent risks and drawbacks, which vary considerably relative to patient physiology and a number of other factors. This chapter seeks to provide its reader with a review of the many perspectives and confounding controversies surrounding odontoid injuries and updates on emerging insights.
EPIDEMIOLOGY
Odontoid fractures account for between 10% and 20% of all cervical spine fractures with a well-described bimodal age distribution of patients with a smaller peak occurring in younger, physically active, and healthy commonly male patients and a second increasingly larger peak of elderly and medically infirm patients with a larger number of female patients (1,2). Many of the fractures in the elderly are the result of low-energy mechanisms such as groundlevel falls, while odontoid fractures in younger adults occur during high-energy deceleration trauma such as motor vehicle collisions. A less common but nonetheless important injury cohort is seen in children under the age of 7 with disruption of the not-yet ossified synchondrosis of the dens.
The main inherent challenges associated with odontoid fractures emerge out of the surrounding circumstances of odontoid fractures: The younger odontoid injury population injured in high-velocity injuries are commonly polytraumatized, with concurrent head injuries, complex associated cervical spine trauma creating variable circumstances (3). The elderly population group is frequently adversely affected by comorbidities, such as dementia, ankylosing spinal disorders, osteopenia, and therapeutic anticoagulant use, to name but a few. Both sets of circumstances test clinicians in their own ways. For instance, both groups of patient share similar concerns of missed injuries
and delay in diagnosis. For multiply injured patients, this can result out of the turmoil of acute polytrauma care, while lack of initial focal patient complaints, dementia, osteopenia, and arthritic changes in elderly patients may explain delay in diagnosis in that age cohort. Fortunately, it appears that spinal cord injury—either of primary or of secondary nature—is relatively rare, perhaps a benefit of the relatively capacious spinal canal found between the foramen magnum and the C2-C3 disk space (4,5). While spinal cord injuries as a result of an odontoid injury are fortunately rare in this fracture cohort, they may be devastating and are probably underreported as an entity due to their associated circumstances—such as respiratory arrest at the time of injury. In patients who survived their injuries, the neurologic involvement reported is under 25% with a spectrum of injuries seen, such as Brown-Sequard Syndrome, mild upper extremity weakness, lower extremity hyperreflexia, as well as greater occipital neuralgia (5, 6 and 7).
With encouraging signs of decreasing numbers of odontoid fractures in the younger patient population perhaps reflecting benefits of greater vehicular safety measures, the clearly emerging clinical concern centers on the increasingly larger segment of elderly patient with odontoid fractures. These injuries occur in two subsets of the elderly: The one subset is highly active despite being affected by some age typical comorbidities. Injuries in this segment occur from car crashes, falls from heights during recreational activities, and work. Pharmaceutically induced anticoagulation, ankylosing spinal columns, pervasive cervical spondylosis, and obesity are some of the more commonly encountered comorbidities of these patients. The other patient cohort usually is injured in a highly consistent fashion—from a simple ground-level fall—yet poses a major threat to patient survival due to the circumstances leading up to the fall. Dementia, diminished neurocognitive skills, and major comorbidities can accumulate to substantial ethical and social challenges for all involved in the care process. These circumstances are not dissimilar to those encountered in other geriatric fractures such as in the hip, the proximal humerus, the distal radius, and others. Most of these “insufficiency fractures” are associated with falls from a standing height and can be linked to advanced pulmonary and cardiovascular disease, peripheral neuropathy, poor posture, weak muscle tone, joint contractures, insufficient proprioception, and generalized deconditioning in the setting of senile osteoporosis or -malacia. Because of the rapidly growing “geriatric” demographic population segment, improved sensible odontoid fracture care algorithms for the elderly are of significant importance from a societal and economic perspective (8).
and delay in diagnosis. For multiply injured patients, this can result out of the turmoil of acute polytrauma care, while lack of initial focal patient complaints, dementia, osteopenia, and arthritic changes in elderly patients may explain delay in diagnosis in that age cohort. Fortunately, it appears that spinal cord injury—either of primary or of secondary nature—is relatively rare, perhaps a benefit of the relatively capacious spinal canal found between the foramen magnum and the C2-C3 disk space (4,5). While spinal cord injuries as a result of an odontoid injury are fortunately rare in this fracture cohort, they may be devastating and are probably underreported as an entity due to their associated circumstances—such as respiratory arrest at the time of injury. In patients who survived their injuries, the neurologic involvement reported is under 25% with a spectrum of injuries seen, such as Brown-Sequard Syndrome, mild upper extremity weakness, lower extremity hyperreflexia, as well as greater occipital neuralgia (5, 6 and 7).
With encouraging signs of decreasing numbers of odontoid fractures in the younger patient population perhaps reflecting benefits of greater vehicular safety measures, the clearly emerging clinical concern centers on the increasingly larger segment of elderly patient with odontoid fractures. These injuries occur in two subsets of the elderly: The one subset is highly active despite being affected by some age typical comorbidities. Injuries in this segment occur from car crashes, falls from heights during recreational activities, and work. Pharmaceutically induced anticoagulation, ankylosing spinal columns, pervasive cervical spondylosis, and obesity are some of the more commonly encountered comorbidities of these patients. The other patient cohort usually is injured in a highly consistent fashion—from a simple ground-level fall—yet poses a major threat to patient survival due to the circumstances leading up to the fall. Dementia, diminished neurocognitive skills, and major comorbidities can accumulate to substantial ethical and social challenges for all involved in the care process. These circumstances are not dissimilar to those encountered in other geriatric fractures such as in the hip, the proximal humerus, the distal radius, and others. Most of these “insufficiency fractures” are associated with falls from a standing height and can be linked to advanced pulmonary and cardiovascular disease, peripheral neuropathy, poor posture, weak muscle tone, joint contractures, insufficient proprioception, and generalized deconditioning in the setting of senile osteoporosis or -malacia. Because of the rapidly growing “geriatric” demographic population segment, improved sensible odontoid fracture care algorithms for the elderly are of significant importance from a societal and economic perspective (8).
In the discussions of odontoid fractures the adult and geriatric perspectives frequently overshadow the pediatric domain. Cervical spine injuries, although rare in skeletally immature children, especially the very young, predominantly occur at the craniocervical junction (Oc-C2), with the majority of fractures being odontoid injuries. The disproportionately larger size and weight of the skull relative to the neck and trunk of a pediatric patient creates a mechanical disadvantage within the upper half of the cervical spine. Upper cervical spine injuries predominate in the pediatric population with the odontoid representing the majority of these injuries (9,10). The last synchondrosis of the axis to ossify is the subdental synchondrosis at the junction of the odontoid process and the body. This process is usually complete by 7 years of age (11). As a result of this developmental process, most pediatric dens fractures occur through this growth plate and are most frequently seen before the age of 7 and often associated with a hyperflexion mechanism (12).
Attempts at identifying general treatment algorithms for all types of odontoid fractures are bound to fail in light of the disparate epidemiology of patients affected and their divergent age-related associated medical burden. Current clinical research has increasingly focused on geriatric odontoid fractures due to starkly rising numbers of these injuries compared to declining fracture numbers in patients below 65 years of age and unresolved care issues in light of 1-year mortality rates regardless of treatment of over 40% (8). Several recent studies are focused on establishing a better understanding of the osteoporotic host and creating evidence-based guidelines for treatment.
ANATOMY AND BIOMECHANICS
The axis develops into its adult form from five primary ossification centers. The ring is formed by three such centers, one in the body and two in the posterior arch. There are also two centers in the dens itself with these two fusing around the time of birth. The last synchondrosis to fuse is the subdental synchondrosis at the junction of the body and the dens. This synchondrosis is usually fused by 7 years of age, but remnants of the growth plate can persist for 2 to 4 years radiographically (9,11).
The craniocervical junction allows for an impressive overall motion excursion and subsequently places considerable biomechanical demands on all aspects of its specialized osseoligamentous components to meet these demands. The atlas or C1 vertebra can be conceived as a transitional segment whose articulation with the occipital condyles provides primarily flexion and extension capabilities (13). The peg-like projection of the dens forms the central anchoring point for the upper cervical spine and articulates with the anterior arch of the C1 ring and provides translational restraint against horizontal displacement of the atlas via a series of ligamentous attachments from the dens to the skull base via the paired alar ligaments and the apical ligament. The odontoid process, or dens, is the most unique vertebrae due to its rostral projection from the body of C2 into the anterior third of the atlas. Anteriorly, the dens forms an articulation with the posterior recess within the center anterior arch of the atlas; posteriorly, it is constrained by the transverse atlantal ligament (TAL). In adulthood, the body of the axis flows into the dens and extends laterally into bilateral lateral masses. The rostral aspects of these lateral masses form slightly convex surfaces for articulation with the atlas. Their coronally rounded contour aids in allowing for rotation of C1 on C2. The rostral component of the lateral masses connects with short and narrow pedicles to the posterior facets, which in turn articulate with C3. The lamina of C2 is large and overhangs C3. The predictably large and bifid spinous process forms a major attachment for the nuchal ligaments and the short cranial rotator muscles. There are numerous norm variants of the excursion of the vertebral artery within and around the axis. This may predispose it to increased risk of injury from trauma and surgery and needs to be taken into account during injury assessment and preoperative planning (14).
Surrounding the dens is a true synovial joint with posterior confinement of the joint capsule provided in close proximity by the TAL and the tectorial membrane (13). The ligaments of the craniocervical junction form an integrated functional unit that centers around the odontoid. The key intrinsic anchoring ligaments of the craniocervical junction can be differentiated into three layers and are situated anterior to the spinal cord. The odontoid ligaments are the most anterior layer and include the single central apical and the paired wing-shaped alar ligaments. The apical ligament is a small fibrous band extending between the dens tip and the basion and can be considered to be
rudimentary in humans. Alar ligaments are horizontally aligned ligaments, which connect either lateral surface of the dens tip to their corollary occipital condyles. Dvorak et al. (13) identified two components of the alar ligaments, a superior and an inferior one, each with their respective biomechanical role.
rudimentary in humans. Alar ligaments are horizontally aligned ligaments, which connect either lateral surface of the dens tip to their corollary occipital condyles. Dvorak et al. (13) identified two components of the alar ligaments, a superior and an inferior one, each with their respective biomechanical role.
The middle layer of the internal craniocervical ligaments is represented by the cruciate ligament. The TAL is the main component of this structure and is a horizontally aligned well-developed condensation of fibers between a bony protuberance of the anteromedial aspect of the medial aspect of the atlantal lateral masses. There are small triangular fibrous bands that extend from the transverse ligament rostrally to foramen magnum and caudally to C2. Posterior to the cruciate ligament lies the tectorial membrane as a thin but broad ligamentous continuation of the posterior longitudinal ligament on its path to its origination in the anterior rim of foramen magnum.
This complex interplay of bony and soft tissue restraints provides stability while facilitating rotation via the relatively flat joint surfaces of the C1-C2 facet joints. Approximately 45 degrees of motion to either side is attainable, representing almost half of the rotation of the entire cervical spine (13). The articulating surfaces are sloped upward from lateral to medial allowing for a degree of helical motion between C1 and C2 with rotation, permitting greater motion before the soft tissue restraints of the alar ligaments tighten. Another unique attribute of the C1-C2 articulation is the relatively large spinal canal that this segment encompasses. In a normal setting, this allows for significant motion without undue pressure on the spinal cord, which, in the injured state, helps explain the relatively low incidence of significant neurologic injuries, even with significant fracture displacement (4).
Although not in immediate proximity of the odontoid process, treatment of odontoid fractures requires knowledge of the integrity and location of the vertebral arteries. These arteries are the only arterial vessels within the human body thatjoin together rather than divide and take four 90-degree turns in the small spatial confinements of the upper cervical spine, likely in support of craniocervical motion. This vascular flow redundancy also preserves continued cerebral blood supply during extremes of head turning with temporary occlusion of one artery. It appears that with progressive age, usually the left vertebral artery becomes more dominant (15,16). Within the transverse foramen of the axis, the vertebral artery may progressively erode the medial bony support of the lateral mass, thus increasingly hollowing out the inferior lateral mass below its articular surface. This can significantly impact the selection of surgical technique, as certain types of screw fixation into the axis may put the vertebral artery at risk (17). In its more cranial portion, the vertebral artery then commences lateral to the atlantoaxial joints and continues rostrally through the more laterally located foramen transversarium of the atlas. Along the superior surface of the atlas, the arteries turn medial and cross on top of the atlas within a groove. At the lateral edge of the posterior occipitoatlantal membrane, the arteries perforate the dura and arachnoid and pass into foramen magnum. Then, they course ventrally on the medulla oblongata and at the level of the pons join together to form the basilar artery.
The normal process of aging plays a role in the prevalence of geriatric cervical spine fractures seen and specifically with fractures of the odontoid. There appear to be two separate osteologic processes that contribute to the fracture predilection of this bony prominence. The first is generalized arthrosis or spondylosis of the spine. Decreased range of motion through the facet joints with increasing spondylosis is an expected naturally occurring age-related change affecting the spine. The relative severity of ankylosis is probably influenced at least in part by genetics. A relative increase in stiffness of the subaxial spine increases biomechanical stresses upon the upper motion segments. With advanced cervical spondylosis, there is also an increased prevalence of arthrosis seen between the tip of the dens and the clivus as well as its articulation with the C1 ring in presence of generalized cervical spondylosis. For reasons incompletely understood, this generalized arthrosis, however, doesn’t entail the atlantoaxial joints. In the setting of such well-preserved atlantoaxial joints, this places an increased rotational moment arm on the base of the odontoid during deceleration trauma (18).
The second process that contributes to the prevalence of odontoid fractures in the elderly is a relative decrease in bone density, especially in the waist of the odontoid and around the body of the axis. The microarchitecture of the axis shows a decreased volume of cancellous bone, poorer trabecular interconnections, and decreased cortical thickness in the lower regions of the dens at its connection with the base with advancing age (19). This baseline susceptibility is compounded exponentially by the relative osteopenia associated with the aging process. Similar to the process seen in the femoral neck and thoracic vertebrae, the trabecular changes associated with diminishing bone density place the geriatric patient at increased risk of fracture, even from relatively trivial low-velocity mechanisms, such as ground-level falls or striking one’s head against a fixed object (Fig. 47.2).
ASSESSMENT
HISTORY AND EXAMINATION
The circumstances surrounding the occurrence of odontoid fractures can make physical examination less than straightforward. In patients with high-impact injury, the serious nature of associated injuries can make the clinical diagnosis difficult. In the elderly patient group, dementia and senility can make communication about pain difficult. In cognitively unimpaired patients, upper cervical spine fractures are usually accompanied by complaints of neck pain, headache, and nuchal tenderness. There may be some swelling and ecchymosis over the fracture site in the back of the neck, but this is not a common finding on physical exam. Palpation may reveal crepitus, but there is a paucity of objective physical examination findings. A full neurologic assessment is obtained, and the subaxial, thoracic, and lumbar spine should be evaluated for any local tenderness or step deformity to rule out any noncontiguous spine fractures. Dysphagia, with associated increased risk of aspiration, may be present from an expected retropharyngeal hematoma and may be more commonly
encountered in patients with advanced age (20). Should a patient be neurocognitively intact and if medically not contrain dicated, a simple swallowing test such as performed in an upright position with a small amount of water ingested from ice chips may help determine presence of traumatically related dysphagia—an important component in the decision-making process for definitive care.
encountered in patients with advanced age (20). Should a patient be neurocognitively intact and if medically not contrain dicated, a simple swallowing test such as performed in an upright position with a small amount of water ingested from ice chips may help determine presence of traumatically related dysphagia—an important component in the decision-making process for definitive care.
Neurologic assessment is preferably performed according to American Spinal Injury Association guidelines (21). In the rare cases of primary spinal cord injury associated with odontoid fractures, asymmetric patterns of upper and/or lower extremity weakness and numbness may be present. Cranial nerve function should be part of any examination of patients with possible head or neck injuries. The abducens and hypoglossal nerves are most commonly affected by craniocervical injuries (22). A wide variety of neurologic injury patterns are possible in patients with trauma to the upper cervical spine. These range from complete pentaplegia with loss of respiratory function to incomplete injuries, such as cervicomedullary syndromes and disorders affecting brainstem function. The cervicomedullary syndromes, which include cruciate paralysis, as described by Bell, and hemiplegia cruciata initially described by Wallenberg, represent the more unusual forms of incomplete spinal cord injury and are a result of the specific anatomy of the spinal tracts at the junction of the brainstem and spinal cord (22). Cruciate paralysis can be similar to a central cord syndrome, although it normally affects proximal more than distal upper extremity function. Hemiplegia cruciata is associated with ipsilateral arm and contralateral leg weakness. Due to the rostral nature of a potentially accompanying spinal cord injury, breathing, swallowing, and gag reflex may be impaired to a variable degree (22). In extreme cases, complete quadriplegia may result from an odontoid fracture. Due to the expected respiratory arrest of an injury at such a level, survival is rare and strongly correlated to timing of airway restoration.
In a geriatric patient with decreased general functional status, the primary focus of assessment is targeted on identifying the odontoid injury in the first place and then looking for typical associated injuries, such as hip and wrist fractures. In geriatric patients with diminished functional baseline status, it is quite common to receive nothing more than vague and nonspecific complaints of neck ache and some occipital pain. It is very helpful in the treatment decision-making process to elicit reliable and meaningful reports of the patient’s preoperative functional status from spouses, family, or care providers and identify associated clinical comorbidities that may affect the management plan (23).
IMAGING
Plain Radiography
Historically, odontoid fractures may have been one of the most commonly missed spinal fractures. Various imaging modalities and protocols have evolved over time to optimize the identification, workup, and management of odontoid fractures (24). The use of plain radiographs in an Emergency Room setting has become increasingly rare in most North American hospitals (25, 26 and 27). As stated above, the classic plain radiographs consisting of a crosstable lateral radiograph, two oblique views, an anteroposterior (AP) open-mouth odontoid view, an AP x-ray, and a swimmer’s view have largely been supplanted by computed tomography (CT) scans that are obtained using a risk-based algorithm (28). This, however, does not mean that plain radiographs are obsolete. Lateral cervical spine radiographs allow for injury detection in over 90% of cervical spine trauma during primary assessment; with an open-mouth odontoid image added to the lateral and traditional AP image, this number can be raised to about 95% of cases (25). The value of plain radiographs should, however, not be minimized as this modality remains the prevalent form of follow-up imaging during postoperative care for assessment of spinal alignment and hardware placement. Especially the addition of dynamic voluntary flexion-extension radiographs to assess for stability in the postprimary phase remains an option for determination of structural stability in select patients. Even in its static form, lateral cervical-spine radiographs can provide helpful insights into cervical spine stability. For instance, side-byside comparison of lateral recumbent and upright lateral radiographs of patients allows for important insights into intrinsic stability of the cervical spine regardless of presence and type of collar, halo vest, and any type of surgery. Therefore, plain radiographs, especially using the lateral cervical spine projection, remain useful as a screening tool and play a more supplemental role in assessing alignment and overall cervical spine stability. They remain the primary imaging modality in follow-up and after healing to ensure with flexion-extension films that acceptable healing has occurred.
Computed Tomography Scan
CT scans of the head have become a priority for all patients with suspected or evident head injuries after initial stabilization (26,29). The addition of a full cervical spine CT screen using a helical scanning protocol adds minimal imaging time to a head CT, yet provides the clinician with a full set of cervical spine images from the occiput to T4 including reformatted views of the upper and lower cervical spine. In most centers, a CT of the cervical spine is currently done as the primary modality to assess for cervical spine injury due to the inherent limitation of the plain radiographs in evaluating the upper cervical spine and cervicothoracic junction (25,26,28). The use of CT imaging has been confirmed in its ability to obtain rapid, efficient cervical spine evaluation and negate the need for plain radiographic imaging at the cost of increased radiation exposure to the patient (26).
Conventional 3- or 5-mm axial cut thickness CT images may not depict axial plane fractures such as commonly seen in odontoid fractures. This circumstance can be mitigated by obtaining sagittal and coronal reformats of the CT scan. An important additional consideration in favor of using CT scans as primary imaging modality for spine trauma lies in the 34% risk of noncontiguous spine fractures that have been reported to be associated with odontoid fractures (29). Three-dimensional image reformations, obtained from fine-cut CT scans, are rarely clinically useful but may assist with the interpretation of more
unusual upper cervical injury patterns and can be of benefit in the management of atlantoaxial region malunions.
unusual upper cervical injury patterns and can be of benefit in the management of atlantoaxial region malunions.
Another use of CT scans in odontoid fracture management is the ability to date fractures to some degree. Differentiation into broad categories of acute (<3 weeks), subacute (<3 to 6 months), and old (>6 months) is usually possible using indirect signs such as prevertebral soft tissue swelling, definition of fracture ends, and presence of ossification responses around the injury zone. This may influence the choice of treatment.
Rapid acquisition CT scanning also allows for angiographic representation of the vertebral and carotid arteries. Flow obstruction of the vertebral arteries may be suspected with highly displaced craniocervical injuries and in presence of fractures involving the transverse and vertebral foramina.
Magnetic Resonance Imaging
Magnetic resonance imaging (MRI) is usually not a primary imaging tool for patients with odontoid fractures. Specific indications for MRI for patients with odontoid pathology may include presence of neurologic injury; ligament disruption; occult fracture; and, of course, workup of neoplasia, inflammatory disease, and infection. In the presence of trauma absent of neurologic deficit or prior radiologic abnormality, MRI may not be indicated (30). T2 weighted fat suppression images can demonstrate hemorrhage or edema within the spinal cord, disruption of ligaments normally responsible for upper cervical stability, as well as subarachnoid and prevertebral hemorrhage (see Fig. 47.2). MRI test is useful to evaluate the severity of nerve compression or spinal cord injury but is less accurate at detecting a fracture than a CT scan.
Radionuclide Scanning (Tc-99 Scan with Single Photon Emission CT)
Radionuclide imaging, usually on form of a Technetium 99 Bone Scan (Tc-99 scan), has a distinct role in identifying occult injuries in a postprimary phase. Persistent neck pain despite unimpressive CT scans and stable plain radiography, even on flexion-extension imaging, may lead a clinician to request this modality. This is especially relevant in pediatric cases, where injuries of the growth plate through the subdental synchondrosis may lead to persistent doubts of presence of an injury.
CLASSIFICATION
In reflection of the overarching impact of the odontoid on the craniocervical junction, the odontoid and its surrounding structures deserve comprehensive assessment. Ultimately, the key treatment question related to odontoid fractures is the determination of primary stability of the injury zone. Unfortunately, there is no clear guidance on this subject—there are many bony and ligamentous injury subtypes to consider, and adjuvant factors, such as patient and bone health, vascular and neurologic injury status, expected patient compliance, and presence of concurrent injuries elsewhere all play major roles in our interpretation of “stability.” Stability relative to the spine generally infers its ability to withstand physiologic loads without the potential for neurologic defects, progressive deformity, or longterm dysfunction from pain and disability. Unfortunately, this concept lacks specificity, thus making structured formal assessment of patients and judgment using best available evidence important factors in the decision-making process for treatment.
Sadly, odontoid fracture classifications provide only a modicum of injury severity and stability rating. The first classification of odontoid fractures was introduced by Schatzker et al. (31) in 1971, who initially separated dens fractures into “high” and “low” depending on where the fractures were in relation to the accessory ligaments. The most widely used classification of odontoid fractures used to date remains that of Anderson and D’Alonzo in 1974, who differentiated three subtypes according to fracture level (Fig. 47.3) (6).
Type I—Type I fractures are rare and occur near the tip of the odontoid process, above the transverse ligament. This is the least common type of odontoid fracture and likely a result of an alar ligament avulsion. They are considered to be stable injuries if they occur in isolation and without concurrent craniocervical ligament disruption. However, this fracture type also may be associated with unstable occipital-cervical dislocation that can result from bilateral avulsion of the alar ligaments or a contralateral occipital condyle fracture. Recognition of a type I injury is therefore most relevant for its “indicator function” of presence of a serious craniocervical disruptive injury (22). A type I acute fracture may also be mistaken for an os odontoideum or vice versa.
Type II—Type II fractures are the most common injuries of the dens. The fracture involves the junction of the body of dens in its thinnest waist part as it joins the body of the axis. While type II fractures are the most common odontoid injuries, occurring in 38% to 46% of cases in the general population, this number rises to 82% to 95% in elderly patients (32,33). Due to its location with limited cancellous bone structure, high biomechanical stressors, and age-related limited bony vascularity, fractures in the waist zone of the type II region are typically fraught with much higher nonunion rates than type II injuries (6,7,29).
Type III—Type III fractures are usually oblique or comminuted and involve the body of C2 in addition to passing through the waist of dens. In distinction to type II fractures, type III fractures extend below the level of the bony recess along the medial edge of the superior articular plafond. Most type III fractures are considered inherently stable and are associated with a relatively high union rate due to their preeminent fracture location involving cancellous bone.
The Anderson and D’Alonzo classification has its limitations. Only fair intrarater and interrater reliability has been reported with plain radiographs and CT imaging. The kappa coefficient for classifications based on plain radiographs was 0.30 and 0.25 (fair agreement) at the first and second readings, respectively. For classifications based on CT scans, the corresponding kappa coefficients were 0.46 (moderate agreement) and 0.67 (substantial agreement). The kappa coefficients for intrarater reliability averaged 0.56 (moderate agreement) when CT scans were used and
0.28 (fair agreement) when plain radiographs were used. The preeminent limitation of the Anderson and D’Alonzo system arises from the difficulty in separating types II and III injuries in a meaningful and therapeutically relevant fashion (34).
0.28 (fair agreement) when plain radiographs were used. The preeminent limitation of the Anderson and D’Alonzo system arises from the difficulty in separating types II and III injuries in a meaningful and therapeutically relevant fashion (34).
Given the wide array of fracture patterns and subtle differences, various modifications of the classic Anderson and D’Alonzo classification have been introduced. In 1988, Hadley described a subtype of the Anderson-D’Alonzo classification typified by significant comminution of the base of the odontoid and referred to this as a type IIA fracture (35). Apfelbaum further distinguished type II fractures in a study looking at odontoid screws according to the AP direction of the fracture line as anterior oblique (16%), posterior oblique (34%), or horizontal (50%) in patients after anterior screw fixation (36). This concept was further clarified by Grauer et al. (37) who proposed a “modified treatment-oriented classification.” They first narrowed the definition of type II fractures to injuries that do not involve the superior articular facets of the axis. By this definition, a type II odontoid fracture could extend into the body of C2 but would be biomechanically similar to the originally described type II injury in the Anderson-D’Alonzo concept. Secondly, Grauer also created three other subtypes based on treatment suggestions and anticipated outcomes of various previously “lumped” type II injuries. Type IIA
fractures have a transverse pattern and demonstrate less than 1 mm of displacement and have no comminution. Type IIB fractures have an oblique orientation with a fracture line extending from anterosuperior to posteroinferior. Type IIC injuries also have an oblique orientation but extend from anteroinferior to posterosuperior. These injury variants are not infrequently associated with significant anterior comminution. Thus, in using current classifications, a type IIA fractures as defined by Hadley is profoundly different from a type IIA fracture as defined by Grauer.
fractures have a transverse pattern and demonstrate less than 1 mm of displacement and have no comminution. Type IIB fractures have an oblique orientation with a fracture line extending from anterosuperior to posteroinferior. Type IIC injuries also have an oblique orientation but extend from anteroinferior to posterosuperior. These injury variants are not infrequently associated with significant anterior comminution. Thus, in using current classifications, a type IIA fractures as defined by Hadley is profoundly different from a type IIA fracture as defined by Grauer.
In close association with typing and subtyping of odontoid fractures, fracture variables such as angulation, distraction, translation, and comminution may heavily influence management and may aid in predicting healing prognosis. Unfortunately, none of the current classification systems take these factors into account other than the type IIA fractures as described by Hadley. In a recent review of the literature, a panel of experts found no optimal or consistent methods for quantifying the actual fracture variables parameters (14). Carlson et al. (38) suggested measuring displacement by drawing lines along the anterior aspect of the dens fragment and the intact caudal body of C2. The angle subtended by these lines would be the degree of fracture angulation. The location of the apex of fracture angulation would be described as anterior or posterior. To measure fracture translation, a tangent line is drawn along the anterior aspect of the odontoid fragment and the anterior aspect of the C2 body. At the level of the fracture, a transverse line is drawn connecting these two lines. This distance is measured in millimeters and represents sagittal fracture displacement. To measure fracture angulation, a tangent line is drawn along the posterior aspect of the odontoid fragment and the posterior aspect of the C2 body. The angle subtended by these lines would be the degree of fracture angulation. The location of the angulation of the fracture apex could be used for the description of anterior or posterior displacement (Fig. 47.4; Table 47.1) (14).
NONOPERATIVE CARE AND OUTCOMES
A review of the literature and its reported results of odontoid fracture care is likely to lead to confusion and bewilderment due to the widely differing and even outright contradictory results. It helps to remember that the vast majority of the literature to date consists of case reports with anecdotal basis of management forming the foundation of our current treatment beliefs. Using GRADE criteria, a systematic review of the literature rated the vast majority of the literature on odontoid fractures to be of “very low” and occasionally “low” quality, with literally no exceptions (39). The current foundation of the literature, however, does allow for a number of important insights as a number of relatively consistent observations are starting to emerge.
The options for care largely revolve around some form of collar immobilization or closed reduction followed by halo-vest immobilization. Over time, a large number of factors associated with odontoid nonunions have been identified. Clearly, any form of collar immobilization, regardless of the design or rigidity of its design, is largely a supportive treatment measure, intended to prevent secondary displacement of all but the most stable osseous injuries, and well proven as a postoperative aid or perhaps a consideration for more palliative care of very ailing geriatric patients (Fig. 47.5).
Displaced injuries usually require some form of closed reduction, which is usually applied through cranial traction with tongs or a halo ring. For isolated odontoid fractures, usually a very low amount of weight will suffice in disengaging the fracture—adjustment of alignment and daily follow-up radiographs to avoid overdistraction are far more relevant concerns.
In considering chances for healing of an odontoid fracture, it helps to remember the geometry of bone surfaces in form of actual contact at the fracture site in addition to stability in considering chances for healing. Ryan and Taylor (40) measured the contact between fracture fragments at different displacements. A malreduction of not more than 20% translation in any plane was calculated to result in 40% loss of contact area. Healing of type II odontoid fractures treated without rigid external immobilization or when missed has a near 100% chance of non-union (7,41).
In theory, a majority of patients with type II fractures could be considered for treatment with halo-vest immobilization as the most rigid form of external immobilization. However, nonunion with the use of the halo-vest treatment has also been reported to occur in 15% to 85% of cases (41, 42, 43 and 44). The lack of success of odontoid fracture healing should be added to the listing of complications incurred by the treatment itself. Generally accepted complications can be differentiated into pin-related issues and torsorelated problems. Pin tract complications include infections (involving superficial soft tissues or bone), unsightly scarring, aspiration, and penetration of the cranium with a wide variety of sequelae, some of which can be serious in nature (7,23,45). Torso-related issues largely revolve around pulmonary problems, aspiration, and pressure ulcers. It appears that there are some patients in whom halo-vest application is clearly not advisable or feasible. This includes patients with multiple injuries, major craniofacial injuries, significant spinal deformity or ankylosing spondylitis, and truncal obesity. Elderly patients with diminished health and mental status may not be suitable candidates for the intricacies of halo-vest care, which includes compliance with aspiration prevention and pulmonary exercises. Recommendations for duration of halo-vest care usually state a duration of 2 to 4 months with a series of check radiographs and daily pin and vest care. In the end, successful fracture union of an odontoid fracture does not imply return of normal motion in the atlantoaxial motion segment. In fact, axial rotational motion remains permanently reduced by at least 30%, likely a by-product of arthrofibrosis, heterotopic ossification, and the preponderant adverse influence of even subtle degrees of malunion on the final functional recovery of this region (Fig. 47.6) (46).
Finally, the actual stability of odontoid fracture reduction remains variable. The lack of definitive immobilization of the cervical spine has been clearly identified by Anderson et al. and Lind et al. who demonstrated the “snaking” phenomenon—an undulation of the lower cervical spine relative to the upper cervical spine, when comparing recumbent lateral to upright lateral radiographs (18). These images, when obtained early on, together with simple swallowing assessments, and other clinical observations, may offer helpful insights into the likelihood
of obtaining healing of the fracture with halo-vest care (44,49, 50 and 51).
of obtaining healing of the fracture with halo-vest care (44,49, 50 and 51).
More recent studies have shown that with attempts at patient selection and maintenance of a meaningful halo protocol, 76% of patients can achieve successful fracture healing with halo-vest immobilization. Pin-related complications occurred in 13% of patients with an overall incidence of 35% of patients incurring some form of complication. An important insight of this study revealed that a majority of patients whose nonoperative care attempts failed did so within the first 3 weeks. Thus, determination of the likely success of nonoperative care with halo/vest immobilization can likely be made within the first 3 weeks, and conversion to most forms of surgery can be readily made during that time frame (Fig. 47.7 and 47.7) (45).
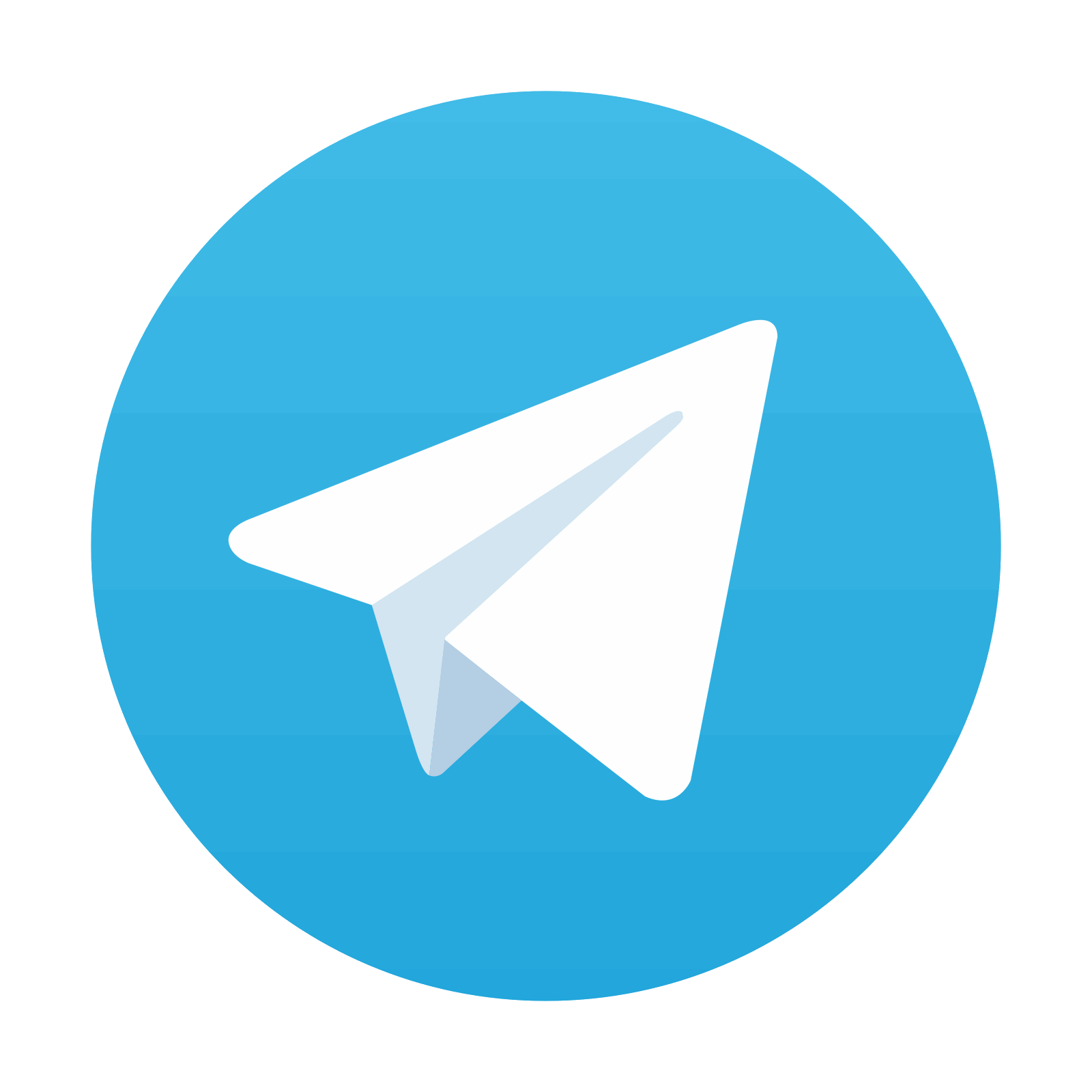
Stay updated, free articles. Join our Telegram channel

Full access? Get Clinical Tree
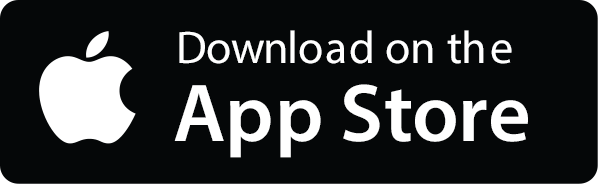
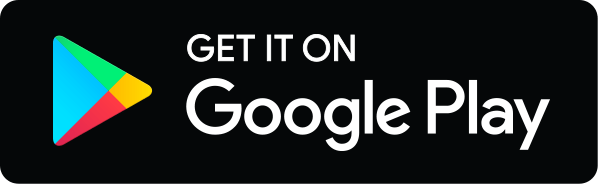
