Overview of Synaptic Transmission
Synapses Are Either Electrical or Chemical
Electrical Synapses Provide Instantaneous Signal Transmission
Cells at an Electrical Synapse Are Connected by Gap-Junction Channels
Electrical Transmission Allows the Rapid and Synchronous Firing of Interconnected Cells
Chemical Synapses Can Amplify Signals
Postsynaptic Receptors Gate Ion Channels Either Directly or Indirectly
WHAT GIVES NERVE CELLS THEIR SPECIAL ABILITY to communicate with one another rapidly and with such great precision? We have already seen how signals are propagated within a neuron, from its dendrites and cell body to its axonal terminals. With this chapter we begin to consider the signaling between neurons through the process of synaptic transmission.
The specialized site at which one neuron communicates with another is called a synapse, and synaptic transmission is fundamental to the neural functions we consider later in the book, such as perception, voluntary movement, and learning.
The average neuron forms several thousand synaptic connections and receives a similar number. The Purkinje cell of the cerebellum receives up to 100,000 synaptic inputs. Although many of these connections are highly specialized, all neurons make use of one of the two basic forms of synaptic transmission: electrical or chemical. Moreover, the strength of both forms of synaptic transmission can be enhanced or diminished by cellular activity. This plasticity of synapses is crucial to memory and other higher brain functions.
Electrical synapses are employed primarily to send rapid and stereotyped depolarizing signals. In contrast, chemical synapses are capable of more variable signaling and thus can produce more complex behaviors. They can mediate either excitatory or inhibitory actions in postsynaptic cells and produce electrical changes in the postsynaptic cell that last from milliseconds to many minutes. Chemical synapses also serve to amplify neuronal signals, so even a small presynaptic nerve terminal can alter the response of large postsynaptic cells. Not surprisingly, most synapses in the brain are chemical. Because chemical synaptic transmission is so central to understanding brain and behavior, it is examined in detail in the next four chapters.
Synapses Are Either Electrical or Chemical
The term synapse was introduced at the beginning of the twentieth century by Charles Sherrington to describe the specialized zone of contact at which one neuron communicates with another. This site had first been described histologically at the level of light microscopy by Ramón y Cajal in the late 19th century.
All synapses were initially thought to operate by means of electrical transmission. In the 1920s, however, Otto Loewi discovered that the chemical compound acetylcholine (ACh) conveys signals from the vagus nerve to the heart. Loewi’s discovery provoked considerable debate in the 1930s over whether chemical signaling existed at other synapses, including synapses between motor nerve and skeletal muscle as well as synapses in the brain.
Two schools of thought emerged, one physiological and the other pharmacological. Each championed a single mechanism for all synaptic transmission. Led by John Eccles (Sherrington’s student), the physiologists argued that synaptic transmission is electrical, that the action potential in the presynaptic neuron generates a current that flows passively into the postsynaptic cell. The pharmacologists, led by Henry Dale, argued that transmission is chemical, that the action potential in the presynaptic neuron leads to the release of a chemical substance that in turn initiates current in the postsynaptic cell. When physiological and ultrastructural techniques improved in the 1950s and 1960s, it became clear that both forms of transmission exist. Although a chemical transmitter is used at most synapses, some operate purely by electrical means.
Once the fine structure of synapses was made visible with the electron microscope, chemical and electrical synapses were found to have different structures. At chemical synapses the presynaptic and postsynaptic neurons are completely separated by a small space, called the synaptic cleft; there is no continuity between the cytoplasm of one cell and the next. In contrast, at electrical synapses the pre- and postsynaptic cells communicate through special channels, the gap-junction channels, that directly connect the cytoplasm of the two cells.
The main functional properties of the two types of synapses are summarized in Table 8–1. The most important difference can be observed by injecting a positive current into the presynaptic cell to elicit a depolarization. At both types of synapses outward current across the presynaptic cell membrane deposits positive charge on the inside of the presynaptic cell membrane, thereby depolarizing the cell (see Chapter 6). At electrical synapses some of the current will enter the postsynaptic cell through the gap-junction channels, depositing a positive charge on the inside of the membrane and depolarizing it. The current leaves the postsynaptic cell across the membrane capacitance and through resting channels (Figure 8–1A). If the depolarization exceeds threshold, voltage-gated ion channels in the postsynaptic cell open and generate an action potential. By contrast, at chemical synapses there is no direct low-resistance pathway between the pre- and postsynaptic cells. Instead, the action potential in the presynaptic neuron initiates the release of a chemical transmitter, which diffuses across the synaptic cleft to interact with receptors on the membrane of the postsynaptic cell (Figure 8-1B).
Table 8–1 Distinguishing Properties of Electrical and Chemical Synapses
Figure 8-1 Functional properties of electrical and chemical synapses.
A. At an electrical synapse some current injected into the presynaptic cell escapes through resting (nongated) ion channels in the cell membrane. However, some current also enters the postsynaptic cell through gap-junction channels that connect the cytoplasm of the pre- and postsynaptic cells and that provide a low-resistance (high-conductance) pathway for electrical current.
B. At chemical synapses all current injected into the presynaptic cell escapes into the extracellular fluid. However, the resulting depolarization of the presynaptic cell membrane can produce an action potential that causes the release of neurotransmitter molecules that bind receptors on the postsynaptic cell. This binding opens ion channels that initiate a change in membrane potential in the postsynaptic cell.
Electrical Synapses Provide Instantaneous Signal Transmission
During excitatory synaptic transmission at an electrical synapse, voltage-gated ion channels in the presynaptic cell generate the current that depolarizes the postsynaptic cell. Thus these channels not only depolarize the presynaptic cell above the threshold for an action potential but also generate sufficient ionic current to produce a change in potential in the postsynaptic cell.
To generate such a large current, the presynaptic terminal must be big enough for its membrane to contain many ion channels. At the same time, the postsynaptic cell must be relatively small. This is because a small cell has a higher input resistance (Rin) than a large cell and, according to Ohm’s law , undergoes a greater voltage change (ΔV) in response to a given presynaptic current (I).
Electrical synaptic transmission was first described by Edwin Furshpan and David Potter in the giant motor synapse of the crayfish, where the presynaptic fiber is much larger than the postsynaptic fiber (Figure 8–2A). An action potential generated in the presynaptic fiber produces a depolarizing postsynaptic potential that often exceeds the threshold to fire an action potential. At electrical synapses, the synaptic delay—the time between the presynaptic spike and the postsynaptic potential—is remarkably short (Figure 8-2B).
Figure 8-2 Electrical synaptic transmission was first demonstrated at the giant motor synapse in the crayfish. (Adapted, with permission, from Furshpan and Potter 1957 and 1959.)
A. The lateral giant fiber running down the nerve cord is the presynaptic neuron. The giant motor fiber, which projects from the cell body in the ganglion to the periphery, is the postsynaptic neuron. Electrodes for passing current and for recording voltage are placed within the pre- and postsynaptic cells.
B. Transmission at an electrical synapse is virtually instantaneous—the postsynaptic response follows presynaptic stimulation in a fraction of a millisecond. The dashed line shows how the responses of the two cells correspond in time. At chemical synapses there is a delay (the synaptic delay) between the pre- and postsynaptic potentials (see Figure 8-8).
Such a short latency is not possible with chemical transmission, which requires several biochemical steps: release of a transmitter from the presynaptic neuron, diffusion of transmitter molecules to the postsynaptic cell, binding of transmitter to a specific receptor, and subsequent gating of ion channels (all described later in this chapter). Only current passing directly from one cell to another can produce the near-instantaneous transmission observed at the giant motor synapse.
Another feature of electrical transmission is that the change in potential of the postsynaptic cell is directly related to the size and shape of the change in potential of the presynaptic cell. Even when a weak subthreshold depolarizing current is injected into the presynaptic neuron, some current enters the postsynaptic cell and depolarizes it (Figure 8–3). In contrast, at a chemical synapse the current in the presynaptic cell must reach the threshold for an action potential before it can release transmitter and elicit a response in the postsynaptic cell.
Figure 8-3 Electrical transmission is graded and occurs even when the current in the presynaptic cell is below the threshold for an action potential. This can be demonstrated by depolarizing the presynaptic cell with a small current pulse through one electrode while the membrane potential is recorded with a second electrode. A subthreshold depolarizing stimulus causes a passive depolarization in the presynaptic and postsynaptic cells. (Depolarizing or outward current is indicated by an upward deflection.)
Most electrical synapses can transmit both depolarizing and hyperpolarizing currents. A presynaptic action potential with a large hyperpolarizing afterpotential produces a biphasic (depolarizing-hyperpolarizing) change in potential in the postsynaptic cell. Signal transmission at electrical synapses is similar to the passive propagation of subthreshold electrical signals along axons (see Chapter 6) and therefore is also referred to as electrotonic transmission. At some specialized gap junctions the channels have voltage-dependent gates that permit them to conduct depolarizing current in only one direction, from the presynaptic cell to the postsynaptic cell. These junctions are called rectifying synapses. (The crayfish giant motor synapse is an example.)
Cells at an Electrical Synapse Are Connected by Gap-Junction Channels
The specialized region of contact between two neurons at an electrical synapse is termed the gap junction. Here the separation between the two neurons (4 nm) is much less than the normal nonsynaptic space between neurons (20 nm). This narrow gap is bridged by the gap-junction channels, specialized protein structures that conduct ionic current from the presynaptic to the postsynaptic cell.
A gap-junction channel consists of a pair of hemichannels, or connexons, one in the presynaptic and the other in the postsynaptic cell membrane. These hemichannels thus form a continuous bridge that provides a direct communication path between the two cells (Figure 8–4). The pore of the channel has a large diameter of approximately 1.5 nm, which permits inorganic ions and small organic molecules and experimental markers such as fluorescent dyes to pass between the two cells.
Figure 8-4 A three-dimensional model of the gap-junction channel, based on X-ray and electron diffraction studies.
A. The electrical synapse, or gap junction, is composed of numerous specialized channels that span the membranes of two neurons. These gap-junction channels allow current to pass directly from one cell to the other. The array of channels shown in the electron micrograph was isolated from the membrane of a rat liver. The tissue has been negatively stained, a technique that darkens the area around the channels and in the pores. Each channel appears hexagonal in outline. Magnification × 307,800. (Reproduced, with permission, from N. Gilula.)
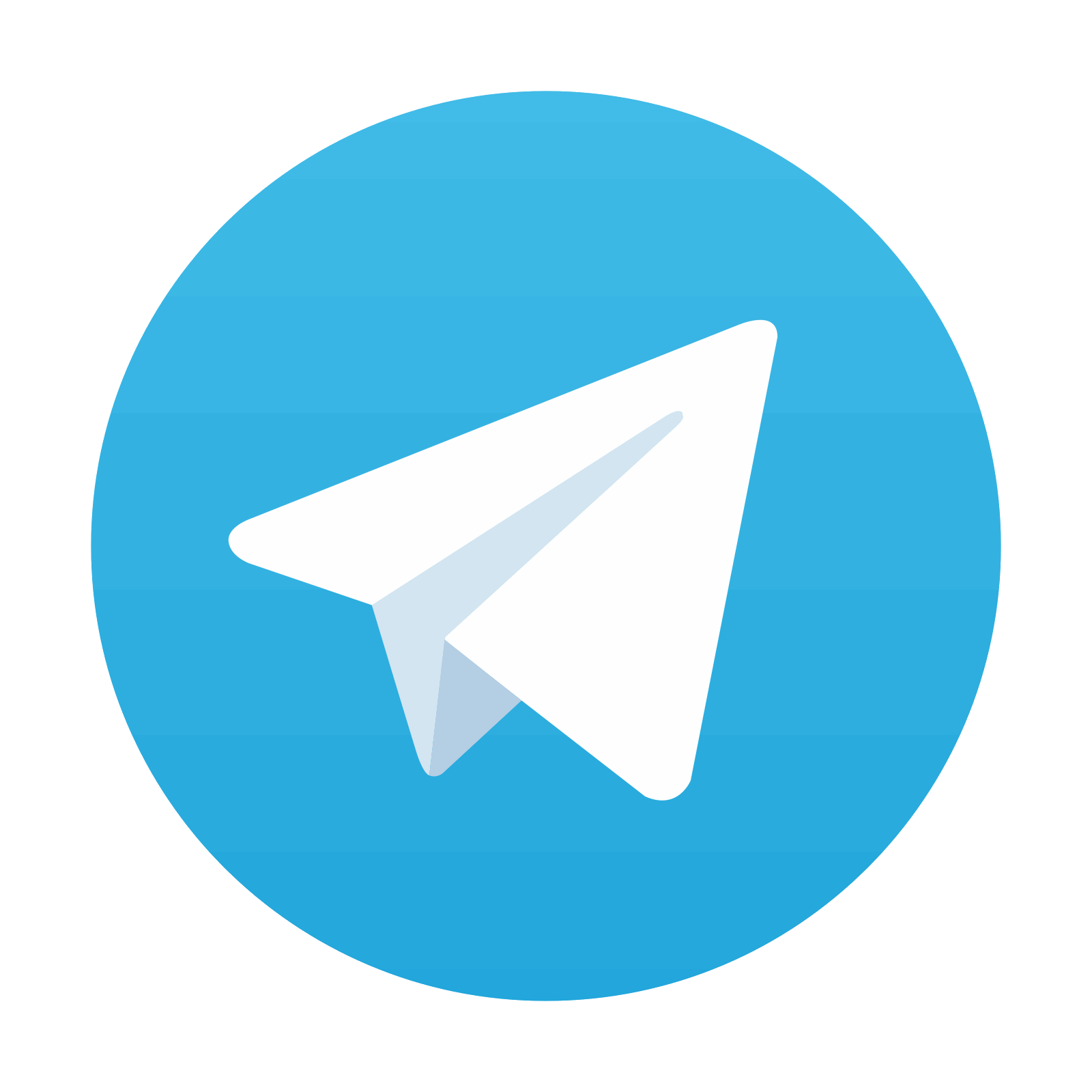
Stay updated, free articles. Join our Telegram channel

Full access? Get Clinical Tree
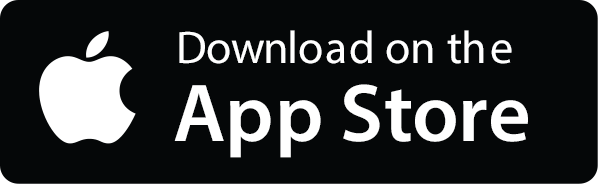
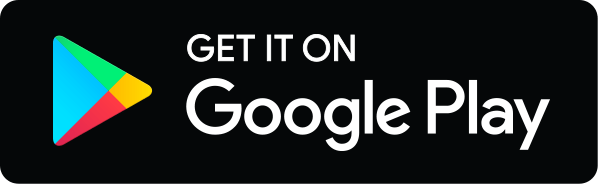