CHAPTER 393 Genetics of Cerebral Cavernous Malformations
Cerebral cavernous malformations (CCMs) are some of the most common types of vascular malformations of the central nervous system (CNS), and they affect nearly 1 in 200 people. CCM lesions are characterized by grossly dilated vascular channels lined by a single layer of endothelium (Fig. 393-1).1 They typically affect the brain and spinal cord but may also involve the skin and retinal vasculature.2 CCMs lack normal vessel wall elements such as smooth muscle and are also devoid of intervening normal parenchyma.3
The CCM population prevalence of 0.5% is an estimate based on both magnetic resonance imaging (MRI) and autopsy studies. Although fairly common in the general population, only 20% to 30% of CCMs become symptomatic.4–7 Patients are typically seen initially in the third through fifth decades of life because of hemorrhagic stroke, headache, seizures, or focal neurological deficits.5 As a result of their rudimentary vessel wall and the low-pressure blood flow through the lesions, CCMs tend to “leak” and cause microhemorrhages as evidenced by a characteristic hemosiderin ring seen on MRI (Fig. 393-2).3 CCM lesions can also lead, albeit rarely, to gross macrohemorrhages causing clinical signs and symptoms reflecting the size and location of the bleeding. Catastrophic hemorrhages are much rarer, again being mainly dependent on the location. Treatment options for CCMs include observation for asymptomatic lesions, antiepileptic medication for seizures, and surgical excision of accessible lesions in patients who suffer from symptomatic hemorrhage or intractable seizures.8,9
Both sporadic and familial forms of CCM exist. The familial form is usually manifested as multiple lesions in the setting of a strong family history of neurological disease. Sporadic forms almost never involve more than two lesions, and a family history is absent. The heritable nature of CCMs has been well recognized since its original description10; however, the limited neuroradiologic data available to early investigators resulted in an incomplete appreciation for affected family members. The advent of MRI has led to the detection of asymptomatic CCMs, and in 1988, Rigamonti and coauthors reported a series of 13 Hispanic American families with CCM, complete with clinical and radiographic features,11 a study complemented in 1998 by the first large series of white, non-Hispanic patients with familial CCM.12 These and other studies have demonstrated that although only 10% to 20% of affected white individuals have a first-degree relative with the disorder, this figure is as high as 50% in Hispanic Americans, a population in which our group has demonstrated a strong founder mutation.10,13
It is now established that the genetic mode of inheritance of familial CCM is autosomal dominant with a high degree of penetrance, thus rendering it an ideal genetic model for human hemorrhagic stroke. Genetic linkage analyses have mapped three CCM loci to chromosomes 7q (CCM1), 7p (CCM2), and 3q (CCM3).14–19 All three causative genes have now been identified, which has allowed new insight into the pathophysiology of CCMs.
Molecular Genetic Approaches
In parametric linkage analysis, a single extended family pedigree, large enough to support linkage independently, is analyzed. A number of DNA markers, in the form of single nucleotide polymorphisms (SNPs) or short tandem repeats, of known location dispersed throughout the entire human genome are subsequently used to investigate the inheritance of polymorphic regions of the genome by statistically computing recombination frequencies. For each marker, evidence of linkage is sought by using cosegregation of the trait (and presumably the disease-causing gene) and a particular variant of the marker being analyzed. This is possible because the closer the disease and marker loci are located on a chromosome, the less likely it is that a recombination event between them will occur and the more likely that the specific marker variant will be present in all individuals who are affected with the disease. Thus, the genetic distance between the marker locus and the disease-causing gene can be estimated in the genetic unit of morgan (M), with one-one hundredth morgan (1 cM) corresponding to a recombination fraction of 1%.20
Statistically, the logarithm of the odds (LOD) score function is used to test for significance of the linkage21 and is a robust measure of its strength. The LOD score represents the logarithm of the odds that the DNA marker locus is linked to the disease trait and is calculated according to the following formula:
The availability of new molecular genetic technologies, including high-density SNP arrays, allowed the development of new methodology, such as CNV analysis. Recent studies using the CNV approach have allowed identification of segments of DNA in the genome ranging from kilobases to megabases in length that are lost (deleted) or gained (duplicated).22 Although CNVs are abundantly present and distributed throughout the human genome, thus suggesting that many benign variations exist,23 it is becoming evident that an increased burden of CNVs is observed in disease states.24–28 We and others have successfully applied this technology to identify rare disease-causing alleles in CCM.29,30
Genetics of Cerebral Cavernous Malformations
Familial CCM is an autosomal dominant disease with high, but incomplete penetrance. It should also be kept in mind, however, that mutations in one of the three identified CCM loci are evident in only 96% of familial CCM cases.12 Thus, the possibility of a fourth, to date unidentified CCM locus should not be excluded. The disease that ensues from mutations in the three loci is clinically and radiographically very similar.
The three CCM genes thus far identified are Krev1 interaction trapped protein-1 (KRIT1) located on chromosome 7q as the CCM1 gene, MGC4607 (or malcavernin) on chromosome 7p as the CCM2 gene, and programmed cell death-10 (PDCD10) on chromosome 3q as the CCM3 gene.31–38 The following sections focus on the molecular biology of these three genes.
CCM1
The CCM1 gene is located on chromosome 7q21 and contains 16 exons that encode a 736–amino acid protein, KRIT1. This is the first CCM gene that was identified and, consequently, has been the most studied in terms of its cellular function. Mutational analysis of this gene has revealed a founder mutation (C742T) that is responsible for more than 90% of familial cases in Hispanic American patients.13,32,33 Numerous studies have now identified in excess of 80 different mutations of this gene leading to clinically evident CCM. Nearly all are nonsense mutations in the form of stop codons or frameshift mutations that result in premature termination of translation.33,35,39–43 Thus, there is strong evidence to suggest that loss of function of the CCM1 protein leads to disease, which probably results from a two-hit mechanism.44 In the two-hit model, the first genetic “hit” is inherited, whereas the second is acquired in a subset of cells and rapidly leads to disease afterward because affected cells can no longer synthesize functionally intact proteins.
Krit1 was initially identified in a yeast two-hybrid screen as an interactor of the small Ras family guanosine triphosphatase Krev1/Rap1a.45 Even though later studies challenged this finding,46,47 more recent studies have confirmed this interaction, thus suggesting that Rap1a signaling might be important in CCM pathophysiology.48,49
The KRIT1/CCM1 protein contains a number of domains implicated in protein-protein interactions, including a FERM domain at the C-terminal, which is typically critical for binding to the cell membrane.50 Recent studies have suggested this domain’s importance in CCM1’s binding cell-cell junctional proteins (see later).48,49 KRIT1 also contains a series of ankyrin repeats, but the proteins that interact with CCM1 through this domain remain unknown.45 Finally, CCM1 contains three NPXY domains that are known to interact with phosphotyrosine-binding (PTB) regions. Two of these domains create a binding site for integrin cytoplasmic domain–associated protein-1α (ICAP-1α)46,47 and CCM2 (see later) (Fig. 393-3).51
Our group was the first to demonstrate that CCM1 interacts with the plus end of microtubules.52 This finding was later confirmed by Beraud-Dufour and colleagues, who also demonstrated that CCM1 can adopt both an open and a closed conformation, depending on the interaction of the N-terminal NPXY motif with its C-terminal.48 In vitro, CCM1 interacts with microtubules in its closed conformation, which would in turn help deliver CCM1 to the plasma membrane, where it would bind to ICAP-1α in its open conformation. Even though both the small G-protein Rap1a and ICAP-1α can disrupt CCM1’s association with microtubules, thereby localizing it to endothelial cell-cell junctions, only ICAP-1α appears to be able to unfold CCM1.48
ICAP-1α is known to bind to β1 integrin, again through an NPXY/PTB domain interaction.53,54 Integrins, specifically β1 integrin, are important proteins that regulate endothelial cell adhesion and migration throughout angiogenesis.55,56 These observations imply a role for CCM1 in angiogenesis with its involvement in the Rap1a/Krev1 and integrin pathways. More recent studies suggested that after localizing to the cell membrane, CCM1 can also bind to β-catenin and play a role in stabilizing adherens cell junctions and regulating endothelial cell permeability.49
In addition, CCM1 binds to both CCM2 and CCM3.51,57 The exact nature of the interaction between the three CCM molecules is currently unknown, but among these three proteins, CCM2 appears to act as a scaffolding protein58 and is potentially recruited to cell-cell junctions through its interactions with CCM1.
Even though the molecules involved in the downstream signaling of CCM molecules are unknown, in vitro studies previously suggested that CCM1 translocates to the nucleus upon cellular stimulation,51 and recent work in Caenorhabditis elegans confirmed this finding by showing interaction of the CCM1 ortholog kri-1 with a transcription factor daf16.59 Mutations in kri-1 result in increased life span, mediated though its interactions with daf16. The importance of this aspect of CCM signaling in humans is currently unknown, but dissecting this molecular pathway will have important implications in understanding disease pathophysiology.
In an attempt to gain some insight into CCM pathophysiology, our group focused on the temporal and spatial expression patterns of the three CCM molecules. Our initial studies demonstrated CCM1 to be expressed in many human tissues, including the heart, brain, kidney, liver, pancreas, and spleen.60 Furthermore, CCM1 is expressed specifically in the endothelium of capillaries and arterioles, but not in the venous system.61 CCM1 (but also CCM2 and CCM3; see later) is abundantly expressed in neurons and astrocytic foot processes (Fig. 393-4), an observation potentially related to the finding that CCM lesions almost exclusively form within the CNS.61
In vivo studies based on mouse models have revealed an essential role for Ccm1 in vascular development: complete loss of Ccm1 results in embryonic lethality at midgestation.62 Ccm1−/− embryos demonstrate vascular defects incompatible with life, namely, dilated precursor brain vessels, an enlarged caudal dorsal aorta (probably caused by increased endothelial proliferation), and variable obstruction of branchial arch arteries and the proximal dorsal aorta.62 All these findings suggest a primary defect in arterial morphogenesis. Ccm1+/− animals are phenotypically normal with no apparent vascular phenotype and do not form CCM lesions. Interestingly, when these Ccm1+/− animals are crossed with p53−/− mice, vascular lesions resembling cavernous malformations in the brain develop in approximately 55% of the compound Ccm1+/−;p53−/− mutants.63 The mechanism underlying the enhanced phenotype in the absence of p53 is not known but is speculated to be an increased rate of somatic mutations leading to more second hits and thereby resulting in the formation of CCM lesions in the compound animals.
When viewed as a whole, these studies are beginning to shed light on the cellular function of the CCM1 protein and its involvement in angiogenesis. CCM1 probably exerts the majority of its effects at the level of the cerebrovascular unit, where it mediates communication between its components, neural cells, and vascular endothelium of the CNS (Fig. 393-5).
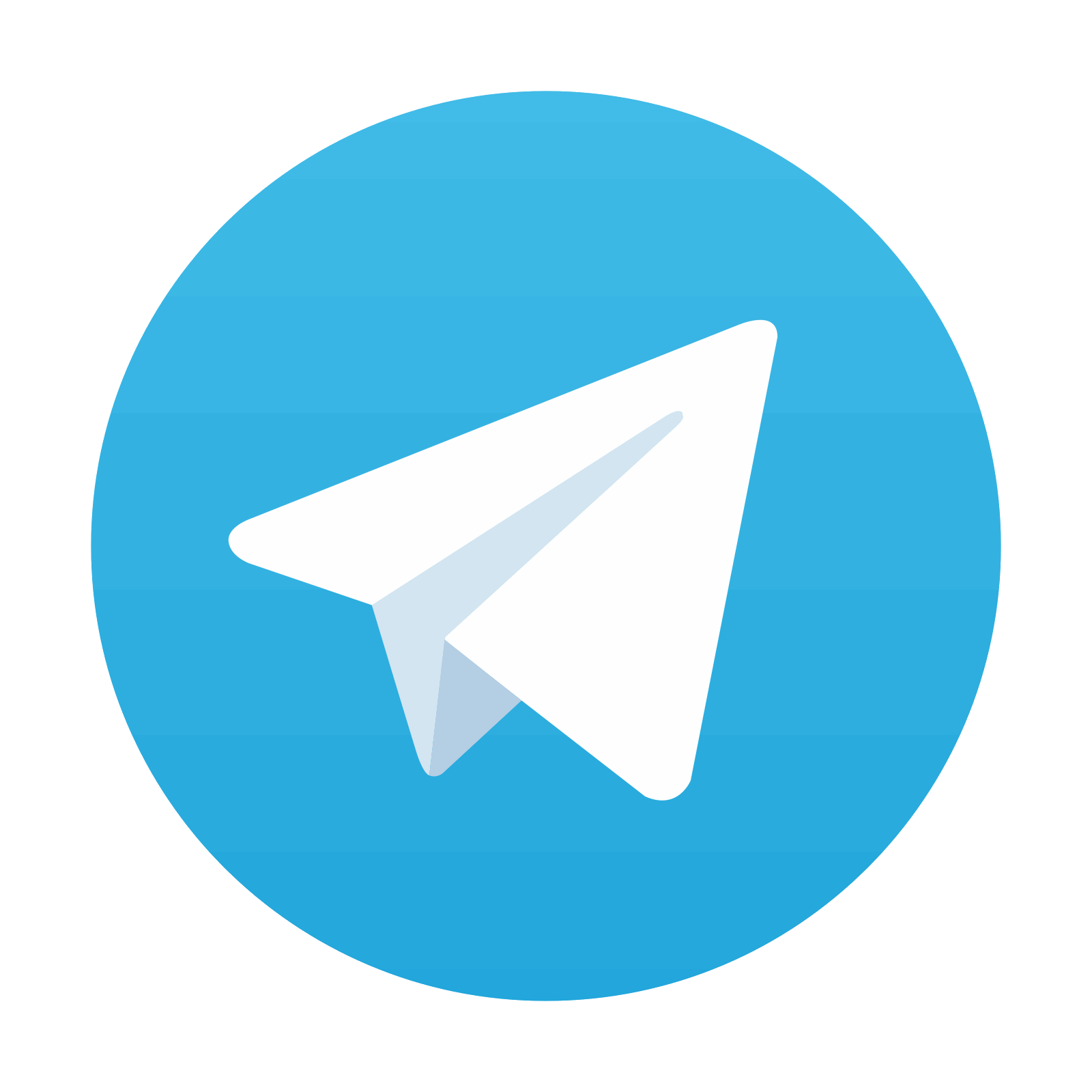
Stay updated, free articles. Join our Telegram channel

Full access? Get Clinical Tree
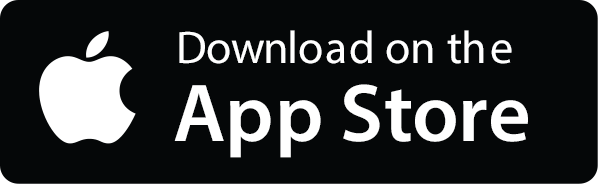
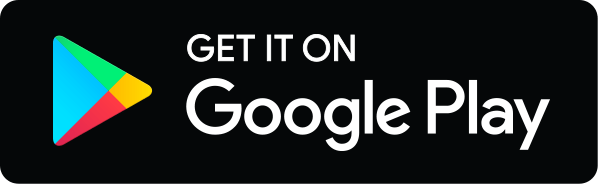