5 Ophthalmologic Evaluation and Management Visual signs and symptoms are extremely frequent manifestations of disease affecting the sellar and parasellar region. Medical treatment, radiation therapy, radiosurgery, and surgical intervention in and around the parasellar region may affect afferent or efferent visual function, producing problems with decreased vision, visual field defects, and double vision. Ophthalmic management of patients with skull base pathology involving the sellar region prioritizes protecting the afferent visual pathways. Rehabilitation techniques focus on patients with acuity and visual field loss, as well as double vision and ptosis. Patients with ocular malalignment related to either direct orbital involvement or involvement of the ocular motor nerves can be treated with short-term therapy, including occlusion, botulinum toxin injection, and prisms. Long-term management includes strabismus surgery designed to maximize the field of binocular single vision. Sensory loss to the anterior segment can compromise corneal function and needs to be addressed with appropriate intervention to open and close the lids, provide adequate tear production and corneal wetting, and deal with the potential loss of neurotrophic factors secondary to corneal denervation leading to corneal epithelial breakdown. The sellar region represents not only the center of the skull base but also the primary ophthalmologic conjunction. Both the afferent visual pathways, represented by the two optic nerves, chiasm, and optic tract, and the efferent visual pathways, including the third, fourth, and sixth cranial nerves, course either above or lateral to the sella. It is thus not surprising that pathology affecting the sellar and parasellar region often presents with ophthalmic manifestations,1–13 and any intervention, be it medical, surgical, or radiologic, can produce its own set of ophthalmic complications14,15 From an ophthalmic point of view, it is important to recognize the visual system in three parts. The afferent system can be thought of as a mapping function, taking the outside world into our consciousness. To perform this function adequately, the anterior segment of the eye needs to refract (bend and focus) light onto the photosensitive retina, which then converts the electromagnetic radiation into a series of impulses. These impulse signals are conducted via the optic nerves and visual pathways back to the cortex, where interpretation takes place. Interruption anywhere along these pathways can result in symptoms of afferent system dysfunction, including decreased vision, blurred vision, and visual field defects. The efferent system, responsible for coordinating ocular motility, provides globe movement through the final common pathways of the six extraocular muscles. These are innervated by the third, fourth, and sixth cranial nerves, which travel through the cavernous sinus lateral to the wall of the body of the sphenoid and enter the orbit through the superior orbital fissure. Involvement of these cranial nerves can result in loss of ocular motility, manifested as double vision, blurred vision, or a sensation of spontaneous ocular motility. Finally, the globe is supported by the orbital tissues making up the adnexal structures. These include the eyelids, responsible for maintaining and distributing an adequate tear film over the cornea, which forms the first part of the refracting surface of the eye. Adnexal tissues also include the lacrimal glands, the accessory lacrimal glands, and the structures responsible for opening and closing the eyes. Any involvement of the innervation of these structures can also result in various ophthalmic symptoms, including pain, numbness, loss of vision, problems with acuity, and double vision. Direct involvement of the orbital structures due to invasion of the orbit, either through the fissures and foramina connecting the intracranial cavities to the orbit or through the bone itself, can result in restricted motility, often producing double vision, proptosis (ie, prominence of the globe), and problems with anterior segment function. Thus, pathology affecting the sellar and parasellar region frequently results in multiple ophthalmic symptoms and signs.16 The autonomic nervous system has both afferent and efferent system effects. Sympathetic and parasympathetic innervation to the pupil controls the amount of light reaching the retina, and the parasympathetic innervation to the ciliary body is responsible for accommodation (ie, the ability to see close up). Sympathetic innervation to Müller’s muscle helps lift the lid up, and parasympathetic supply to the lacrimal gland controls tear formation and, thus, the anterior refracting surface of the eye. The history of visual symptoms often provides a clue to the presence of parasellar lesions and directs decisions regarding imaging. The most common ophthalmic manifestation of pathology affecting the suprasellar region is visual loss. Compression or secondary ischemic involvement of the optic nerve, chiasm, or optic tracts often produces decreased vision or less commonly appreciated visual field defects.14,17 The most important question is whether this is in one eye or both eyes. Loss of vision restricted to one eye implies globe, retina, or optic nerve pathology. If both eyes are involved, there still may be bilateral globe, retina, or optic nerve pathology, but chiasmal or retrochiasmal lesions are also in the differential diagnosis. The next question is that of the onset. Answers should include whether the visual loss is diffuse, central, or off to one side, and whether it is constant or intermittent. If variable, one needs to inquire as to what can make it better or worse, and whether it is progressive—either acutely, stepwise, or slowly. Any other associated neurologic or systemic complaints should also be sought. Patients should always be asked about a prior history of vascular disease (hypertension, diabetes, hypercholesterolemia, angina, myocardial infarct, irregular heart beat, cardiac valvular disease, or a documented stroke); inflammatory disease (demyelinating disease, infections including zoster, autoimmune conditions); cancer; or migraine. The patient’s occupation may give a clue to exposure to toxins and agents that can affect pupil size or motility, as may a travel history. It is helpful if the patient has had prior imaging studies. History often supplies clues to “where” the pathology is, which in turn alters the differential diagnosis. As with all complaints, rule No. 1 is essential: “Get the old records.” Often, patients with complaints of visual loss will be found to have previous compromise of the visual pathways, manifested by records documenting decreased vision. Attention should be directed to identifying any source of prior ophthalmic evaluation, including the records of previous ophthalmologists and optometrists, or even the results of visual screening, such as for the Department of Motor Vehicles or military. Previous refractive correction needs to be identified, including the potential problems of nearsightedness, farsightedness, and astigmatism. If the patient wears glasses or contact lenses, these should be available and all testing done with them in place. Bitemporal visual field defects are often unnoticed,18 and homonymous defects are often identified incorrectly as loss of vision in the ipsilateral eye. Patients may describe objects “disappearing” off to one side while they are reading. It is important to identify which parts of words seem to be disappearing as a clue to a visual field defect. It would be unusual for parasellar pathology to present with positive visual phenomena. Positive visual phenomena, often described as zigzag lines, lights, colors, a kaleidoscope, cracked glass, or broken images, are most commonly seen with migraine. They can uncommonly be seen with cortical pathology. Rare types of formed visual hallucinations usually occur with occipital involvement, but deafferentation anywhere along the visual pathways, including the optic nerve, chiasm, and tract, may result in release phenomena as known as the Charles Bonnet syndrome. Distortion of vision (seeing lines as bent, warped, broken, or missing, or seeing objects as too large or too small) may represent cortical pathology but is usually due to pathology affecting the retina, more specifically the macula. This can be seen with parasellar lesions that extend to involve the orbit but would be uncommon. Additional uncommon manifestations of pathology affecting the afferent system are visual obscurations.19 These are brief, usually bilateral loss of vision, often associated with change in position. This is typical in patients with disc edema, and it may be seen with parasellar lesions that cause increased intracranial pressure. Transient visual loss associated with exercise may represent long-standing inflammation involving the optic nerves (Uhthoff phenomena20). Monocular loss of vision lasting minutes may also occur with thromboembolic phenomena (amaurosis fugax, usually related to carotid disease), or dimming or darkening of vision with ocular hypoperfusion (ocular ischemic syndrome or arteritis, particularly in the elderly). Less common symptoms include darkening of vision associated with exposure to bright lights. This can sometimes be seen in patients with carotid compromise, potentially with parasellar lesions. Less commonly, transient loss of vision may be due to ocular disease (angle closure, hyphema, dry eye) and very rarely to orbital disease (gaze-evoked amaurosis secondary to an orbital tumor). The second most common ophthalmic complaint associated with parasellar pathology is double vision.21 This should first be characterized as monocular or binocular, with the examiner specifically questioning whether it disappears when either eye is covered. Failure to resolve when either eye is covered indicates a monocular problem. Although this may occur as a cortical phenomenon (palinopsia), it usually represents ghost images secondary to high astigmatism due to corneal warping, surface pathology, or cataract. Double vision that resolves by covering either eye is usually due to ocular malalignment. Attention should be directed to whether or not the double vision increases with gaze in one particular direction, specifically looking to the right or to the left, and in the four corners (up right, down right, down left, and up left). Although the natural presumption in the case of incomitant deviations (deviations that change with direction of gaze) is that they are due to a cranial nerve palsy,22–25 it is important to recognize that alternative causes of paretic malalignment include neuromuscular transmission deficits (myasthenia gravis), and internuclear connection problems (brainstem) causing a skew deviation or internuclear ophthalmoplegia. Supranuclear palsies due to cortical or corticobulbar pathway pathology may limit motility but usually do not produce malalignment or diplopia. Limitations secondary to supranuclear processes may be overcome with infranuclear stimulation (vestibular stimulation or irrigation of the ear [calorics]). In addition to paretic causes, incomitant ocular malalignment producing diplopia may also be due to restriction of the extraocular muscles. This requires involvement of the extraocular muscles themselves within the orbit and is most commonly seen in patients with thyroid orbitopathy. It can also be seen following trauma, with orbital inflammation, and with tumors that either extend to involve the extraocular muscles or are metastatic to the extraocular muscles or orbital tissues (particularly breast cancer). Restrictive phenomena should be suspected when there is history of orbital asymmetry, including proptosis, enophthalmos, exophthalmos, lid position abnormalities, prominent episcleral vessels, and a rushing sound in the patient’s head. Patients should be asked whether the double vision is side to side or if there is a vertical component. If the diplopia is strictly horizontal, then the problem must ultimately involve the two medial and lateral rectus muscles or their innervation. If there is a vertical component (indicating involvement of the vertical muscles [both obliques and the inferior and superior recti]), it needs to be addressed first. Patients should also be asked whether the double vision is worse at near or distance. Not all patients with ocular malalignment will experience double vision. This may be the case if there is a history of strabismus or poor vision in one eye. Similarly, subtle abnormalities in ocular alignment, often due to mild cranial nerve palsies, may not be appreciated as double vision, but rather as “blurred” vision when the patient looks in the direction of the weakened muscle. Patients will also often adopt head posturing, including head turns or tilts, to avoid the blurred or double vision. Old records should be sought for a previous history of strabismus, including records of eye muscle surgery and problems with ocular alignment in childhood. Congenital cranial nerve palsies may decompensate, producing the onset of double vision later in life. Clues to this may be provided by old photographs showing long-standing head posturing (particularly useful in the setting of a congenital fourth nerve palsy or Duane syndrome). Other, less common symptoms of efferent pathology include oscillopsia (a sensation of the world jumping), vertigo (a sensation of the world rotating), and tilt symptoms (a sensation of the world tilted, most commonly seen in Wallenberg lateral medullary plate syndrome owing to infarction of the posterior inferior cerebellar artery). These are usually due to pathology affecting the brainstem centers of stability and would thus be very uncommon with parasellar lesions. Adnexal involvement can be sought with a history of numbness or pain. Pain in and around the eyes unassociated with numbness is often unrevealing. A history of numbness, however, almost always indicates significant pathology. Prominence of the globe or fullness within the orbit may indicate intraorbital pathology, potentially extending from the parasellar region. A history of lid position abnormalities, including lid retraction or ptosis, may be a clue to pathology affecting the oculomotor nerve or the orbital tissues themselves. Afferent information is carried via the optic nerves, which consist of approximately 1.2 million axons of the ganglion cells located within the inner retina. Because the fovea is located nasal to the disc, fibers from the temporal portion of the retina arc around the macula, separating fibers that are just above the temporal horizontal midline from those just below. This anatomic separation occurs within the retina and at the disc and is maintained throughout the optic nerve. Thus, any pathology that affects the optic nerve tends to produce visual field defects that are either a little bit above or a little bit below the nasal horizontal midline on visual field testing (as we see things opposite to their anatomy). This is the anatomic origin of the arcuate visual field defects that we see with any pathology affecting the optic nerve. The fovea, which is the most sensitive portion of the retina, has the highest spatial resolution based on its relatively high (1:1 or 1:2) ganglion-to-receptor cell ratio, (in the peripheral retina, the ratio may be as low as 1:1000). In addition, the fovea, or the center of the macula, is devoid of rods and has the highest concentration of cones, responsible for color vision. Thus, color abnormalities are a common concomitant of the dysfunction of the relatively small macular fibers, which can be compromised with compressive lesions.26 Color vision may be affected relatively early in patients with mild compression of the optic nerve. Involvement of the small fibers, which are particularly sensitive to compression, may also result in relative central scotomas. The optic nerve is approximately 1.5 mm in diameter as it exits the globe, extends less than a millimeter through the sclera, then becomes myelinated, increasing in size to approximately 3 mm. It is surrounded by the optic nerve sheath and the trabecular arachnoid, which allows cerebrospinal fluid (CSF) up to the back of the eye. This access of CSF to the back of the globe is responsible for the potential development of disc edema secondary to increased intracranial pressure. There is redundancy in the optic nerve within the orbit, which permits the eye to move, but can be restricted if substantial proptosis ensues owing to invasion of the orbit. The central retinal artery and vein run within the optic nerve in the 8 to 10 mm just behind the globe, but outside the optic nerve posterior to this region, where the optic nerve is supplied by small branches from the optic nerve sheath. Extension of a meningioma along the optic nerve sheath can compromise the vascular supply to the optic nerve posteriorly. It also can compromise the venous outflow, resulting in the development of collaterals to the choroid circulation (optociliary shunt vessels)27 (Fig. 5.1). The optic canal measures 6 to 8 mm in length and extends superiorly and medially; it is bordered temporally by the optic strut, which separates it from the superior orbital fissure, and nasally by the sphenoid sinus. In approximately 8% of cases, there is bone dehiscence, so that the optic nerve actually may have no bony protection from pathology (or surgical exploration) within the sphenoid sinus. The exit of the optic canal takes place under a fold of dura, the falciform ligament. Pathology that elevates the optic nerve can thus compress the superior portion of the nerve, resulting in an inferior visual field defect. The intracranial portion of the optic nerve (uncovered by any dural sheath) is usually approximately 10 mm. The chiasm is variably placed, so that in the case of a preplaced chiasm, there may be a much shorter distance from the exit of the canal to the beginning of the chiasm. Similarly, in a relatively postplaced chiasm, the intracranial portion of the optic nerve may be longer, such that the chiasm actually rests posterior to as well as superior to the sella. This variability is responsible for the spectrum of clinical manifestations seen in suprasellar tumor involvement. In the setting of a relatively postplaced chiasm, tumors or other mass lesions of the parasellar region often involve the optic nerve, producing central scotomas and arcuate visual field defects (Fig. 5.2). In the setting of a preplaced chiasm, tumors that arise from the sella can compress the optic tract, producing homonymous visual field defects.17 The optic nerves meet and create the chiasm, which lies variably above the level of the sella. Because more of the pupillary fibers in the anterior visual pathways cross than do not cross, pathology affecting the optic tract may produce a contralateral afferent pupillary defect28 (Fig. 5.3). Damage to the tract may have a second distinguishing characteristic (not seen in cortical or optic radiation pathology) because retrograde degeneration will result in band (or bowtie) atrophy in the contralateral optic nerve head. It should be noted that this takes weeks to months to occur and will not be seen in the setting of an acute tract lesion, whereas the contralateral afferent pupillary defect develops immediately. The chiasm is usually inclined at about a 45-degree angle, so that pathology arising from the sella most frequently affects the anterior and inferior aspects of the chiasm, first producing the characteristic superior bitemporal visual field defects seen in most patients with suprasellar pathology29,30 (Fig. 5.4). The macular fibers tend to cross more posteriorly within the chiasm, and involvement of the posterior chiasm or the anterior third ventricle can result in relatively bitemporal scotomatous defects or bitemporal inferior field defects (Fig. 5.5). Recent studies have suggested that the looping of the crossing fibers into the contralateral optic nerve, described in earlier anatomy texts, is an artifact of the patients who were in the original studies (following enucleation or loss of an eye).31 Even though the so-called Wilbrand knee32 is probably an artifact, pathology that affects the optic nerve just anterior to the chiasm often produces a central scotoma with a relative temporal defect in the opposite eye. This, therefore, remains a useful localizing sign. Rarely, a lesion may compress the optic nerve without touching the anterior chiasm. This can produce a unilateral temporal field defect, referred to as the junctional syndrome of Traquair.33 Involvement of the posterior aspect of the chiasm is potentially seen with craniopharyngiomas or other pathology affecting the pituitary stalk, including sarcoid, histiocytosis, and germinomas. Even with posterior chiasmal compression, however, the majority of lesions are pituitary tumors because these are simply much more common. The efferent visual pathways originate within the horizontal and vertical gaze centers, located within the pons and midbrain, respectively. The horizontal gaze center itself is actually within the abducens nerve nucleus, although the burst cells, which are within the paramedian pontine reticular formation, generate horizontal saccades. Pathologic involvement of the horizontal gaze center results in absence of gaze to that side. The opposite eye can still adduct owing to convergent triggering of the medial rectus in the area of the midbrain. The vertical gaze centers are located within the dorsal rostral midbrain tegmentum. A set of three nuclei (the rostral interstitial nucleus of the medial longitudinal fasciculus [MLF], the interstitial nucleus of Cajal, and the nucleus of Darkschewitsch) are responsible for coordinating vertical gaze movements. Compression of the dorsal portion of the midbrain,34 such as is seen with extension of lesions of the pineal gland, can result in a classic dorsal midbrain syndrome, with absent up gaze, retraction convergence nystagmus, Collier’s sign of lid retraction, and light near dissociation due to involvement of the pupillary crossing fibers in the posterior commissure.35 Seesaw nystagmus is a rare finding in patients with parasellar lesions that extend to compress the midbrain.36 In this, one eye will elevate and intort, while the other eye depresses and extorts. This pattern then reverses. Signals from the horizontal and vertical gaze centers travel to the extraocular muscles via the third (oculomotor), fourth (trochlear), and sixth (abducens) cranial nerves. The sixth nerve exits the pontine brainstem to run in the subarachnoid space up the clivus. It pierces the dura and enters Dorello’s canal underneath the petroclinoid ligament (in conjunction with the inferior petrosal sinus), entering into the body of the cavernous sinus, where it crosses next to the carotid artery. The sympathetic fibers in the wall of the carotid artery leave the carotid within the cavernous sinus in conjunction with the sixth nerve to run more anteriorly before entering the superior orbital fissure along with branches of the first division of the fifth cranial nerve (nasociliary branch).37 The sixth nerve enters through the superior orbital fissure within the annulus of Zinn to innervate the lateral rectus muscle approximately one-third of the way anteriorly along its medial surface. The fourth, or trochlear, nerve nucleus is located just caudal to the third nerve nuclear complex within the tegmentum of the midbrain. Its branches run dorsally to cross in the superior medullary velum. It is the only cranial nerve that exits the brain dorsally and has the longest unprotected course in the subarachnoid space. It runs around the midbrain and travels along the edge of the tentorium anteriorly to enter the lateral wall of the cavernous sinus between the third nerve above it and the first division of the fifth nerve below it. The fourth nerve can be easily injured when the tentorium is opened (Fig. 5.6). The trochlear nerve is the only motor nerve that runs outside the annulus of Zinn, crossing over the optic nerve to innervate the superior oblique muscle approximately one-third along its course. Surgery to reach the optic nerve through the superior orbit can damage the trochlear nerve as it crosses in the orbital apex. Fig. 5.2 The fields (A,B) of a 65-year-old woman who presented with a 1-year history of progressive visual loss in the right eye. Visual acuity was 2/200 OD and 20/30 OS with a >1.8 log unit right afferent papillary defect. The right field (B) demonstrates a central scotoma, and the left field (A) demonstrates an inferior arcuate defect. Her funduscopic examination (C,D) revealed evidence of drusen of the optic nerve heads bilaterally with relative optic atrophy OD > OS. The view on the right (C) was much better than the view on the left (D) because she had had her cataract on the right side removed. The arcuate changes on the left eye were compatible with the buried drusen, and the difference between the total deviation plot and the pattern deviation plot was presumably related to her cataract. Fig. 5.2 (Continued) The central scotoma in the right eye, however, could not be explained by the optic nerve head drusen, and an angiogram revealed evidence of bilateral ophthalmic segment internal carotid artery aneurysms with right optic nerve compression (E). Evidence of bilateral optic neuropathies were confirmed on the optical coherence tomogram (OCT), which shows thinning of nerve fiber layer in both eyes (F). Fig. 5.2 (Continued) B-scan reveals hyperrefractile bodies in the optic nerve head (G,H), demonstrating that this is optic nerve head drusen, not papilledema. She underwent stenting and coil embolization of both carotid aneurysms (I) with stabilization of her visual function. Comment: Although central scotomas are most commonly associated with macular disease, certain optic neuropathies often cause central loss of vision. Rare causes include nutritional, toxic, and hereditary (Leber) optic neuropathies. Inflammatory and compressive lesions are more common causes of visual loss. In this case, a paraclinoid aneurysm compressed the right optic nerve, producing visual loss and a central scotoma. B-scan of the optic nerve head can help distinguish papilledema (disc elevation due to increased intracranial pressure) from optic nerve head drusen. Fig. 5.3 This 50-year-old patient was referred in September of 2008. Seventeen months earlier, she had developed a “funny smell” and was seen by her local doctor, who ordered MRI. This revealed evidence of a right temporal lobe lesion, and she underwent a craniotomy in May of 2007 (16 months before her evaluation) with subtotal resection of a high-grade glioma. Three days before her referral, she had noticed increasing problems seeing to the side. Visual fields reveal a fairly dense left homonymous hemianopsia (A,B). Visual acuity was preserved with 20/30 vision at distance and 4-point vision at near. She did, however, have a 0.9 log unit left afferent pupillary defect, indicating involvement of the right optic tract, which produced the left homonymous hemianopsia. Her discs at that time looked normal, and an optical coherence tomogram (OCT) of her nerve fiber layer also looked entirely normal (C). Fig. 5.3 (Continued) This 50-year-old patient was referred in September of 2008. Seventeen months earlier, she had developed a “funny smell” and was seen by her local doctor, who ordered MRI. This revealed evidence of a right temporal lobe lesion, and she underwent a craniotomy in May of 2007 (16 months before her evaluation) with subtotal resection of a high-grade glioma. Three days before her referral, she had noticed increasing problems seeing to the side. Repeat scan at this time revealed a lesion extending from the temporal lobe to involve the basal ganglia as well as the right optic tract (D–G). Three months later, she returned with visual acuity down to 1/200 in the right eye and 20/30 in the left. She now had a dense left homonymous hemianopsia. Her afferent pupillary defect had increased to 1.8 log unit. Fig. 5.3 (Continued) This 50-year-old patient was referred in September of 2008. Seventeen months earlier, she had developed a “funny smell” and was seen by her local doctor, who ordered MRI. This revealed evidence of a right temporal lobe lesion, and she underwent a craniotomy in May of 2007 (16 months before her evaluation) with subtotal resection of a high-grade glioma. Three days before her referral, she had noticed increasing problems seeing to the side. Three months later, she returned with visual acuity down to 1/200 in the right eye and 20/30 in the left. She now had a dense left homonymous hemianopsia. Her afferent pupillary defect had increased to 1.8 log unit. Although her optic discs did demonstrate some subtle optic atrophy, OD (H) greater than OS (I), the progressive damage to the anterior visual pathways could be better seen in her OCT, which showed severe thinning of the nerve fiber layer on the right side and moderate thinning of the nerve fiber layer on the left (J). Comment: Optic tract involvement may be suspected when there is an afferent pupillary defect on the same side as the visual defect. OCT, by giving us an in vivo biopsy, is a more quantitative way of assessing damage to the anterior afferent visual pathways. Although it is likely that when we first saw her she already had ongoing damage to the optic tract, there is a delay in the development of thinning of the nerve fiber layer and subsequent optic atrophy. The quantitation of her afferent pupillary defect also demonstrated progression, indicating involvement not only of the right optic tract but also subsequently of the right optic nerve in this patient with an aggressive glioblastoma. Fig. 5.4 This 40-year-old woman presented with a 3-week history of headaches and 2 weeks of blurred vision. Visual acuity was found to be 20/40 and 20/200 with near vision of 4 point and 26 point. Her visual fields revealed asymmetric bitemporal visual field defect (A,B). Interestingly, her discs really did not look that abnormal (C,D), and her optical coherence tomogram (OCT) showed normal nerve fiber layer thickness (E). Fig. 5.4 (Continued) This 40-year-old woman presented with a 3-week history of headaches and 2 weeks of blurred vision. Visual acuity was found to be 20/40 and 20/200 with near vision of 4 points and 26 points. Interestingly, her discs really did not look that abnormal (C,D), and her optical coherence tomogram (OCT) showed normal nerve fiber layer thickness (E). Her MRI revealed a suprasellar mass lesion compressing the chiasm (F–H). Fig. 5.4 (Continued) This 40-year-old woman presented with a 3-week history of headaches and 2 weeks of blurred vision. Visual acuity was found to be 20/40 and 20/200 with near vision of 4 points and 26 points. Her prolactin was elevated at 739 ng/mL. She was started on cabergoline (Dostinex) 0.5-mg tablets twice a week, and 6 weeks later she had dramatic improvement in automated static perimetry (I,J). Comment: Superior bitemporal defects are the most common chiasmal syndrome due to compression of the anterior inferior chiasm by a lesion arising from the sella. Elevated prolactin indicated a prolactinoma, which responded well to medical treatment with cabergoline. Quantitative improvement in perimetry is common with medical or surgical decompression of the chiasm. The normal OCT indicated that the compression was probably not long-standing and might be a good prognostic indicator for visual recovery. Fig. 5.5 This 53-year-old patient was referred to the neuro-ophthalmology office because of “another tumor.” Her visual fields demonstrated an inferior bitemporal visual field defect (A,B), and her optic discs indicated optic atrophy (C,D). Fig. 5.5 (Continued) This 53-year-old patient was referred to the neuro-ophthalmology office because of “another tumor.” Her optical coherence tomogram (OCT) confirmed the presence of bilateral optic atrophy with nerve fiber bundle dropout, more on the left than the right (E). Her past medical history was remarkable for galactorrhea in 1992. She was found to have an elevated prolactin level and in June of 1995 had undergone transsphenoidal resection of a cystic nonsecretory pituitary tumor. Review of her MRI demonstrated a cystic lesion arising from the sella and compressing the posterior aspect of the chiasm (F–H). Fig. 5.5 (Continued) This 53-year-old patient was referred to the neuro-ophthalmology office because of “another tumor.” She returned 6 months later stating that her vision had not changed, but automated static perimetry now demonstrated denser bitemporal visual field defects (I,J), though her discs showed no change (K,L). Fig. 5.5 (Continued) This 53-year-old patient was referred to the neuro-ophthalmology office because of “another tumor.” OCT demonstrated further thinning of her nerve fiber layer on the right side (M). She underwent decompression of what pathologically proved to be a Rathke cleft cyst. Within 6 months, her visual fields had improved, although still with a residual bitemporal defect (N,O). Fig. 5.5 (Continued) This 53-year-old patient was referred to the neuro-ophthalmology office because of “another tumor.” OCT showed still further thinning of her nerve fiber layer (P). Comment: In the setting of a preplaced chiasm or with a posteriorly oriented suprasellar lesion, compression of the posterior aspect of the chiasm produces an inferior bitemporal defect. OCT thinning is delayed, and it is not unusual following decompression for the fields to improve but the OCT to show progressive thinning. The oculomotor nerve originates within the tegmentum of the midbrain just rostral to the fourth nerve and just caudal to the vertical gaze centers. Several subnuclei make up the oculomotor nucleus, including a single central subnucleus for the levators of both eyes and a pair of subnuclei for the parasympathetics (Edinger-Westphal nuclei) innervating the sphincter of the iris and ciliary body. The superior rectus subnuclei send out fibers that cross over to the opposite side. The medial rectus subnucleus has been divided into several areas and probably has a subsubnucleus responsible for convergence, although this has been poorly identified. The third nerve exits on the ventral aspect of the midbrain, having passed through the area of the red nucleus, as well as the cortical spinal pathways in the peduncle. There are multiple rootlets as the third nerve exits the brainstem, and the nerve itself passes between the superior cerebellar artery below and the posterior cerebral artery above. The nerve runs parallel to the posterior communicating artery (where it can be affected by an aneurysm) and enters the superior lateral aspect of the cavernous sinus. There is a variable sheath that makes up the inner lateral wall of the cavernous sinus. Within the lateral wall of the cavernous sinus, the third nerve usually divides into a superior and an inferior division. These both enter the superior orbital fissure within the annulus of Zinn. The superior division innervates the superior rectus and levator muscles on their inferior surfaces. The inferior division innervates the medial rectus and the inferior rectus approximately one-third of the way along their inner surfaces and the inferior oblique muscle at its midpoint, just lateral to the border of the inferior rectus. An additional branch containing the parasympathetics to the iris sphincter and ciliary muscles runs with the inferior division and synapses in the ciliary ganglion at the lateral aspect of the optic nerve in the orbital apex. The short posterior ciliary nerves then convey the parasympathetic innervation to the globe and extend anteriorly to the sphincter and ciliary muscles. The adnexal structures include the globe itself, which consists of a firm scleral shell composed of collagen and a specialized anterior portion, the cornea, which is optically clear. The cornea is kept clear by the action of the endothelial pump, which returns interstromal fluid into the anterior chamber, relatively dehydrating the corneal stroma. The inner retina is perfused through the central retinal artery, which branches into a superior and inferior arcade. The blood supply to the outer retina (the rods and cones) is through the choroid. The choroidal blood actually makes up more than 80% of the blood circulating through the eye and is supplied through the posterior ciliary arteries around the optic nerve and the anterior ciliary arteries, which run within the rectus muscles. The retinal pigment epithelium, which underlies the retinal receptors, is responsible for support and also plays an active role in the metabolic pathways that are required for the rods and cones to function. The choroid is the posterior extent of the uveal tract. More anteriorly, the uveal tract consists of the ciliary body, which is responsible for forming aqueous (which keeps the eye inflated and supplies oxygen and nutrition to the nonvascularized lens and corneal endothelium), and the iris, which regulates the amount of light coming into the eye. Zonules, which originate from the ciliary body, support the intraocular lens. Contraction of the ciliary body releases tension on the lens, which then increases its refractive power by becoming rounder (permitting us to see objects up close). The surface of the eye is covered by a nonkeratinized epithelial layer that is contiguous from the cornea through the conjunctiva, lining both the surface of the globe (bulbar) and the inner surface of the eyelids (tarsal). The initial refracting surface of the eye is the tear film, which is supplied by the accessory lacrimal glands lining the upper tarsus and within the cul de sac both superiorly and inferiorly. The main lacrimal gland, located supratemporally in the anterior orbit, drains into the superior cul de sac. Although the main lacrimal gland is responsible for reflex tearing, it also supplies some basic tear production. The main lacrimal gland is innervated by the parasympathetics, which originate near the salivary nucleus. These fibers leave the brainstem in the nervus intermedius and join the seventh nerve. At the geniculate ganglion, they course with the greater superficial petrosal nerve, which parallels the carotid artery within the petrous bone, to the area of the foramen lacerum, where they join the vidian nerve. They synapse in the sphenopalatine ganglion. The postganglionic fibers then run through the inferior orbital fissure before joining the lacrimal nerve to the lacrimal gland. The eyelids are another important adnexal structure. As the outer surface of skin is keratinized, it is critical that the lids be held in an appropriate position and the keratinized surface not come in contact with the cornea. This is accomplished through the tarsus, a fibrous tissue that keeps the lids oriented. The lids are held tightly in position by the medial and lateral canthal tendons attached to the bone and are elevated by the levator and Müller’s muscles superiorly. The lids are closed by the orbicularis muscle, innervated by the temporal and zygomatic branches of the seventh nerve. The pretarsal orbicularis is largely responsible for reflex blinking, whereas the remainder of the preseptal and orbital orbicularis is responsible for forced lid closure. The tarsus also contains meibomian glands, which secrete an oily film that decreases tear evaporation. Goblet cells contained within the perilimbal conjunctiva are responsible for wetting the cornea, which is otherwise hydrophobic. The globe is moved within the orbit by six extraocular muscles: the four rectus muscles (medial, lateral, superior, and inferior) and the two oblique muscles (superior and inferior). The rectus muscles insert on the anterior aspect of the globe and will pull the eye in the direction in which they insert. The superior rectus produces elevation as well as adduction and incyclotorsion. The inferior rectus causes depression, adduction, and excyclotorsion. The oblique muscles, because they insert posterior to the equator, move the eye in the opposite direction, with the superior oblique muscle causing depression as well as incyclotortion and abduction. The inferior oblique moves the eye up and produces excyclotortion and abduction. The eye is permitted to move because of its encasement within Tenon’s capsule, which isolates the globe from the surrounding structures. The sheaths of the extraocular muscles also permit movement. These sheaths form a portion of the complicated septal anatomy within the orbit, with pockets of fat surrounding and protecting the extraocular muscles, as well as the cranial nerves and the vascular supply to the eye, muscles, optic nerve, and eyelids anteriorly. Any damage to the tissue planes often results in restricted motility and diplopia. The orbit communicates with the intracranial space through the optic canal, which transmits the optic nerve as well as the ophthalmic artery, and the superior orbital fissure, which transmits the ocular motor cranial nerves as well as the three branches of the first division of the trigeminal nerve, the superior ophthalmic vein, and various sympathetic and parasympathetic innervation to the structures within the orbit. The orbit also communicates inferiorly with the nose, allowing drainage of the excess tears through the lacrimal sac and the nasolacrimal duct. Collateral circulation links the ophthalmic artery (branch of the internal carotid artery) to multiple branches of the external circulation via the anterior and posterior ethmoid arteries, the recurrent meningeal artery through the superior orbital fissure, and the terminal branches of the supraorbital, supratrochlear, and infratrochlear arteries with the terminal branches of the angular, transverse facial, and temporal arteries. A basic understanding of the neuro-ophthalmic examination includes qualitative and quantitative assessment of the afferent and efferent systems. Multiple texts and chapters have addressed the neuro-ophthalmic evaluation of the afferent system.15,33,38–44 Ophthalmic evaluations, like all examinations, can be divided into those that are qualitative, potentially localizing in nature, and those that are quantitative, providing for an assessment of the natural history of disease as well as the effects of intervention. Qualitative assessment is often adequate to confirm the presence of a lesion and to direct additional work-up. Quantitative assessment is required, however, to determine whether or not lesions are changing over time, and whether therapy has been effective. Although qualitative acuity measurements may be listed as “normal” or “reduced,” in almost all circumstances quantitative measurement of afferent visual function should be obtained. It is important to recognize that central vision as measured by Snellen or near acuity represents only macular function, and it does not assess visual perception outside the point of fixation. Macular function is traditionally recorded as the smallest Snellen optotype that can be seen, usually at the fixed distance of 20 ft. The top of the fraction recorded (eg, 20/40) indicates the testing distance and the bottom smallest optotype that can be seen. Thus, in this case, at 20 ft the patient cannot see anything smaller than the 40 optotype. The optotype is defined by the size of the letter required to subtend 5 minutes of arc at the named distance. Small improvements in distance acuity testing were introduced by Bailey and Lovie in 1976, and a modification of the chart (also called the ETDRS [Early Treatment Diabetic Retinopathy Study] chart), is often used in multicenter trials.45 All visual function testing should be obtained with the patient’s correction (glasses or contact lenses) in place. Often, acuity seems to be lost simply because the patients are not wearing their glasses. A rapid screen for the presence of refractive abnormalities, as well as corneal or surface anomalies, can be obtained with the use of a pinhole. Improvement in central visual function when the patient looks through a pinhole usually indicates that at least a component of the decreased vision is related to uncorrected refractive error, or potentially to early corneal or lens pathology. In addition to best corrected distance acuity, near vision should be checked routinely (Fig. 5.7). Although this may be recorded as “equivalent,” as a general rule, it should be recorded in the point system, again with the appropriate refraction for near, and with a notation of the distance at which the patient is holding the reading card. The Jaeger system, although used by ophthalmologists, does not have the universal applicability (the point system is used in all type, including computer printouts) and therefore is less useful. Lack of equivalence between distance and near measurement is another clue to the possibility of an uncorrected refractive error, but it also may occur in patients with various types of cataract formation. An additional essential part of evaluation of the afferent system is testing extrafoveal function (visual fields).33,41 This can be done qualitatively by confrontation testing (Fig. 5.8), semiqualitatively on tangent screen testing, and quantitatively by Goldmann or automated static perimetry.46 At a minimum, all patients should be assessed for the potential presence of visual field defects that respect the vertical midline, indicating chiasmal or retrochiasmal pathology, or the nasal horizontal midline, indicating optic nerve pathology. In addition, as compressive lesions may affect the central portion of the visual field, patients should be tested for relative central visual field defects. These are common with optic nerve compression. Visual fields (like other afferent system tests) should be recorded as if you are the patient looking out. Thus, the right visual field is to the right side and the left is to the left, with the temporal fields peripherally and the nasal fields centrally. Visual field defects are particularly useful in localizing pathology, even without quantitative assessment. Bitemporal visual field defects, originally described clinically by McKenzie in 1835, clearly localize pathology to the area of the chiasm (Figs. 5.4 and 5.5). Homonymous visual field defects, in which there is a field defect to the same side in both eyes, indicates pathology affecting the post-chiasmal visual pathways (Fig. 5.3). Involvement of the optic nerves may produce either a central scotoma (Fig. 5.2) or variations of arcuate visual field defects, found on qualitative assessment by a relative step across the nasal horizontal midline. Arcuate defects are better evaluated in a more quantitative fashion either by Goldmann kinetic perimetry or automated static perimetry. In the interpretation of visual fields, the pattern deviation plot with statistical analysis is most important in terms of recognizing and appreciating the localizing value of visual field defects. Arcuate defects indicate pathology affecting the optic nerve (less likely the retina), whereas respect for the vertical midline indicates involvement at or behind the chiasm. Central scotomas not due to macular pathology are classically seen with toxic, metabolic, or hereditary pathology, but are not uncommon with compressive or inflammatory lesions. Evaluation of the afferent system also involves two additional studies: assessment of pupillary response and assessment of the retina and optic disc. Teleologically, the pupil is responsible for regulating the amount of light coming into the visual system.47 When there is excess light, the pupils become smaller, and when it is relatively dark, the pupils dilate. This is under control of the sphincter muscle, innervated through the third nerve, and the dilator muscles, innervated by the sympathetics traveling with the carotid artery through the superior orbital fissure. Pupils should be examined for regularity and symmetry. Pupil size may be measured with a pupil gauge. Asymmetry is not rare, essential anisocoria, but the difference should be the same in light and dark.48 The presence of asymmetric optic nerve pathology produces an afferent pupillary defect; moving a bright light from one side to the other results in dilation of both pupils when the light is moved to the side where the optic nerve is involved while the patient fixes a distant accommodative target.49 The presence of an afferent pupillary defect may be further quantitated by the placement of neutral-density filters in front of the better-functioning eye until the response is balanced50 (Fig. 5.9). Although an afferent pupillary defect cannot be detected in a patient with one eye, it is possible to detect an afferent pupillary defect even when there is only one working pupil. Statements about “reverse Marcus Gunn” pupil should be avoided. No matter which pupil is being observed, the relative afferent pupillary defect indicates the side on which less light is getting into the system. The presence of an afferent pupillary defect parallels asymmetric visual fields more than central acuity and optic nerve appearance. The eye gives us a unique ability to see in vivo into the functioning of the central nervous system. Funduscopic evaluation permits us to look at the beginning of the optic nerve, which is really the most anterior portion of the white matter tract of the brain connecting the photoreceptors via the ganglion cells to the geniculate and then the cortex. The presence of increased intracranial pressure, potentially seen in patients with parasellar lesions, can produce disc edema as it results in constipation of axonal transport (Fig. 5.10). Persistent disc edema due to increased intracranial pressure will eventually produce optic atrophy associated with deteriorating afferent visual function. This may be documented with photographs of the posterior pole and can be qualitatively assessed based on relative disc pallor and more recently quantitatively assessed with OCT (optical coherence tomography; Fig. 5.5). Papilledema is uncommonly seen with pituitary tumors unless they become large enough to obstruct the foramen of Monro. Other tumors arising in the parasellar region, however, more frequently present with increased intracranial pressures, especially meningiomas arising from the olfactory groove, from the tuberculum, or even from the anterior clinoid and medial sphenoid wing. These may present with bilateral disc edema due to increased intracranial pressure or unilateral disc edema and contralateral optic atrophy (Foster Kennedy syndrome) due to compression of one optic nerve.51 It should be noted that since the advent of imaging studies, the incidence of disc edema with all intracranial tumors has markedly decreased.14 This would be particularly true in patients with parasellar lesions, as it would be unusual for patients to be asymptomatic long enough for these lesions to reach the size that leads to papilledema. Fig. 5.9 In the presence of an afferent pupillary defect, neutral density filters may be placed in front of the better-functioning eye to quantitate the amount of asymmetry between the two eyes. It is important to use a bright light and spend equal time in the two eyes. Fig. 5.10 This 39-year-old patient presented in August 2006 with a 1-month history of pounding headaches. Visual acuity was 20/20 bilaterally, but his visual fields demonstrated enlargement of the blind spot bilaterally, worse on the left (A) than the right (B), and bilateral disc edema (C,D). Fig. 5.10 (Continued) This 39-year-old patient presented in August 2006 with a 1-month history of pounding headaches. Visual acuity was 20/20 bilaterally, but his visual fields demonstrated enlargement of the blind spot bilaterally, worse on the left (A) than the right (B), and bilateral disc edema (C,D). Optical coherence tomography (OCT) confirmed the presence of marked thickening of the nerve fiber layer bilaterally, worse on the right than the left (E). Fig. 5.10 (Continued) This 39-year-old patient presented in August 2006 with a 1-month history of pounding headaches. MRI revealed evidence of significant hydrocephalus, with a small colloid cyst blocking the foramen of Monro (F–I). Fig. 5.10 (Continued) This 39-year-old patient presented in August 2006 with a 1-month history of pounding headaches. Following excision of the colloid cyst, the discs are seen to return to normal (J,K), and the OCT shows marked thinning with return to normal (L). Comment: Obstruction of ventricular flow in this case resulted in secondary increased intracranial pressure with bilateral disc edema and bilateral cecal scotomas. With restoration of the normal CSF flow, pressure was reduced, the disc edema resolved, and the thickening of the nerve fiber layer reversed (as seen on OCT). The recent development of OCT has opened a new door to the more quantitative assessment of the optic nerve, disc, and nerve fiber layer. This can be seen to be thickened in patients with disc edema of any etiology (Fig. 5.10) and, more importantly, can quantitate the presence of loss of nerve fiber layer due to pathology that affects the optic nerve (paralleling optic atrophy; Fig. 5.5). It should be noted that there is a delay in the thinning of the nerve fiber layer for weeks, if not months, between the acute onset of optic nerve pathology and thinning seen on OCT. It is also important to note that the OCT will not thin to zero because there are support structures within the retina that will keep nerve fiber layer thickness measurements at least in the upper 30s or lower 40s range. Asymmetry between the two sides may be a useful sign of previous damage to one optic nerve. A finding of nerve fiber layer thinning may also indicate that a lesion is much more long-standing than otherwise appreciated (Fig. 5.3). OCT may also be prognostic in terms of visual recovery following surgical or medical decompression (Fig. 5.4). Just as the older literature suggested that the best prognosis was in patients with relatively brief visual loss and the absence of optic atrophy on funduscopic evaluation, the lack of OCT thinning may also be taken as a relatively good prognostic sign for potential recovery. Conversely, the presence of significant thinning of nerve fiber layer may indicate a worsened prognosis for recovery following decompression. It should be emphasized, however, that even with significant thinning, there may be substantial improvement in optic nerve function, particularly as measured by central acuity, but even by visual fields. It is important to note that not all decreased vision is due to optic nerve or chiasmal pathology. Abnormalities in refraction and abnormalities of the cornea, lens, vitreous, or retina can also produce decreased vision. This will often require more detailed work-up. One of the tests that may be particularly suggestive of an alternative explanation for visual loss would be the absence of an afferent pupillary defect. Although an afferent may not be present if both optic nerves are involved, the presence of unilateral decreased vision but no afferent pupillary defect strongly suggests either an anterior segment or a retina problem. In photo-stress test a light is shined in the eye for 10 seconds, then the patient is timed until he or she can read down to one line worse than best previoiusly recorded vision. This may be a subtle way of detecting a relative macular problem as differentiated from an optic nerve problem.52 Patients who have macular pathology will have a delay in their recovery, whereas patients with optic nerve problems will not. The view of the back of the eye may also be very helpful. If there is an anterior segment problem, the observer often cannot see in with a direct ophthalmoscope (Fig. 5.11), and therefore, it is not surprising the patient cannot see out. Similarly, clear macular pathology may explain the patient’s visual limitation. The appearance of the macula, unfortunately, does not always indicate its function. Additional functional tests, such as multifocal electroretinography (ERG) and autofluorescence, and anatomic tests, such OCT of the macula, may be necessary. One unusual symptom of a dense bitemporal visual field defect is the presence of postfixational blindness. When a patient converges his visual axes to look at a near target, anything beyond that near target disappears. This is not usually noticed by the patient but can be easily demonstrated on examination. Extensive literature addresses the importance of ocular motility to visual function.21,53,54 Because we are foveate animals, to maximize our visual potential, we need to bring the eyes into alignment with the object of interest. To do that, we have developed the efferent visual system, which allows the movement of each eye. In addition, because we have overlapping visual fields, both eyes must be in alignment simultaneously. Acuity is adversely affected by movement of the eye relative to the visual environment. Thus, it is critical that the eyes be kept stable. This includes stability when the head moves and when the object of interest moves. Stability can be best judged by observing the fundus with a direct ophthalmoscope.55 Any tendency for the eye to drift will be picked up as a movement of the observed optic disc. This can be done in primary and eccentric gaze. Gross instability may be directly observed, magnified with a Fenzel viewing system, or recorded with an eye-tracking system (electro-oculogram [EOG], infrared, coil).56 Each individual movement of the eyes is referred to as a duction. Ductions can be qualitatively assessed by simply looking at ocular excursion but also by looking to see if “the sclera can be buried.” Ductions may also be quantitatively assessed by measurement linearly, by rotational measurements with prism, or most accurately by measuring ductions on a hemispheric bowl (Goldmann apparatus) or an arc perimeter. These measurements may be particularly helpful in following patients who are likely to develop progressive limitation in individual ocular motility, such as those with infiltration of the extraocular muscles, or in the setting of disease processes such as myasthenia gravis and progressive external ophthalmoplegia. Normally, a patient should be able to abduct and adduct approximately 50 degrees; depression is approximately 45 degrees, and elevation decreases from a maximum of 40 degrees in childhood to 15 degrees in the elderly. Movement of the two eyes together is referred to as version. These may be movements left (levoversion), right (dextroversion), up (sursumversion), or down (deorsum-version). Vergence refers to movements of the eyes in opposite directions. Convergence is necessary to look at a near target, bringing the axes closer together, and divergence is required for looking at a distance target. Abnormalities of convergence and divergence can occur with midbrain lesions and can uncommonly be seen with parasellar lesions that enlarge to affect or involve the midbrain. Abnormal versions can lead to ocular malalignment. This, as mentioned, usually results in diplopia. Subtle abnormalities in versions can be picked up by dissociative tests such as the red glass test or, better yet, the use of a Maddox rod.57 A white light is viewed by one eye while a Maddox rod placed over the opposite eye produces the sensation of seeing a line perpendicular to the cylinders of the Maddox rod. To assess horizontal alignment, the Maddox rod is placed so that the cylinders are aligned horizontally, thus producing the sensation of seeing a line running vertically (Fig. 5.12A). If the light is not seen as on the line, then there is relative horizontal malalignment of the eyes. As the patient will see the light in the opposite direction to which the eye is deviated, if the patient reports seeing the light as crossed over the line, it means that the patient has a relative exodeviation, or deviation out. Similarly, if the patient reports the light on the same side as the eye viewing, the patient has the eyes relatively crossed in, or an esodeviation. If the Maddox rod is aligned vertically, the patient will see a horizontal line (Fig. 5.12B). If the light is above or below the line, there is a vertical deviation. A tendency to deviate (phoria), as picked up by a Maddox rod or red glass, is common in a large percentage of the population. Most people, however, maintain alignment by the use of fusional amplitudes, which can make up for a tendency of the eyes to deviate. A breakdown of the fusional amplitudes results in a manifest deviation (tropia). Torsional abnormalities (often seen when the third or fourth nerve is involved) can be measured with double Maddox rods. Occasionally, patients are unable to perform Maddox rod or other subjective tests. Alignment may be assessed by cross-cover testing and prism measurements or, in nonresponsive patients, by judging the position of the light reflex on the cornea (Hirshberg’s test). A more quantitative test not requiring patient response is Krimsky’s test, in which the light reflex is simultaneously centered on the cornea with the use of prisms, thus placing a number on deviation.58 As mentioned, not all patients who are tropic have diplopia; they may have facultative suppression, in which the brain simply ignores one image. This is usually seen in patients with a long history of malalignment, or congenital strabismus and amblyopia. Fusional amplitudes vary. They are much greater horizontally than vertically and are greater for convergence, bringing things in, than for divergence. The implications are that a patient who has a subtle problem with abduction (eg, a mild sixth nerve palsy) is more likely to be symptomatic than one with a subtle adduction problem (eg, an internuclear ophthalmoplegia). A small tendency to deviate vertically usually produces double vision because vertical fusional amplitudes are much less than horizontal, and most patients cannot fuse more than 3 or 4 diopters (1–2 degrees) of vertical malalignment. Fusional amplitudes require bilateral simultaneous vision. In the setting of a dense bitemporal defect, each eye is actually seeing half of the visual field, and any tendency to drift (a small hyperphoria that would otherwise be controlled) can result in double vision due to “hemifield slip.” Although the red glass test and Maddox rod are usually considered qualitative (assessing the presence of deviation but not quantitating it), it is possible to use them to quantitate deviation by adding a prism to balance the deviation. Quantitative assessment of ocular deviation may also be obtained by cross-cover testing in primary position, measuring both horizontal and vertical deviation with prisms and also the deviation in the other eight cardinal positions of gaze, including up, down, left, right, up right, down right, up left, and down left. Prism measurements will depend on which eye is under the prism. In incomitant deviations, when the patient fixates with the normal eye and the prisms are placed over the abnormal eye, one measures the “primary” deviation. If the prisms are then switched to the normal eye, the deviation measured when the abnormal eye is fixating is called the secondary deviation. The secondary deviation is always greater than the primary. In the setting of a comitant deviation (the same in all directions), the primary and secondary deviations are equal. In addition to the quantitative use of orthoptic or cross-cover testing with prisms, the use of a Lancaster red-green test59,60 or a Hess screen61 gives a semiquantitative assessment of ocular motility. This is particularly useful in following patients with ocular deviation (Fig. 5.13). An additional quantitative assessment of ocular deviation can be obtained by using a Goldmann bowl and plotting the area of binocular singularity. Thus, a patient with an abduction deficit, perhaps due to a sixth nerve palsy, may be seen to have double vision when looking in the direction of the sixth nerve dysfunction but single vision when looking away. By plotting the binocular single vision fields, patients can be followed to determine whether or not sixth nerve function is improving or worsening. It should be noted that finding a deviation, either qualitatively and quantitatively, does not tell you whether the deviation is due to a paretic problem (eg, a cranial nerve palsy, a neuromuscular transmission deficit, or an internuclear problem) or due to restrictive phenomena secondary to local orbital invasion. Restrictive syndromes are traditionally diagnosed by forced ductions but may also be recognized with the use of a suction cup62 or the elevation of intraocular pressure in eccentric gaze.63 Rarely, ocular malalignment may be due to primary overaction syndromes. Parasellar lesions that can produce overaction include ocular neuromyotonia, which often follows radiation of lesions in the parasellar region. Patients with ocular neuromyotonia can report a sensation of the eye “sticking” when they look in the direction of the affected muscle. In sixth nerve ocular neuromyotonia, when the patient looks to that side, the lateral rectus muscle will continue to fire even after the patient looks back straight, resulting in a transient exodeviation producing double vision. Fig. 5.13 This 24-year-old presented with a 4-month history of intermittent diplopia. Visual acuity and visual fields were normal, but she had upbeat nystagmus in primary position increasing on up gaze. MRI demonstrated a dorsal midbrain intra-axial lesion with an exophytic component (A,B). Fig. 5.13 (Continued) This 24-year-old presented with a 4-month history of intermittent diplopia. Visual acuity and visual fields were normal, but she had upbeat nystagmus in primary position increasing on up gaze. She underwent a suboccipital craniotomy and biopsy, which demonstrated a low-grade glioma. Postoperatively she had worsening of her diplopia, with an exo deviation increasing on gaze to either side (C) and a right hypertropia increasing on left gaze and with right head tilt. Hess screen confirmed the incomitant vertical deviation (D). Two months later, her double vision had significantly improved (E). Comment: Following craniotomy, this patient had evidence of bilateral internuclear ophthalmoplegia and a right fourth nerve palsy, which spontaneously improved. The Hess screen provides quantitative follow-up. Making the diagnosis of a cranial nerve palsy depends on whether or not it fits a pattern. A sixth nerve palsy should be seen as an esodeviation increasing on ipsilateral gaze and decreasing on contralateral gaze (Fig. 5.14). The deviation should be minimal when the patient looks away from the side of the sixth nerve. A fourth nerve palsy consists of an ipsilateral hyperdeviation that increases on contralateral gaze and ipsilateral head tilt (Fig. 5.6). The deviation should be minimal when the patient looks up and to the same side as the involved superior oblique. A third nerve palsy produces the pattern of an exodeviation increasing on contralateral gaze and a variable hyperdeviation with a contralateral hyperdeviation on up gaze and an ipsilateral hyperdeviation on down gaze (Fig. 5.15). This may be variable depending on whether or not there is divisional third nerve palsy. Two additional “fellow travelers,” the eyelid position and the pupil, should be assessed. Specifically, because the third nerve innervates the levator, there is usually a concomitant ptosis, and because the third nerve also controls the sphincter muscles of the iris, the pupil on that side is often larger and does not react normally. In the setting of a complete third nerve palsy, fourth nerve function can be determined by whether the patient has incyclotorsion while looking down. Small vessels at the limbus can be observed to see if they rotate in when the patient attempts to follow down. The finding of a concomitant fourth and third nerve palsy usually indicates cavernous sinus pathology. Multiple cranial nerve palsies may occur, particularly in the setting of cavernous sinus pathology secondary to parasellar extension.16,64,65 However, “pieces” of a third nerve palsy, such as isolated medial rectus or isolated inferior rectus weakness, are unlikely to be related to third nerve dysfunction. Instead, they more likely indicate other causes of ocular motor problems, including myasthenia gravis, skew deviation, and restrictive pathology. The pattern of deviation is particularly easily seen on a Hess screen but also can be assessed quickly with a Maddox rod or red glass. The acute onset of multiple cranial nerve palsies is characteristic of pituitary apoplexy.66–68 These are often bilateral and accompanied by involvement of the afferent visual pathways, resulting in decreased vision, afferent pupillary defect, and field defects plus associated mental status changes. This event was originally felt to be an uncommon condition related to bleeding into a preexisting pituitary tumor. The advent of imaging has indicated that small bleeds into pituitary tumors are far more common than previously documented, and most patients do not have a substantial change in their symptomatology. Nonetheless, variations of pituitary apoplexy still do occur, and the sudden onset of multiple cranial nerve palsies and afferent system dysfunction should bring that to mind. Fig. 5.14 This 78-year-old woman presented in September 2009 with double vision. Her afferent system was entirely intact, but her nine cardinal positions demonstrated an abduction deficit on the left (A). Fig. 5.14 (Continued) This 78-year-old woman presented in September 2009 with double vision. This is confirmed on her Hess screen, which shows a limitation in left abduction (B). CTA revealed a left carotid cavernous aneurysm (C). Her pupils were also noted to be unequal, with a miotic pupil on the left side and mild ptosis (D). Following Iopidine, the smaller left pupil now is larger, indicating hypersensitivity on the left (E). Comment: This is an example of a combined left sixth nerve and Horner syndrome secondary to a carotid artery aneurysm. The pattern of deviation is easily appreciated on the Hess screen. Adnexal assessment is addressed by measuring the palpebral fissure opening, usually with a millimeter ruler (Fig. 5.16). Upper lid range gives a clue to the function of the levator muscle and Müller’s muscle (Fig. 5.17 A,B). The presence of ptosis can be quantitated by measuring the distance between the center light reflex on the cornea and the upper lid margin. This median reflex distance (MRD; Fig. 5.18) decreases in patients with ptosis, or lid droop, and increases in patients with lid retraction. The presence of pathology within the orbit may be suspected by widening of the palpebral fissure, clear evidence of the eye bulging, or more quantitative evidence of relative proptosis or exophthalmos. An additional qualitative assessment may simply be obtained by looking over the patient’s forehead for evidence of increased prominence of the globe. Hertel measurements are used to quantitatively assess the distance from the orbital rim to the corneal plane (Fig. 5.19). Although the range for normal patients has been published (varying by population), far more valuable is the symmetry expected between the two sides. More than 2 mm of difference between the two eyes usually calls for an explanation. A previous history of trauma, inflammation, or orbital tumors should be sought before it is assumed that this is related to intraorbital extension of parasellar pathology. Proptosis that varies, particularly induced by Valsalva maneuver or bending forward, usually indicates a vascular anomaly such as a venous varix. Rarely, pulsations of the eye may be seen with loss of the bony confines of the orbit. This may be related to previous surgery but can occur with congenital, neoplastic, or vascular anomalies eroding the bone. Vascular abnormalities including carotid cavernous fistulae may also cause pulsations. One other clue to the presence of pathology involving the orbit itself may be obtained by balloting the eye. Resistance to retropulsion usually indicates pathology within the orbit. This is particularly useful when there is asymmetry between the two sides. In addition to axial displacement of the globe forward, the globe may be displaced inferiorly (dystopia) or in or out relative to the distance from the midline of the nose. This may indicate the origin of pathology affecting the globe and other adnexal structures. Displacement inferiorly usually indicates involvement of the orbit through the roof (Fig. 5.1). Displacement of the globe medially would indicate a more central skull base pathology, often entering the orbit through the ethmoid sinus and medial wall. Involvement of the lateral wing of the sphenoid can produce both axial proptosis and displacement of the globe medially or inferiorly. Rarely, masses may actually be palpated within the orbit. The anterior segment, including the conjunctiva, cornea, iris, anterior chamber, and lens, is best evaluated at the slit lamp. Although a penlight may be helpful, the slit lamp offers binocular magnification as well as oblique and transillumination (useful for detecting old inflammation). The pupils should be examined for irregularity and symmetry. If the pupils are unequal, they should be recorded in the light and dark. If the difference is greater in light, then the larger pupil is not constricting. Assuming there are no pharmacologic (red top dilating drops), inflammatory, or traumatic causes, one must distinguish between Adie’s pupil (postganglionic parasympathetic dysfunction, usually after viral infection or trauma, with vermiform iris movements, light-near dissociation, and tonic redilatation) and third nerve dysfunction, which should be associated with abnormal eye movements. If the pupillary difference is greater in the dark, then the smaller pupil is not dilating (Fig. 5.20). Both third nerve palsy and Horner syndrome are common symptoms of parasellar pathology affecting the cavernous sinus. A subtle dilation delay may be seen on serial photographs of the pupils taken after shutting offthe lights as a sign of sympathetic dysfunction (Horner syndrome).69 Horner syndrome may be confirmed by a lack of dilation to cocaine drops or by reversal of anisocoria following apraclonidine (Iopidine) drops (Figs. 5.14 and 5.20). Paredrine drops will dilate the pupil of a patient with a first- or second-order Horner syndrome (brainstem, neck, or lung apex), but not a third-order Horner syndrome (seen with parasellar disease). The larger pupil due to a third nerve palsy should react to 1% pilocarpine, whereas a pharmacologically dilated pupil will not. Fig. 5.16 A millimeter ruler can be used to measure the palpebral fissure opening. Two additional critical items in adnexal assessment include evaluation of the fifth cranial nerve and lid closure. Sensation should be checked qualitatively simply by asking the patient to compare sensation in the three divisions of the distribution of the trigeminal nerve. More quantitative assessment can be obtained with an esthesiometer (Fig. 5.21), measuring corneal sensitivity. This may be particularly useful in potentially progressive lesions affecting the first division of the fifth nerve, resulting in corneal hypesthesia. A second critical function is that of lid closure. Although lid closure may be affected by proptosis alone, most of the time, marked problems with lid closure are secondary to weakness in the orbicularis muscle. Neuromuscular transmission deficits should always be considered, as should primary involvement of the orbicularis muscle itself (mitochondrial myopathy, as seen in patients with chronic progressive external ophthalmoplegia). Most weakness of lid closure, including decreased blinks and incomplete blinks, is related to facial nerve weakness. The presence of facial nerve paresis in the setting of suspected parasellar pathology would indicate much more extensive involvement or more than a single lesion. Fig. 5.21 The esthesiometer may be used to quantitatively assess corneal sensitivity. The presence of facial nerve weakness combined with sensory loss has a particularly worrisome implication and needs to be recognized early in the care of patients with parasellar pathology. These patients are likely to develop problems with neurotrophic keratitis, resulting in corneal epithelial breakdown and potentially corneal infection and melt. Primary loss of sensation that is progressive often indicates neurotrophic spread of a skin cancer along the trigeminal nerve. This is particularly common in patients with squamous cell carcinoma and adenoid cystic carcinoma. Extension usually goes back to the cavernous sinus, with motility problems following the loss of sensation. Overaction of the orbicularis may occur in four settings. Most common is blepharospasm, in which increased lid closure bilaterally is often associated with other orofacial movements (Meige syndrome). Hemifacial spasm is usually unilateral and is associated with compression of the root exit zone of the facial nerve. Quivering movements of the face are seen with both facial myokymia (often due to intra-axial pathology such as multiple sclerosis) and benign facial fasciculations. Accompanying facial weakness is usually a sign of significant disease. Occasionally, the patient cannot initiate lid opening (apraxia of lid opening). An additional adenxal problem associated with parasellar lesions is that of alteration in tear production. Baseline tear production can be assessed by measuring the wetting of a filter strip (Schirmer’s test). Although most literature suggests that baseline tear secretion is unaffected by pathologic denervation of the parasympathetics to the lacrimal gland, lesions affecting the parasympathetics often result in at least mild reduction in baseline tear secretion as measured by asymmetry in Schirmer’s test. Even more importantly, surgical intervention can interrupt the parasympathetic pathways, resulting in decreased baseline tear production. Although the presence of lid retraction is most commonly seen with thyroid orbitopathy (less commonly with Collier’s sign, seen with dorsal midbrain syndrome), one important type of lid retraction occurs while the patients attempt to look down and in. This is usually seen in patients with preceding third nerve palsy and indicates aberrant regeneration of the third nerve (Fig. 5.22). Fibers that used to go to the medial rectus and inferior rectus are now misdirected to the levator. Other signs of aberrant regeneration include pupillary miosis on up and down gaze, persistent co-contraction of the superior and inferior recti (leading to restricted vertical movement), and adduction with elevation and depression. The lid elevation with depression and adduction is the most classic and obvious sign of aberrant regeneration. In rare cases, a slowly expanding parasellar lesion, such as a giant cavernous carotid aneurysm or meningioma, may produce aberrant regeneration without a history of an acute third nerve palsy. This form of “primary aberrant regeneration” is very suggestive of a slowly expanding parasellar lesion.70,71 Relative enophthalmos is most commonly due to previous trauma, including blowout fractures of the orbit or previous hemorrhage resulting in orbital fat atrophy. Chronic inflammation of the maxillary sinus can result in enlargement of the orbit and enophthalmos (so-called silent sinus syndrome). Metastatic breast carcinoma often can produce shrinkage of the fat, resulting in a combination of enophthalmos and restricted motility. Enlargement of the greater wing of the sphenoid, frequently related to meningioma, often presents with proptosis. However, involvement of the orbit from any direction, including through the superior orbital fissure, from and through the roof with hyperostosis, and from the sphenoid sinus and posterior ethmoids, can result in axial displacement (proptosis) as well as dystopia, with the globe displaced inferior or laterally, and less commonly medially or superiorly. Ability to improve compromised optic nerve function is limited. Unlike goldfish, which can regrow their optic nerves, at this point we have limited options to influence the recovery of optic nerve function. It should be pointed out that visual fields often will continue to improve for periods of up to a year or more following surgical decompression of optic nerve or chiasmal compression (Fig. 5.23). This can be seen with gradual improvement in visual field function in patients with pituitary tumors following surgery.72,73 It is likely that this functional improvement is separate from anatomic improvement because OCT thinning and optic atrophy tend to remain (Fig. 5.23). It is important to note that psychophysical functioning, electrophysiologic studies, and anatomic studies such as OCT and disc appearance, although paralleling each other, may not always be exactly linked. This is particularly obvious in patients with optic neuritis, whose central acuity and even visual fields may return to normal despite clear evidence of residual optic atrophy, OCT thinning, and electrophysiologic abnormalities, including prolonged latency on visual evoked potential. Thus, it is important to recognize that each of these assessments of afferent visual system does not replace the others. Patients with visual impairment can be helped with various low-vision aids. These may be low-tech, including simple high-plus magnifiers that allow patients to see small objects by holding them closer, or fairly sophisticated magnification systems, including computer-driven closed circuit TV monitors. Although substantial work is being done on bionic replacement for dysfunctional afferent visual pathways, at present this is strictly theoretic and research-driven. Low-vision aids, however, can be very useful if patients can define the exact function they would like to improve. Although it is often impossible to resupply visual acuity for some of the activities of daily living, such as driving, reading can be helped, as can functioning at particular defined distances. Visual field defects also represent a substantial challenge. Although there have been attempts at encouraging visual plasticity, it is unlikely that major field defects can be bypassed. Practice with visual field defects, however, can markedly improve the ability to read. Simple techniques such as orienting letters vertically, in the case of patients with homonymous visual field defects, can sometimes improve the ability to read,74,75 and the use of simple tricks, such as leading the eye proprioceptively with a finger or a ruler to find the next line, can be helpful in the rehabilitation of patients with marked visual field abnormalities. High-power prisms have been used to try to bring objects into a seeing field from a nonseeing field. This has limited applicability, however, and it certainly should not be promised as a way of restoring the ability to drive to patients with dense homonymous visual field defects. They may occasionally be useful to alert patients to events in their nonseeing field. Fig. 5.22 This 54-year-old woman was seen in March of 2009 with mild persistent double vision. On examination, she could see 20/20 bilaterally with 4-point and 3-point vision. Her visual fields were full. She did, however, have limitation in vertical gaze on the right and 1.5 mm of right ptosis, and when she looked down and to the left, her right eyelid was elevated (A). Hess screen confirmed limitation in vertical (particularly up) gaze (B). Binocular single vision fields demonstrated single vision when she looked straight ahead but double when she looked up or down (C). Eight months earlier, she had acutely developed a severe headache. CT revealed evidence of subarachnoid hemorrhage, and CTA demonstrated a right posterior communicating artery aneurysm (D). Fig. 5.22 (Continued) This 54-year-old woman was seen in March of 2009 with mild persistent double vision. On examination, she could see 20/20 bilaterally with 4-point and 3-point vision. Her visual fields were full. This was confirmed on angiography (E,F). She was treated with coil embolization (G). Comment: Aberrant regeneration is common following traumatic oculomotor palsies but is also not uncommon following aneurysmal ophthalmoplegia. The most common manifestation of aberrant regeneration of the third nerve is lid elevation with attempted adduction and depression. It is usually not possible to restore normal ductions. In the setting of restrictive strabismus, particularly after involvement of the orbit by a tumor or by a surgical approach, restrictive strabismus can be released by recessing the affected fibrotic muscle. This, unfortunately, does not usually improve ductions overall. In the setting of marked limitation of ductions, something that may occur following prolonged complete involvement of the third cranial nerve or more likely the sixth cranial nerve, in which the eye may gradually drift into abduction or adduction, the eye can be recentered with eye muscle surgery. This should be undertaken only once there is stability. A more common challenge is the problem of double vision, seen in patients with ocular malalignment. The most likely short-term solution is simply to occlude one eye. Although this sometimes takes some getting used to, patients can function perfectly well and can engage in most activities of daily living, including driving, with a single eye. The chief challenge early on is that of reaching for near objects because it is difficult to judge near distances. The loss of stereopsis, however, is usually not important for any distance substantially beyond arm’s length. Even for objects close up, over time patients learn to use monocular clues to more accurately judge distances. Although botulinum toxin (Botox) injections into the muscle opposite to the involved muscle can result in decreased contracture, it is unlikely that the patient will achieve substantial functional realignment because the effects tend to be variable, and injection of the extraocular muscles often results in inadvertent development of vertical movement problems. Prisms are seldom useful in the acute setting because most deviations secondary to ocular motor nerve palsies are markedly incomitant, meaning the deviation varies depending on where the patient is looking. Sometimes, however, realignment is possible, at least in primary position, and a second prism power in down reading gaze may allow them to use both eyes together. Fig. 5.23 These are the visual fields (A,B) of a 48-year-old woman who was referred with a 1-month history of decreasing vision. Visual acuity when evaluated was 20/30 on the right and 8/200 on the left, with a 0.3 log unit afferent pupillary defect. Her funduscopic examination was unremarkable, and optical coherence tomography (OCT) demonstrated a normal nerve fiber layer (C). Fig. 5.23 (Continued) MRI, however, revealed a suprasellar lesion compressing the chiasm and impacting both optic nerves (D,E). Her past medical history was remarkable for a 10-month history of polydipsia and polyuria, a 7-month history of amenorrhea, and a 1-month history of severe headaches, nausea, and vomiting. Transsphenoidal surgery demonstrated a craniopharyngioma, which was endoscopically decompressed. Follow-up visual fields 2 months later revealed dramatic improvement in the bitemporal defect (F,G), with visual acuity improving to 20/25 and 20/20. Fig. 5.23 (Continued) Despite the improvement in her fields, her OCT demonstrated thinning of the nerve fiber layer nasally in both eyes (H). Follow-up 6 months after that revealed further improvement in the bitemporal visual field defects (I,J), but with some residual optic atrophy (K,L) and further thinning of the nerve fiber layer, worse on the left than the right (M). Fig. 5.23 (Continued) Follow-up 6 months after that revealed further improvement in the bitemporal visual field defects (I,J), but with some residual optic atrophy (K,L) and further thinning of the nerve fiber layer, worse on the left than the right (M). Comment: The presence of a normal nerve fiber layer when the patient was first evaluated suggested that the compression of the chiasm was relatively acute. Recent studies suggest that this may be a good prognostic sign for improvement in both acuity and visual fields, as was the case here. Although acuity often improves immediately following decompression, visual fields may take months or more to show improvement. Despite the field improvement, however, she was left with residual optic atrophy and nerve fiber layer thinning. Over time, if the deviation does not clear or if we have been unable to improve things, there is a natural tendency for any residual deviation to become increasingly comitant. This so-called spread of comitance is due to the unequal innervation of the yoke muscles (violation of Hering’s law). This change makes it easier to correct any residual deviation with the use of prisms, or possibly with extraocular muscle surgery. It is imperative that patients remain stable before any sort of surgical intervention is considered. A minimum of 6 to 9 months is required before eye muscle surgery can be entertained. As mentioned, Botox injection to the extraocular muscles is sometimes useful, particularly to avoid contracture of shortening muscles due to unopposed action. This is particularly true in patients with complete sixth nerve palsies who develop progressive esodeviation that can be at least partially relieved or tempered by injecting the medial rectus muscle. This can be accomplished under direct electromyographic control; small doses of Botox can weaken the overacting medial rectus, at least over the short run, and reduce or mitigate further contracture. As mentioned, it is important to undertake a quantitative assessment of ocular motility, including quantitative use of a Maddox rod or cross-cover testing with prisms or, better yet, use of the Hess screen and binocular single vision fields to demonstrate stability, before eye muscle surgery is considered. Once the eye is stable, eye muscle surgery can be planned, particularly with use of the Hess screen. It is usually impossible to improve the ductions of a limited eye secondary to persistent residual cranial nerve palsy. This is particularly true in patients who develop aberrant regeneration of the third nerve. In this, persistent co-contraction of the superior and inferior rectus muscles limits vertical excursion. As it is impossible to rewire the eye, the only way of increasing binocularity is to limit the movement of the better-moving eye. Although this takes some explaining to the patient and family, it is possible to increase the area of binocularity and reduce diplopia. This is best accomplished through the use of posterior fixation sutures (Faden procedure), in which a combination of recessing the muscle and adding sutures placed well posteriorly limits the overall excursion of the better-moving eye (Fig. 5.24). Again, it is essential to make sure the eye is stable before this sort of intervention is considered. In the setting of a complete sixth nerve palsy, to provide a tonic abducting force, a transposition procedure can be accomplished, moving the superior and inferior rectus muscles to the insertion of the lateral (Fig. 5.25). This may be done as a full-thickness transposition or as a partial-thickness transposition, which may potentially spare some of the circulation to the anterior segment. The presence of pulleys controlling the position of the muscles76 limits the extent of transposition, and an important recent modification of this technique involves the use of posterior fixation sutures along the area of the globe equator. This moves the effectiveness of the transposition more posteriorly and results in a greater abducting effect than does a simple transposition procedure alone. This so-called Foster modification is part of the routine in moving the eye out following a complete sixth nerve palsy. A similar procedure may be used in the absence of elevation (double elevator palsy), in which the medial and lateral rectus muscles are moved superiorly to act as elevators. The pulley system controlling the extraocular muscles again limits the amount of transposition possible. Decisions about the type of extraocular muscle surgery usually depend on whether or not the defect is complete. In the setting of a complete sixth nerve palsy, the usual sequence would be to perform a transposition procedure to give some tonic abduction to the eye, followed by limiting procedures on the opposite yoke muscle (the medial rectus in the opposite eye). In the setting of an incomplete sixth nerve palsy, weakening of the medial rectus in the opposite eye, which would include a recession procedure and Faden operation, would be the primary surgical intervention. This can later be fine-tuned by a recession and resection of the various muscles on the involved side to move the eye into better alignment. It is also important to recognize that any subtle residual defect can be managed with prism in glasses, although ground-in prism is limited to approximately 6 degrees of malalignment (12 diopters of ground-in prism). Although larger Fresnel prisms may be applied to the glasses, these usually degrade acuity by blurring visual function. They are often not tolerated for lengths of time but can be tried as a temporary measure. Probably the most important aspect of adnexal rehabilitation is to provide for adequate lid function. In the setting of a seventh nerve palsy, it is critical to ensure lid closure to avoid neuroparalytic keratitis. Short-term measures include aggressive lubrication, including ointment at nighttime, and potentially taping the eye at night. During the day, adequate lubrication without blurring of the vision can be obtained with semiviscous solutions such as Refresh Liquigel and GenTeal Gel. Partial tarsorrhaphies or even central tarsorrhaphies can protect the globe in the short term if the patient has epithelial breakdown, particularly in the setting of combined facial nerve palsy and trigeminal nerve palsy. Failure of seventh nerve function to recover usually requires surgical intervention to help close the lid. Although it is still possible to implant an elastic band (Arion sling) or springs, the easiest way to help lid closure is with gravity assist by implantation of a gold or platinum weight. Although some weights can be applied externally, most of these are usually implanted in a pretarsal or, less commonly, preseptal location. Although the preseptal implants are less obvious cosmetically, they tend not to be as effective. Some surgeons will use a single-size weight, but it is usually advisable to tailor the gold weight to the particular status of the patient. I prefer to implant the heaviest weight that the patient can easily lift up, producing a minimal amount of ptosis. The heavier the weight, the more likely are good lid closure and eye protection. It is imperative to note that patients who are otherwise compromised and therefore supine in bed should never have a gold weight implanted because they cannot sit up to allow gravity to have an appropriate effect. In that setting, it is often better to simply perform a tarsorrhaphy to protect the eye. Fig. 5.24 In June of 2007 a 50-year-old woman was referred for diplopia. Her acuity and visual fields were normal, but she had evidence of an abduction deficit on the left side (A) with an esotropia increasing on left gaze (B). Binocular single vision fields (C) confirmed diplopia on left gaze. Seven months earlier, she had begun to have episodes of “voices in my head.” EEG demonstrated an epileptogenic focus, and MRI in April had revealed a sphenoid wing meningioma (D,E). Fig. 5.24 (Continued) In June of 2007 a 50-year-old woman was referred for diplopia. One month later, she had undergone pterional craniotomy and tumor resection. Repeat MRI demonstrated damage to the left lateral rectus muscle (F,G). One year later, when her motility failed to improve spontaneously, she underwent a right medial rectus recession and Faden procedure. This limited the adduction on the right to match the limited abduction on the left (H) and completely eliminated her double vision (I). Comment: With both paretic and restrictive causes of limitation in motility, it is often impossible to improve ductions. Although the eye can be straightened in primary position to maximize binocularity, surgery on the contralateral eye may be advisable. Fig. 5.25 This 48-year-old patient noted some ringing in her ears, and MRI demonstrated a parasellar right cavernous meningioma. She underwent pterional craniotomy and resection, which left her with a right IV, V, and VI cranial nerve palsy. Immediately following surgery, the abduction deficit could be seen on her nine cardinal positions (A), and the Hess screen (B) confirmed a problem with abduction. There was no recovery of nerve VI, and by 4 months following surgery she had developed 50 diopters of right esotropia (C). She was treated with injection of 5 units of Botox into the right medial rectus muscle, which resulted in a decrease in adduction on the right (D) as well as some inadvertent problems with down gaze on the right side, but her right eye did not deviate out at all, indicating that her nerve VI was still complete. Fig. 5.25 (Continued) This 48-year-old patient noted some ringing in her ears, and MRI demonstrated a parasellar right cavernous meningioma. She underwent pterional craniotomy and resection, which left her with a right IV, V, and VI cranial nerve palsy. As the Botox began to wear off, the right eye began to drift back in (E). Botox was reinjected into the right medial rectus muscle, and the patient underwent a full tendon transfer of the right superior and right inferior rectus muscles to the origin of the lateral rectus along with a posterior fixation suture (Foster modification), which substantially straightened her eye (F). Seven months later, she had an area of binocularity centrally, although she still had double vision when she looked down, left, or right (G,H). Fig. 5.25 (Continued) This 48-year-old patient noted some ringing in her ears, and MRI demonstrated a parasellar right cavernous meningioma. She underwent pterional craniotomy and resection, which left her with a right IV, V, and VI cranial nerve palsy. Seven months later, she had an area of binocularity centrally, although she still had double vision when she looked down, left, or right (G,H). This has remained stable (I), and when seen 30 months following her strabismus surgery, she still had an area of binocularity centrally and was pleased with her ability to function (J,K). She was noted to have some redness in the right eye due to neurotrophic changes because of the loss of normal sensation. Comment: The patient was made functional here by muscle transposition surgery. She remains at risk because of the lack of fifth nerve innervation and therefore can easily develop epithelial breakdown. It is imperative that these patients be followed carefully. Because of the proximity of both the afferent and efferent visual pathways to lesions in the parasellar region, surgical intervention, whether transsphenoidal or transcranial, carries substantial risks for exacerbating existing or causing new neuro-ophthalmic findings. It is often critical to have a baseline examination before intervention because unrecognized previous compromise related to the presence of tumors can put patients at increased risk. Medicolegally, it is also nice to know what is preexistent. As mentioned above, quantitative assessment of both the afferent and efferent visual pathways is paramount. The presence of OCT thinning or optic atrophy may indicate previous optic nerve compromise, even when central visual function and visual fields appear normal. Subtle comitant deviations, including eso-, exo-, and hyperdeviations, may be left over from previous cranial nerve palsies. Fusional amplitudes may mask these. Preexisting motility problems may also be unrecognized if the patients have poor central vision or a previous history of strabismus, amblyopia, or facultative suppression. Transsphenoidal surgery has the potential for injuring the optic nerve in its path through the lateral wall of the sphenoid bone, or the chiasm and tract in their suprasellar location. Additionally, injury to the carotid artery may produce secondary ischemic effects that may cause central peripheral visual field defects or even a chiasmal syndrome. Blood liberated into the subarachnoid space can result in secondary vasospasm and related ischemic changes. Following transsphenoidal surgery, infectious or inflammatory conditions can affect the optic nerve and chiasm. Overpacking the sphenoid sinus and sella can actually compress and elevate the optic nerves and chiasm, producing additional or new visual field defects. Later herniation of the chiasm into an empty sella may also produce delayed problems with visual exacerbation. Worsening of extraocular motility following transsphenoidal surgery usually implies involvement of the cavernous sinus. As the ocular motor nerves run lateral to the carotid artery, it is unusual to produce cavernous sinus syndrome unless there is aggressive manipulation, hemorrhage, vasospasm, or vascular compromise. It is even less likely that the orbital structures themselves may be injured during transsphenoidal surgery, although the orbit may be entered if the surgeon becomes disoriented laterally. The potential for injury of the afferent and efferent visual pathways with transcranial surgery depends on the approach. A transfrontal approach can result in injury to the optic nerves and chiasm and frequently cause problems with the olfactory nerve. A pterional approach can injure the optic nerve, particularly during a takedown of the anterior clinoid (especially if this is done extradurally). Aggressive surgery in the area of the cavernous sinus, of course, can result in compromise of the third, fourth, and sixth cranial nerves, but also the fifth nerve, producing neurotrophic problems (Fig. 5.25). Aggressive retraction of the scalp flap can injure branches of the seventh nerve, and although this usually causes brow ptosis alone, it can theoretically compromise lid closure. The burr hole placed at the pterion can be misdirected into the orbit, producing damage to the superior rectus and levator muscles (resulting in ptosis and diplopia), or to the lateral rectus (Fig. 5.24). Overly aggressive retraction of the temporal lobe during a pterional approach can produce secondary ischemia and infarction, compromising the visual pathways within the temporal lobe and resulting in homonymous hemianopsia, usually superior and incongruous, secondary to involvement of Meyer’s loop. Radiation therapy can result in delayed radiation optic neuropathy, usually appearing 6 months to 2 years following fractionated radiation therapy. This often presents with the sudden onset of altitudinal visual field loss followed by central acuity loss. Magnetic resonance imaging (MRI) findings include enhancement of the optic nerve extending to the chiasm. Radiosurgery with the gamma knife, Cyber Knife, or modified linear accelerators (LINAC) may result in acute necrosis, which can involve the optic nerve, chiasm, or optic radiations in the temporal lobe.77 Stereotactic radiosurgery that encompasses the cavernous sinus has a small but not insignificant incidence of worsening of ocular motor palsies. The neuro-ophthalmic evaluation is an essential part of the assessment of any patient suspected of having a parasellar lesion. Although there is a tendency to believe that the clinical evaluation has been replaced by imaging, it is usually the examination that leads to appropriate imaging, and it clearly is quantitative assessment in the evaluation that will determine whether or not lesions are changing (natural history), even when the imaging studies remain the same. This can be an important indicator for intervention and may also be used to follow patients after intervention to determine whether that intervention, be it medical treatment, surgery, or radiation, has been effective. The psychophysical testing of the neuro-ophthalmic evaluation is far better attuned to what our patients experience than any of the radiologic or anatomic studies that may indicate disease. As such, determining what our patients see and how their eyes move and function is a critical part at all stages in following those with parasellar pathology. Requirements: Snellen chart, good lighting, patient’s refractive correction for distance, pinhole if no refractive correction is available. If a refractionist is available, this should be used in the absence of glasses. Testing points: Test each eye separately, making sure that the opposite eye is occluded. Encourage patients to guess. Ensure adequate lighting. Watch out for the ability to read only the last letters because this may indicate a visual field defect. Use a 200 E (Fig. 5.26) to quantitate acuity less than 20/400, reporting the distance at which the patient can see the direction in which the E is pointed. Patients should be allowed to hold the E in any position they like (make sure the opposite eye is covered) to avoid visual field defects. Test patients who cannot see the 200 E at any distance for “hand motion”; determine the direction in which the tester’s hand is moving. If hand motion is not present, determine if the patient can perceive light (“light perception”) and its direction (“with projection”). Requirements: Use of a near chart (Fig. 5.7), near refractive correction (or add), measurement of distance at which the card is held. Testing points: Make sure each eye is tested separately. Make sure the patient is wearing appropriate near correction. Allow patients to hold the reading material at the distance at which they see most clearly. Measure the distance to the near chart. Record the near acuity in the point system. If no correction is available, have a plus lens (usually +2.50 to +3.50) to put in front of the patient’s eye to ensure that the patient is adequately corrected for near. Requirements: Commercially available (Ishihara AO pseudoisochromatic plates) color vision plates are designed to test for congenital red-green color blindness (8% of the male population). Subtle reduction in color vision can be determined with quantitative color testing by using the American Optical Hardy-Rand-Ritter (AO-HRR) plates, the 100 hue test, or the desaturated D-15 test. Other color tests are available. Testing points: Subtle washout of colors can be recorded as a substitute if formal color vision testing is not available by comparing colored (usually red) test objects (Fig. 5.27). Fig. 5.27 Subtle optic nerve dysfunction may be appreciated as dimming or desaturation of a red test object. This is particularly useful for comparing the two eyes and may also be used to pick up subtle visual field defects by comparing red test objects across the vertical midline. Requirements: Special contrast sensitivity testing, including the Vistech system, sinusoidal gradings, and Pelli-Robson charts. Adequate lighting and appropriate refractive correction are critical.78 Testing points: Subtle abnormalities in contrast sensitivity may be detected following previous damage to the optic nerves even when Snellen acuity is normal. Requirements: Stereo testing can be done with the Titmus test and Randot test; usually, polarized glasses are used (Fig. 5.28). Fusion and fusional amplitudes can be measured with an amblyoscope. Testing points: The presence of normal stereopsis indicates that both maculae are working well. This is a triple check on the previous Snellen vision and near vision.79 Requirements: Central scotomas in particular can be picked up on Amsler grid testing (Fig. 5.29), in which patients are given a grid and asked to look at the center dot and describe whether parts of the grid are missing. A response of distortion of the lines is particularly seen in patients with macular disease. Qualitative perimetry may include Amsler grid testing and confrontation testing (Fig. 5.28), in which fingers are counted (one, two, or five) at an equal distance from the patient, allowing the observer to compare his or her own field with the patient’s field.80 This should be done monocularly. A moving or waving target can also be used to pick up dense defects. The visual fields of patients who are confined to bed may be tested by projecting a target with a laser pointer on the ceiling. This can be presented statically (on/off) or kinetically (moving from periphery to the center). Semiquantitative testing may be obtained by using a tangent screen on which variously sized test objects are moved from nonseeing to seeing kinetically or presented statically by flipping them over. Comparison testing across the vertical midline, particularly of red test objects, may pick up subtle desaturation. This can also be used across the nasal horizontal midline. Simultaneous appearance in both hemifields can pick up subtle neglect due to higher cortical dysfunction. Quantitative visual field testing usually involves dedicated apparatus, either kinetic (Goldmann bowl perimeter) or static (Humphrey, Octopus automated static perimetry). Because of longer time and duration, static perimetry in particular is often concentrated centrally, although peripheral testing is possible when indicated. Testing points: Visual field testing is required to assess extrafoveal function. Three anatomic correlates of visual fields emphasize the importance of testing centrally across the nasal horizontal midline and across the vertical midline. Therefore, all visual field tests should be designed to detect central scotomas, field defects that respect the nasal horizontal midline, and field defects that respect the vertical midline. Visual field testing should be thought of as qualitative (looking to detect the presence of an abnormality in the visual field) versus quantitative (looking for how much of a visual field defect there is). Field testing is divided into kinetic perimetry, in which a fixed-intensity target is moved from areas of nonseeing to areas of seeing, and static, in which a fixed location is tested with an increasingly intense stimulus. Fig. 5.29 An Amsler grid is very useful for picking up subtle distortion, which may be seen with macular disease, but it may also detect early visual field abnormalities, particularly if the patient is asked to compare parts of the grid across the vertical and nasal horizontal midline. Requirements: Fixation target so that accommodation does not change (patient should be instructed to fixate on a distant target, not just a light or “my nose”). An external camera can document pupil size in varying illumination. A bright light (not enough to produce an aversive response but bright enough to ensure adequate pupillary reactivity), such as a muscle light or indirect ophthalmoscope, should be used. A pupil gauge or ruler can quantitatively measure pupil size. A neutral-density filter bar can be placed in front of the better-functioning eye to grade an afferent pupillary defect (Fig. 5.9). Testing points: Requirements: A direct ophthalmoscope (Fig. 5.11) is a very sensitive means of assessing stability of the eyes in primary and eccentric gaze.55 More quantitative analysis of eye stability, as well as spontaneous and voluntary movements, can be obtained with infrared tracking, video monitoring, EOG, or electromagnetic coil recording.56 Testing points: Requirements: Observation is usually adequate for qualitative assessment. Ocular motor recording with video, infrared tracking, EOG, or search coil technology will permit more quantitative analysis. Testing points: Requirements: Qualitative malalignment21 may be directly observed, noted by asymmetry of the corneal light reflex (Hirshberg), or identified by dissociative testing (red glass, Maddox rod; Fig. 5.12). Quantitative measurements may be made with prisms or on an amblyoscope. Dissociative tests such as the Maddox rod can be quantitated by adding prisms. The Hess screen61 and binocular single vision assessed with a Goldmann bowl can further quantitate relative movements of the two eyes. Malalignment due to restrictive pathology may be separated from paretic problems by forced duction testing (anesthetic, forceps, suction cup62,83) or measurement of intraocular pressure in eccentric gaze (pneumotonometer, Tonopen). Testing points: Ductions can be assessed qualitatively by determining whether the eye crosses midline and whether it reaches appropriate eccentric gaze. The easiest clue to an abnormality in ductions is provided by asymmetric movement of the eyes. Ductions can be further quantitated with a linear ruler measurement, prisms, or most quantitatively with a Goldmann bowl. Versions are movements of the eyes relative to each other. They can be qualitatively noticed if there is adducting or abducting delay or if one eye reaches the desired position more quickly than the opposite eye. This will also be seen as malalignment. Malalignment may be measured qualitatively by using a red glass or a Maddox rod test,57 or quantitatively by using a Hess screen61 and cross-cover testing with prisms. Maddox rod permits easy demonstration of incomitant deviations (does not distinguish between paretic and restrictive causes) and allows critical identification of the direction of maximal deviation and also of minimal deviation. Requirements: A ruler is critical for measurements of the palpebral fissures (Fig. 5.16), upper lid range (Fig. 5.17), and orbital symmetry. External photographs are very useful for documenting adnexal anatomy. A Hertel apparatus can be used to determine proptosis (Fig. 5.19). Sensation can be qualitatively evaluated with a cotton tip or quantitatively with an esthesiometer (Fig. 5.21). Sophisticated orbital evaluation can be done with an ultrasound (Fig. 5.2). Testing points: Requirements: High-tech equipment for examining the orbit and adnexal structures include a slit lamp, ultrasound, OCT unit (for both anterior and posterior segment evaluation), and fundus cameras. Testing points: Fig. 5.31 This 52-year-old man presented with the acute onset of the “worst headache of his life” and severe blurred vision. CT showed evidence of an aneurysm at the skull base (A) in the distal vertebral artery with a dissection extending into the basilar artery and subarachnoid hemorrhage. Funduscopic examination revealed evidence of bilateral preretinal, interretinal, and subretinal hemorrhage related to an acute increase in intracranial pressure (B,C). The patient’s Terson syndrome resolved over 6 months, with a return in visual acuity from the 20/400 when he was first seen to 20/30 and 20/40. Comment: Terson syndrome is vitreous hemorrhage associated with a subarachnoid hemorrhage. Most cases occur in the setting of a ruptured intracranial aneurysm. The hemorrhage may often clear spontaneously over weeks to months. Those that fail to clear may be treated with vitrectomy. Direct ophthalmoscope (light, plus lenses) Near card (can use as near accommodative target) 200 E +3.00 lens Pinhole Ruler Amsler grid Red top dilating drops (tropicamide) Maddox rod Their glasses Name of their eye doctor for old records Hertel Optokinetic nystagmus (OKN) strip or drum Neutral-density filter for quantitating an APD External camera Indirect ophthalmoscope Portable slit lamp Pressure measure (applanation tonometer, Tonopen) Titmus test Schirmer strips Stethoscope for auscultation (carotid, orbit) Slit lamp with biomicroscopic lenses Gonioprism Color tests (AO-HRR plates, D-15, 100 hue) Contrast tests (Pelli-Robson, Vistech) Automated static perimetry OCT Fluorescein angiography Visual evoked potential (latency increase suggests inflammation, not compression) Multifocal ERG (abnormality suggests maculopathy) Hess screen Goldmann bowl Ultrasound Video recording Infrared tracking Scleral search coil
Core Messages
Introduction
History
Anatomy
Afferent System
Efferent System
Adnexal Structures
Evaluation
Afferent System Evaluation
Efferent System Evaluation
Adnexal Assessment
Rehabilitation
Rehabilitation of Afferent System Dysfunction
Efferent System Rehabilitation
Adnexal Rehabilitation
Exacerbation of Ophthalmic Findings Following Surgical Intervention
Conclusion
Appendix I: The Neuro-ophthalmic Examination for the Neurosurgeon
Assessment of the Afferent System
Snellen Acuity
Near Vision
Adjunctive Macular Function Tests
Color Vision
Contrast Sensitivity
Stereo Acuity
Visual Field Testing
Assessment of the Pupil
Assessment of the Efferent System
Ocular Motor Stability
Assessment of Ocular Motor Systems
Assessment of Ocular Malalignment
Adnexal Evaluation
Basic Adnexal Evaluation
Adjunctive Adnexal Assessment
Appendix II
Basics in Your Pocket
Patient Supplies
Your Neuro-ophthalmologist
In the Clinic
High Tech
References
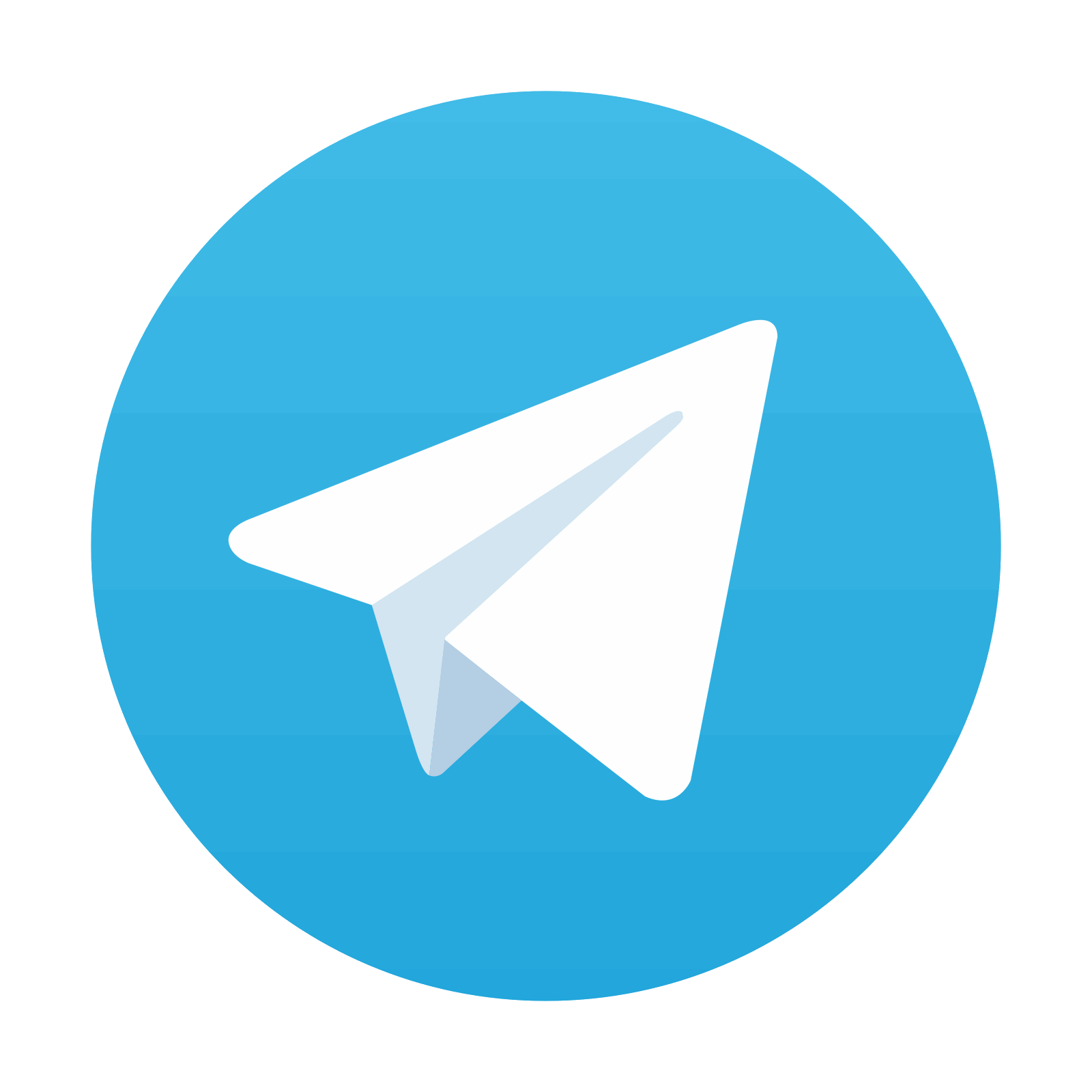
Stay updated, free articles. Join our Telegram channel

Full access? Get Clinical Tree
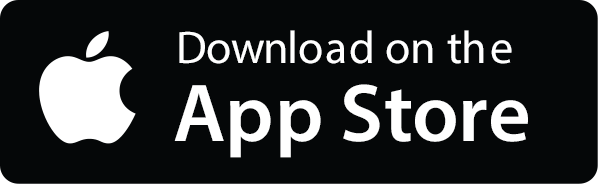
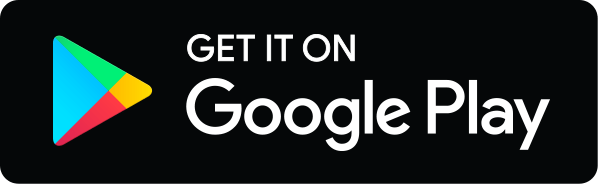