CHAPTER 234 Operative Neurophysiology of Peripheral Nerves
Historically, a surgeon confronted with a peripheral nerve lesion in continuity faced an enigma. Should the lesion be resected and repaired, or should an attempt at neurolysis and removal of scar tissue be made? For some lesions, the answer to the question was often intuitive and based on the physical appearance of the lesion and the nerve itself. Unfortunately, this appearance shows poor correlation with the functional integrity of the nerve and represents weak grounds for a decision.1 If the surgeon chose to resect the lesion and repair a nerve injury that was undergoing spontaneous regeneration, that regeneration would be interrupted and the patient’s recovery would be delayed and diminished.1,2 If the surgeon were compelled to have more information to make a decision and waited, the delay would compromise recovery. In the absence of spontaneous regeneration, when a long window of time can elapse from the time of injury, the efficacy of regeneration after repair is greatly reduced.3 The majority of these alternatives lead to a poor outcome. Preoperative clinical electromyographic studies in the laboratory only served to determine continuity of axons with muscle and could give just limited information concerning regeneration.4,5 The surgeon was thus compelled to abide by the intuitive decision and accept the resulting outcome as the best possible effort.
More recently, the advent of operative neurophysiology has provided a new source of information that facilitates making this decision during surgery. With the use of stimulation and recording to neurophysiologically evaluate a section of injured nerve, the presence of adequate numbers of functional axons can be determined directly.6,7 The method is benign and easy to use and provides specific information to the surgeon that is not available in any other way. It permits the surgeon to develop a strategy for accurately diagnosing and treating a specific lesion in continuity. This strategy involves a delay in surgery of 2 to 6 months. During this time, any components of the lesion that are neurapraxic should resolve and return of function would be evident. Function that remains absent could then be attributed to axonotmetic or neurotmetic lesions.1,2 During this window, axonotmetic and mild neurotmetic lesions (Sunderland grade 3) would begin the process of spontaneous regeneration, and the growing axons could be revealed at surgery by stimulation and recording methods. However, more severe neurotmetic lesions, Sunderland grade 4, do not exhibit significant spontaneous regeneration because regrowth is impeded by scar. The absence of a significant compound nerve action potential (CNAP) 2 to 6 months after injury indicates a Sunderland grade 4 injury.1,8 With this information in hand, the surgeon should be comfortable with the decision to resect the lesion in continuity and repair the resection. This gives the patient the best chance for some functional return.9,10
When the interval between nerve injury and surgical exploration is long (3 to 5 years), there is opportunity for ineffective fiber regeneration to occur. In this case, a minimal number of very small motor units may be present, although there may be no voluntary function.2,11 These small motor units are not capable of mediating voluntary function. However, when these few motor units are artificially synchronized by a stimulus pulse that activates all of them simultaneously, some visible contraction of distal muscles may be observed, but there may be no CNAP recorded from the distal nerve under these conditions. Such contraction is deceptive because it does not provide any indication of a regenerative process that will lead to significant functional recovery. Therefore, the use of visible contractions of associated muscles as a means of detecting nerve conductivity is not recommended with long-standing injuries.1,2,12
Electrical Characteristics of Nerves
A large named nerve such as the median or ulnar nerve in the forearm may contain many thousands of individual axons. Within this nerve would be populations of different types of axons of varying caliber and myelination. These different types of axons all have their specific, individual electrical characteristics. Although these individual characteristics will be discussed, the combined activity of all these axons together is called the CNAP. Each individual axon demonstrates an “all-or-none” action potential, whereas many axons firing nearly simultaneously produce the CNAP, which varies continuously up to a maximum amplitude. The amplitude of the CNAP is continuously graded by size and is directly proportional to the number of axons that fire together.13 Many of the properties of the CNAP will closely resemble the properties of the individual axons that are most numerous in the nerve. Thus, the CNAP is a reflection of the general histology of a particular nerve.14 A section of nerve that contains abundant large-diameter, heavily myelinated axons will produce a CNAP that is large in size, is relatively narrow in width, and demonstrates rapid conduction velocity. When graphically displayed, this CNAP would have a narrow base and appear tall and thin because the majority of axons in this nerve would have very similar characteristics. By contrast, a different nerve that might contain fewer, smaller, lightly myelinated and many unmyelinated axons would produce a smaller CNAP and conduct at a lower velocity because there would be greater diversity in the electrical characteristics of the individual axons in this nerve. Graphically, this CNAP would have a broader base and would appear short and stubby. The important principle is that the CNAP is produced by a population of axons and its appearance gives some indication of the internal composition of axons in the nerve.14
As an action potential propagates down a single axon, only a small section of the axon will undergo the active processes—changes in sodium and potassium permeability—at any given time. For a small population of axons arranged side by side, such as a small peripheral nerve, the active processes occur in similar adjacent axons at almost the same time when the whole nerve is electrically stimulated.13 Considering the whole nerve, we could identify a small segment of the nerve that would contain active axons, referred to as the active length of the nerve. With larger nerves, the number of axons is much greater, and consequently the diversity within the population is greater. Therefore, the active length of a large nerve is greater than the active length of a small nerve. In an effort to record the difference in potential that is the CNAP, we must provide contact with the nerve at the active length of the nerve, as well as contact with the nerve at a point that does not contain active axons. Thus, placing two electrode contacts along the length of a peripheral nerve for the purpose of recording the CNAP requires that the spacing between these electrode contacts be greater than the active length for that nerve. With small nerves we can space our electrode contacts close together, 2 to 3 mm along the length of the nerve, but for larger nerves they must be moved farther apart, 5 to 7 mm, to obtain optimal recordings. Similar distances would also be ideal for the stimulating electrodes. Under these conditions the amplitude of the CNAP from a normal nerve would range between 100 and 400 µV or greater. The small size of this CNAP might tempt one to use averaging to enhance the response. However, the averaging process cannot be recommended because it could lead to a deceptively large response that would falsely indicate large numbers of normal fibers.1
The distance between the stimulating and recording electrodes also has limitations. Stimulus artifact is a common problem encountered in operative recordings. When the distance between the stimulating and recording electrodes is less than approximately 2 cm, the amount of stimulus artifact becomes so great that it can obscure a small CNAP. This limitation becomes an important consideration in children, in whom long lengths of nerve simply may not be available.15 In these instances it may be advisable to place stimulating or recording electrodes outside the surgical field. Although both subdermal needle electrodes and surface electrodes can be used, it is best to use these as stimulators and make recordings from within the operative site. In this way, sensitivity is maximal and the findings are most accurate. Similar considerations apply when preoperative magnetic resonance neurography has made it possible to identify the precise location of an injury—targeted minimal-access exposure based on this information may not be compatible with the need to access an adequate length of nerve for intraoperative recording.
When sufficient length is available within the operative site to accommodate both stimulating and recording electrodes, there may still be problems with stimulus artifact. Use of a tripolar stimulating electrode may significantly reduce this stimulus artifact and permit recordings that would not otherwise be possible. This solution has been considered elsewhere and adequate reference is provided.7,16 Another source of excessive stimulus artifact is the wires connected to the electrodes. When both the stimulating and recording wires exit the surgical field together and in close proximity, artifact is induced in the recording wires from the stimulating wires. It is therefore recommended that the wires connecting electrode pairs to the instrumentation exit the surgical field separately to eliminate this source of interference.
The duration of the stimulus pulse can also be adjusted to reduce stimulus artifact. When relatively long-duration stimulus pulses are used, on the order of 0.2 msec, stimulus artifact is considerable. Reducing stimulus duration to a value between 0.02 and 0.05 msec provides considerably less stimulus artifact. Under these conditions, however, it may be necessary to raise the intensity of the stimulus to ensure that it is adequate.13,17 With stimulus pulse durations less than 0.5 msec, the rheobase portion of the strength-duration relationship is encountered. At durations of 0.02 to 0.05 msec there is little hazard in providing stimulus pulses between 40 and 60 V (15 to 25 mA) with the electrodes in direct contact with the nerve. Such high intensity may be necessary to demonstrate nerve activity in some portions of heavily scarred, injured nerve. When one or more fascicles of a scarred, injured nerve conduct, function can be demonstrated by stimulating and recording from these few fasicles.18
An additional advantage of using short-duration stimulus pulses is that such stimulation preferentially activates larger-diameter, more heavily myelinated fibers.13,17 Small-diameter unmyelinated axons are not as sensitive to short-duration pulses. If the purpose of recordings is to demonstrate the important functional components of a peripheral nerve, the larger fibers that mediate significant motor function should be considered. The short-duration stimulus pulses enhance the dichotomous distinction between small-diameter unmyelinated fibers that may not have any functional correlate and the larger diameter myelinated fibers that will be associated with motor units capable of producing good motor function.
In the case of very proximal injuries, there may not be sufficient length of nerve to apply both stimulating and recording electrodes.19 To demonstrate conductance over a very proximal portion of nerve, somatosensory evoked potentials may be very useful. Stimulation at a level distal to the point of injury may produce a response in the sensory cortex that clearly demonstrates connection. However, it should be pointed out that the presence of only a small number of functional sensory fibers can lead to a normal somatosensory evoked potential. A significant nerve lesion may still be present even though the somatosensory evoked potential is normal.
Recently, transcranial motor evoked potentials have been used to discriminate motor nerve function from sensory nerve fibers.20 This technique involves stimulation of the motor cortex transcranially and recording directly from nerves. It may also be useful in dealing with very proximal lesions such as avulsive injuries.
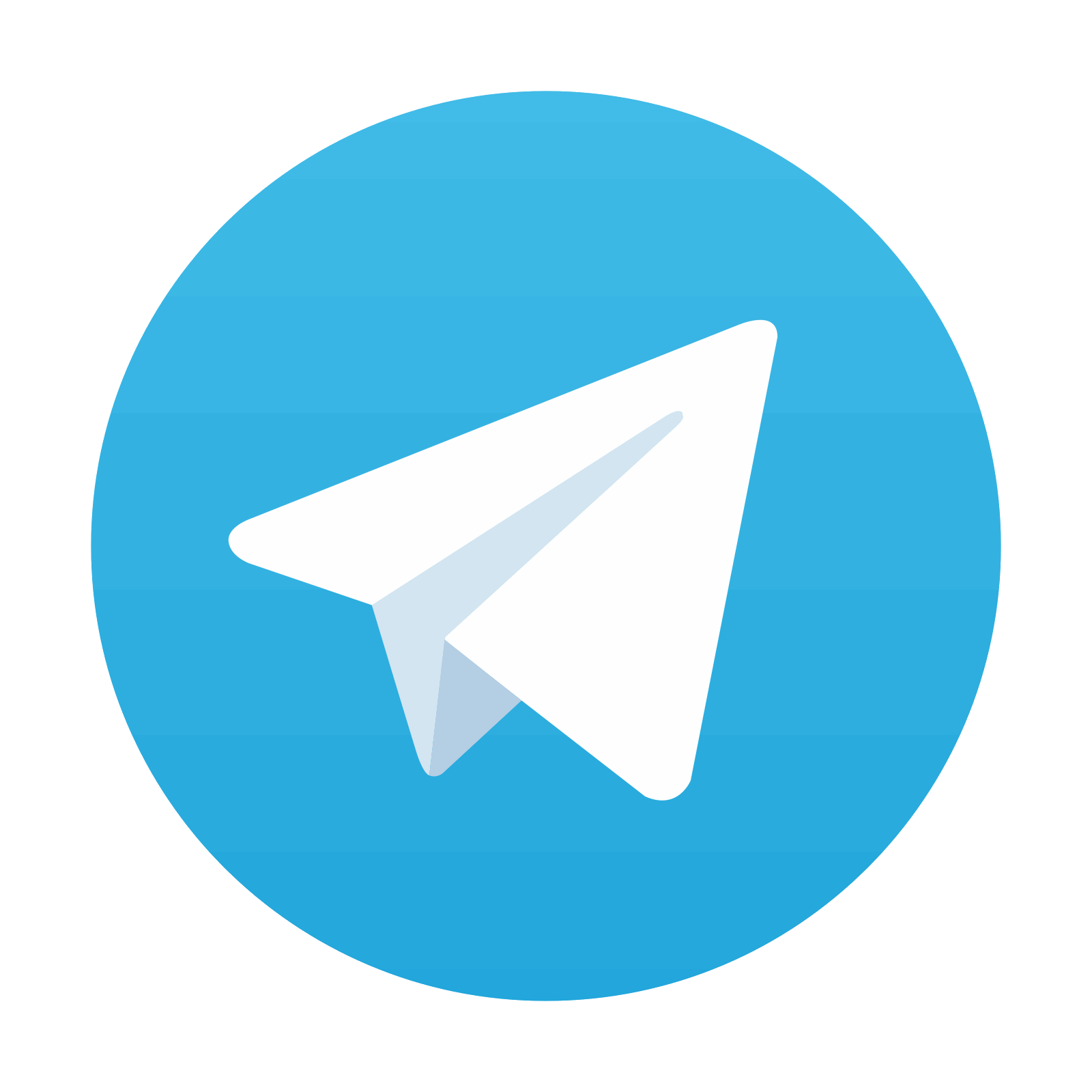
Stay updated, free articles. Join our Telegram channel

Full access? Get Clinical Tree
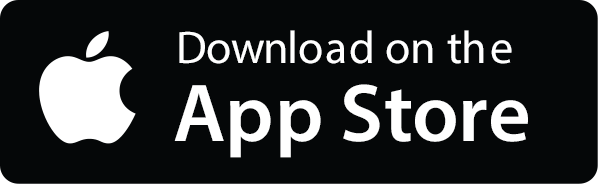
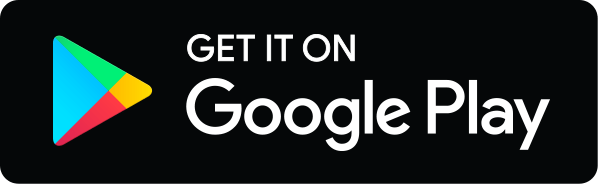