Nociception is the form of somatic sensation that detects noxious, potentially tissue-damaging stimuli. Pain has both a localizing somatic sensory component and an aversive emotional and motivational component.
Pain begins with peripheral nociceptors, which have their cell bodies in dorsal root ganglia (DRG) and in the trigeminal ganglia in the head; these neurons synapse, respectively, in the dorsal horn of the spinal cord or the trigeminal nucleus, the medullary extension of the dorsal horn.
Peripheral nociceptors express a set of channels, most notably transient receptor potential (TRP) channels, that are sensitive to noxious mechanical, thermal, and chemical stimuli. These neurons also express receptors for inflammatory mediators and substances released by damaged cells.
Primary nociceptors release a large number of neuropeptide and nonpeptide neurotransmitters in the dorsal horn and trigeminal nucleus. These regions are important sites of integration for both ascending nociceptive information and descending antinociceptive (endogenous analgesic) influences.
Sensitization is a clinically significant process in which nociceptors in an area extending beyond a tissue injury exhibit decreased thresholds for activation. Sensitization can be initiated by inflammatory mediators such as prostaglandins and leukotrienes.
Damage to neurons in nociceptive pathways can lead to severe chronic pain syndromes, termed neuropathic pain.
Plasticity within the dorsal horn and trigeminal nucleus, termed central sensitization, may be key in the initiation of chronic pain syndromes by increasing the excitability of neurons in nociceptive pathways. Numerous mechanisms have been implicated in central sensitization, including plasticity mediated by NMDA glutamate receptors.
Opiate drugs selectively suppress nociception, but not other sensory modalities, by binding to endogenous opioid receptors in descending analgesic pathways. They are the most potent analgesic compounds known, but their chronic use is limited by tolerance to their analgesic effects and by risk for addiction.
Nonsteroidal anti-inflammatory drugs (NSAIDs), which are more weakly analgesic compared with opiates, act by blocking prostaglandin-mediated sensitization, specifically by inhibiting the enzyme cyclooxygenase that is required for the synthesis of prostaglandins from arachidonic acid.
Several other medications are used to treat chronic pain syndromes, including combined serotonin–norepinephrine reuptake inhibitor (SNRI) antidepressants and compounds (eg, gabapentin, pregabalin) that inhibit certain voltage-gated Ca2+ channels.
Chronic pain is a significant public health problem associated with severe patient suffering and disability and, in some cases, drug abuse. Pain begins, however, as an adaptive response to actual or potential harm to the body with the stimulation of peripheral nociceptors, neurons specialized to detect noxious stimuli. Pain is not simply a modality of somatic sensation such as touch or vibration sense, however. Pain also has physiologic, behavioral, and aversive emotional components. Without its alerting and aversive components, its activation of withdrawal reflexes and avoidance behaviors, or its capacity to inscribe powerful emotional memories, pain would not reliably trigger escape from danger and avoidance of future harm 11–1. When it becomes chronic, however, pain loses its adaptive role as a defender of the body’s tissues and instead becomes a pressing medical problem. The aversive emotions associated with pain no longer serve a protective function, but rather produce suffering and, all too often, maladaptive behaviors. Chronic pain may result from continuing tissue damage, as can occur with cancer, but much chronic pain results from chronic inflammatory processes or damage to the pain pathways themselves (eg, by trauma, nerve compression, diabetes, or herpes varicella-zoster infection), in which case it is termed neuropathic pain. Neuropathic pain continues long after tissues have healed, and thus becomes a persistent false alarm.
11–1 Congenital Inability to Perceive Pain
Three consanguineous families from northern Pakistan were found to contain individuals who had never experienced pain. These individuals possess morphologically normal nociceptive neurons and intact somatic sensation aside from nociception, and exhibit normal intelligence. They understood something about pain (and the dangers it portends) by observing others, but were simply incapable of experiencing it themselves. What they were lacking, as a result of homozygosity for a loss-of-function mutation in the SCN9A gene, was a functional protein to form the α subunit of a particular voltage-gated Na+ channel, known as NaV1.7 (Chapter 2), which is highly expressed in nociceptive neurons. This is a remarkable finding because it demonstrates that in humans—the same is not true in the mouse—a single protein plays an absolutely essential role in the function of otherwise normal-looking peripheral nociceptors. Because the affected individuals are otherwise healthy, the finding also supports the development of selective NaV1.7 antagonists as new analgesic medications.
These families also illustrate the protective role of pain. All affected members had significant injuries to their lips and tongues from biting themselves. All had frequent bruises and cuts and most had limb fractures, often diagnosed as a result of a limp or inability to use a limb. The index case for the study, a 10-year-old child, worked as a street performer. He placed knives through his arms and walked on hot coals—and died before his 14th birthday from jumping off a roof.
Other families, with a form of hereditary sensory and autonomic neuropathy, lack nociceptive neurons because of a mutation in the TrkA receptor (the receptor for nerve growth factor [NGF]; Chapter 8). TrkA is expressed in all normally developing peripheral nociceptors, and is required for their survival. Similar to individuals with mutations in SCN9A, people lacking TrkA have injuries to lips and tongue and to limbs. The latter are reported to include joint damage and loss of finger tips. Even though individuals who do not feel pain learn second hand to be aware of injury risks, they cannot overcome the loss of pain, which while troublesome is ultimately a protective form of experience. Findings such as these have prompted interest in TrkA antagonists or NGF neutralizing antibodies as novel analgesics.
In sum, pain has both a discriminative sensory aspect and an emotional and motivational aspect. Because of its discriminative sensory component, a person can describe where the pain seems to arise (although, as will be discussed, pain from viscera can seem to come from the body surface) and can delineate its characteristics (eg, sharp, pricking, aching, burning). Because of its emotional and motivational component, pain interrupts ongoing behaviors and demands attention; it also powerfully motivates learning and thereby suppresses behaviors that put an organism in harm’s way. The emotional and motivational component gives pain its survival value, but also can produce suffering and disability when pain is chronic.
Pain begins with primary afferent nociceptors that have cell bodies in dorsal root ganglia (DRG) and that make synapses in the dorsal horn of the spinal cord 11–1. Primary nociceptors in the face and head have their cell bodies in trigeminal ganglia and synapse in the brainstem continuation of the dorsal horn, called the trigeminal nucleus. Throughout this discussion we will use the terms DRG and dorsal horn with the understanding that the same principles apply to their rostral extensions in the head.
11–1
Nociceptive pathways. A primary afferent (or first-order) nociceptive neuron synapses in the superficial layers of the dorsal horn. Morphologically, these are bipolar neurons with cell bodies in the dorsal root ganglion (or in the head, the trigeminal ganglion) and bifurcated axons that project both to the periphery and to the spinal cord (or in the head to the trigeminal nucleus of the brainstem). Axons of dorsal horn (or second-order) nociceptive neurons cross the midline and ascend in the spinoreticular or spinothalamic tracts (right). The spinothalamic tract comprises the neospinothalamic and paleospinothalamic tracts. In the neospinothalamic tract, second-order neurons synapse in the ventroposterolateral (VPL) nucleus of the thalamus, and third-order neurons project to somatosensory regions of the cerebral cortex. In the paleospinothalamic tract, second-order neurons synapse in the intralaminar thalamic nuclei and project to association cortex and limbic structures. Descending analgesic systems believed to originate with cortical neurons and neurons of the amygdala (left) activate descending analgesic systems by activating neurons in periaqueductal gray area (PAG) of the brainstem. A projection from the PAG to the rostral ventral medulla that descends to the dorsal horn mediates opiate-dependent descending analgesia.

The peripheral terminals of primary afferent nociceptors are morphologically undifferentiated free nerve endings. However, these nerve endings express a panoply of receptors and channels 11–2. They express a large number of G protein–coupled receptors, including bradykinin B2, eicosanoid, purine nucleoside P2Y, histamine, serotonin 5HT1 and 5HT2A, γ-aminobutyric acid GABAB, α2-adrenergic, somatostatin, neuropeptide Y, and μ, δ, and κ opioid receptors. They also express ligand-gated channels, such as the ATP-gated P2X3 receptor, and a subset of mature nociceptors express TrkA, the protein tyrosine kinase that binds nerve growth factor (NGF). (All developing nociceptors express TrkA and are dependent on NGF; see 11–1.) In addition, nociceptive terminals express diverse channels involved in transducing noxious signals, including transient receptor potential (TRP) channels 11–1; 11–2, heat-sensitive K+ channels, and acid-sensing ion channels (ASICs). These various types of ion channels can be gated directly by noxious heat, cold, mechanical stimulation, acid, or irritant chemicals. Alternatively, TRP channels may be coupled to activation of G protein–coupled receptors, as appears to be the case for the bradykinin B2 receptor 11–3.
11–2
Receptors and channels on the peripheral terminals of primary nociceptors. The detail is from a section of a hypothetical cutaneous free nerve ending. It shows a transient receptor potential (TRP) channel, the TRPV1 channel, that is gated by capsaicin and heat, an acid-sensing ion channel (ASIC), and a voltage-gated Na+ channel that might be activated by the generator potentials caused by stimulating the TRP and ASIC channels. Voltage-gated K+ and Ca2+ channels (not shown) would also be expressed. Many G protein–coupled receptors might be expressed as described in the text. Shown are the bradykinin B2 and P2Y ATP receptors, which signal primarily via Gq; the μ (or other) opioid receptors, which signal via Gi/o; and the prostaglandin PGE2 EP receptors, different subtypes of which signal via Gi/o or other G proteins. Another receptor family, the tyrosine receptor kinases, is represented by TrkA, the nerve growth factor (NGF) receptor.

Channel | Family | Exemplary Stimuli1 | Location |
---|---|---|---|
TRPV1 | TRPV |
| PN3 |
TRPV2 | TRPV |
| PN |
TRPV3 | TRPV |
| PN, tongue |
TRPV4 | TRPV |
| PN |
TRPM8 | TRPM5 |
| PN |
TRPA1 | TRPA |
| PN |
11–2 The Capsaicin Receptor and TRP Channels
Capsaicin, the compound that gives chili peppers (capsicums) their heat, has turned out to be a powerful experimental tool for the study of pain. Many individuals who have touched their eyes after cutting chili peppers can attest to the intense burning pain that capsaicin can produce. The discovery of the capsaicin receptor, first described as the vanilloid receptor (VR1) for the family of pungent chemicals that includes capsaicin, opened an important door in the study of nociception. VR1 was the first of a large family of channels that are the key detectors of noxious stimuli on peripheral nociceptors.
VR1 turned out to be structurally homologous to transient receptor potential (TRP) channels, already known from Drosophila photoreceptors; thus, VR1 is now denoted as TRPV1. Across phylogeny TRP channels serve as major sensory transducers, with diverse ligands and gating mechanisms. In humans, they are gated not only by noxious thermal, chemical, and mechanical stimuli but also by nonnoxious heat and cold. As well, TRP receptors transduce sweet, bitter, and umami tastes in humans and other animals, and detect pheromones in the mouse.
TRP channels are formed in different cell types by a large number of different but related proteins of which there are six mammalian subfamilies based on amino acid homology. TRP channels are composed of six transmembrane subunits that assemble into tetramers with a central pore that forms a nonselective cation channel. In general, channel opening will admit Na+ and Ca2+, thus depolarizing cells. Gating of TRP channels is complex 11–1; different channels can be activated by changes in ambient temperature (eg, TRPV1,2,3,8), by mechanical stimuli, by exogenous small molecules (eg, capsaicin), by endogenous lipids (eg, arachidonic acid metabolites, anandamide, diacylglycerol), and perhaps by ions such as Ca2+ and Mg2+. Knockout experiments demonstrate clearly that response to a single stimulus, for example, a particular temperature, does not map to a single channel. Moreover, there is growing evidence that TRP channel subunits form heteromultimers, which generates an even larger number of functional channels.
In addition to direct gating by exogenous stimuli, TRP channels can be gated or modulated by G protein–coupled receptor-activated second messengers, such as phosphatidylinositol (4,5)-bisphosphate (PIP2) and diacylglycerol (DAG), by protein phosphorylation, and by lipid signals such as anandamide (an endocannabinoid; Chapter 8). The precise TRP channels expressed in a cell determine, to a great extent, the ability of that cell to sense important features of the environment. Mutations in several TRP channel subunits have been established as the cause for specific diseases (Chapter 2).
11–3 Bradykinin
Bradykinin, a nine-amino-acid peptide, is one of the most potent pronociceptive, or algesic, substances known. It is capable of eliciting intense pain and burning sensations when administered to humans. The term bradykinin (brady, slow; kinin, movement) refers to the substance’s ability to produce a slow-onset contraction of smooth muscle in the gut in vitro.
Bradykinin and kallidin are products of the kininogen propeptides (resulting from two splice variants) released by the enzymatic action of kallikreins, which are primarily concentrated in plasma but also can be found in smaller quantities in tissues. Kallikreins are inactive in their basal state; they become activated when they are cleaved by factor VII, a protease that also plays a role in blood clotting cascades. Factor VII is activated in response to contact with collagen and other negatively charged substances exposed during tissue injury. After they are formed, bradykinin and kallidin promote pain and inflammation; thus, factor VII is primarily responsible for the coordinated response of blood clotting, pain, and inflammation that occurs after tissue is damaged.
Bradykinin acts via two G protein–coupled receptors, termed B1 and B2. B2 receptors are highly expressed by primary nociceptors, and are important in acute pain and inflammation; B1 receptors may contribute more to chronic pain and inflammation. Surprisingly, mice lacking TRPA1 or TRPV1 channels have a markedly diminished nociceptive response to bradykinin. It appears that the B2 receptor is coupled to these channels via activation of phospholipase Cβ (PLCβ), which is activated by Gq-linked receptors (Chapter 4). This observation raises the interesting possibility that other neurotransmitter receptors expressed in nociceptors, such as 5HT2A and P2Y receptors, which are also Gq linked and activate PLCβ, may stimulate TRPA1 and TRPV1. Additionally, it suggests that TRP channels may serve as effectors of G protein–coupled receptor activation.
Many of the TRP channels transduce environmental stimuli such as temperature and exogenous chemicals. Many of the G protein–coupled receptors and ligand-gated channels detect tissue damage that causes, for example, release of ATP and of K+ and H+ ions from damaged cells. In the vicinity of an injury, serotonin is released from platelets, and histamine from tissue mast cells. Bradykinin, one of the most potent activators of nociception, is produced from plasma kininogen by the actions of kallikreins, enzymes that are activated rapidly at the site of an injury 11–3 (see 11–5). However, the interactions of G protein–coupled receptors and of TRP and other channels are complex and an important avenue for research on pain and analgesia.
Because nociceptors are specialized to respond only to noxious stimuli, the channels that transduce environmental signals have high thresholds for activations—in marked contrast to the transducers for light touch 11–3. When activated, noxious stimulus-detecting channels produce inward (depolarizing) currents within the peripheral terminals of primary nociceptors that can initiate trains of action potentials that are then transmitted to the dorsal horn of the spinal cord. Nociceptive neurons of DRG represent “first-order” neurons that synapse on “second-order” neurons within the dorsal horn of the spinal cord (see 11–1). These second-order neurons may be local interneurons or projection neurons that carry nociceptive information to the brainstem and thalamus.
11–3
Physiologic recordings from two cutaneous primary sensory neurons. The nociceptor (left) has a higher threshold, but exhibits an increasing firing rate once within the noxious range. The thermoreceptor, which detects nonnoxious heat, has a low threshold and exhibits no change in firing rate as the stimulus intensity increases. This is revealed by action potentials elicited from the two neurons as a function of stimulus intensity (which increases from top to bottom); 45°C is the threshold for tissue damage.

Primary nociceptive neurons must convey signals that distinguish between innocuous and noxious stimuli. Nociceptors accomplish this in one of two ways. They either possess high thresholds for stimulation as noted above or possess the capability of coding the intensity of a stimulus in the frequency of impulses relayed centrally, so that noxious stimuli produce the highest firing rates (see 11–3). Aδ nociceptors, or high-threshold mechanoreceptors, respond to noxious mechanical stimuli, especially sharp objects; approximately half of the mechanothermal nociceptors also respond to noxious thermal stimuli. The majority of C fibers in cutaneous nerves, which are unmyelinated, have higher thresholds than those of myelinated axons, and respond nonselectively to noxious mechanical, thermal, and chemical stimuli. They are therefore known as polymodal nociceptors. Activation of C fibers is mediated by a wide range of endogenous chemicals that are released in response to damaged tissue or inflammation.
Finally, not all receptors and channels expressed on primary nociceptors transmit pain signals. Cannabinoid CB1 and μ, δ, and κ opioid receptors expressed on nociceptors have been demonstrated to mediate analgesia. Indeed, evidence from genetically engineered mice, with CB1 receptors deleted only in primary nociceptors, suggests that peripheral CB1 receptors play a major role in cannabinoid-mediated analgesia. This might permit development of cannabinoid analgesics that do not cross the blood–brain barrier and are thus free of cognitive and psychotropic effects (Chapter 16). Local analgesics and anesthetics take advantage of the presence of opioid receptors and Na+ channels, respectively, on the nociceptor terminals to provide temporary relief to specific body regions (see below). Typical routes of administration include topical application or injection in the vicinity of peripheral nerve endings or major nerve trunks.
Peripheral nerves contain several different types of axons that carry sensory information; the greater the diameter and thickness of myelination of the axons, the greater their conduction velocity. The axons of cutaneous afferent nerves belong to one of three main classes. The largest and most heavily myelinated axons that originate from the skin are called Aβ fibers. Aα axons, which are thicker, innervate muscles and joints; these axons are involved in proprioception, the transmission of sensory information related to posture, motion, and position. Aβ fibers include cutaneous mechanoreceptors, which are free nerve endings that respond to light touch and the bending of hairs. Primary nociceptive neurons have small-caliber axons, some of which are thinly myelinated Aδ axons and most of which are unmyelinated C fibers. Given their lack of myelination, C fibers conduct impulses very slowly (<2 m/s) compared with Aδ fibers (approximately 20 m/s) 11–2; 11–4.
11–4 First and Second Pain
When a person forcefully stubs a toe or otherwise hurts a distal extremity, he or she experiences two distinct sensations. The first sensation arrives swiftly and is sharp and brief; the second sensation, which arrives after a brief delay, typically burns, is more unpleasant, and tends to be more prolonged. The first sensation, or first pain, is caused by more rapidly conducting Aδ fibers, whereas the more aversive second pain is caused by slowly conducting C fibers.
Differences in the timing of first and second pain are directly related to the conduction velocities of Aδ and C fibers. Imagine accidentally placing a finger tip into a cup of scalding coffee. If the distance from finger tip to spinal cord were 1 m, an Aδ fiber conducting at 20 m/s would deliver the message to the CNS in 0.05 seconds. A C fiber conducting at 0.5 m/s would require 2 seconds to deliver the same message. Under such circumstances, the response of Aδ fibers leads to a rapid spinal reflex that causes the finger to be withdrawn and thus rescued from further harm. In contrast, the response of C fibers is slow enough that, by itself, would have permitted serious tissue damage to occur.
These two types of fibers can be separated in other ways summarized in 11–2 with a view to better understanding pain. Aδ fibers are relatively sensitive to pressure but are relatively insensitive to low doses of local anesthetics. Conversely, C fibers are more sensitive to local anesthetics. Because of such differences, it is possible to selectively suppress the firing of Aδ fibers, for example, by inflating a blood pressure cuff around a limb, and the firing of C fibers, for example, by an appropriate injection of local anesthetic. The blocking of Aδ fibers by such means suppresses the sharp first pain that occurs in response to an acute noxious stimulus, but, interestingly, that may cause the delayed second pain to be stronger and more aversive. These findings suggest that Aδ fiber stimulation may reduce C fiber–mediated nociception. This may be the reason why slapping or rubbing a patch of skin prior to receiving an injection may decrease the overall aversiveness of the experience.
Aδ Fibers | C Fibers | |
---|---|---|
Diameter | 2–5 μm | 0.2–1.5 μm |
Conduction velocity | 6–30 m/s | 0.5–2 m/s |
Myelination | Thinly myelinated | Unmyelinated |
Time to conduct 1 m | Approximately 0.05 s | Approximately 2.0 s |
Responsiveness to stimuli | High-threshold mechanoreceptors, mechanothermal receptors | Polymodal |
Blocked by | Pressure | Low doses of local anesthetics |
At sites of tissue damage, diffusable mediators, including arachidonic acid metabolites such as prostaglandins and leukotrienes, cytokines such as interleukin-1 (IL-1), chemokines, and growth factors such as NGF, induce a form of neural plasticity within nociceptors called sensitization. The area of sensitized nociceptors usually extends beyond the borders of tissue damage 11–4. Some mediators such as bradykinin 11–3 can both activate and sensitize nociceptors. Typically, sensitization decreases the thresholds for activation of nociceptors. Thus, previously innocuous stimuli, such as light touch, now activate nociceptors and are experienced as painful; this is termed allodynia. Moreover, the response to noxious stimuli is exaggerated, referred to as hyperalgesia. At its most extreme, sensitization might produce pain in the absence of a stimulus (ie, spontaneous pain). Sensitization of C fibers can be measured in humans using the technique of microneurography. Possible mechanisms of sensitization include increased expression and distribution of nonselective cation channels, ectopic nerve activity, and second messenger–dependent phosphorylation of TRP channels and Na+ channels in pain pathway neurons in a manner that alters their thresholds for opening.
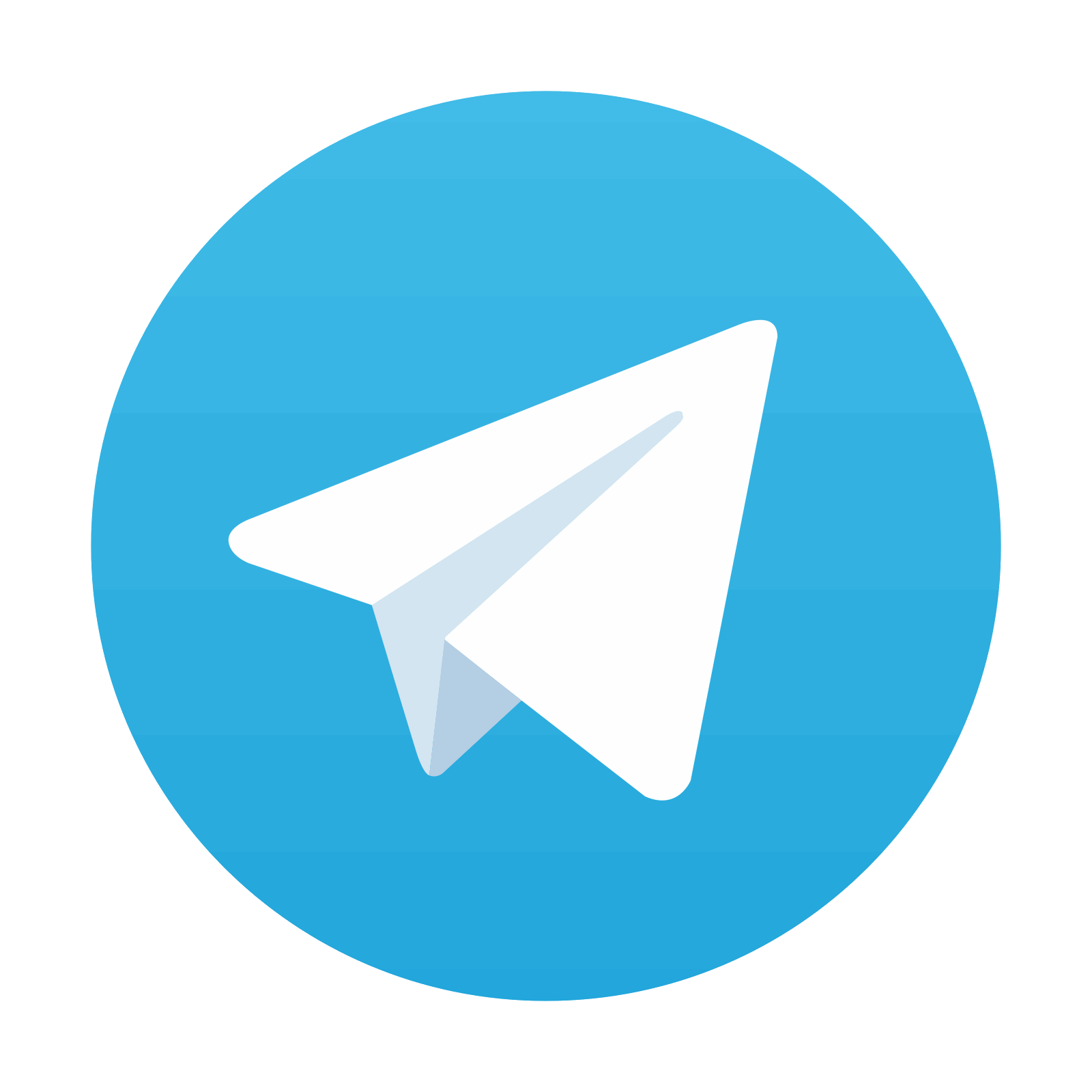
Stay updated, free articles. Join our Telegram channel

Full access? Get Clinical Tree
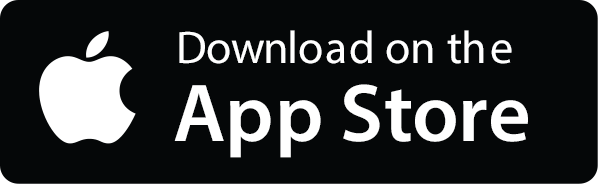
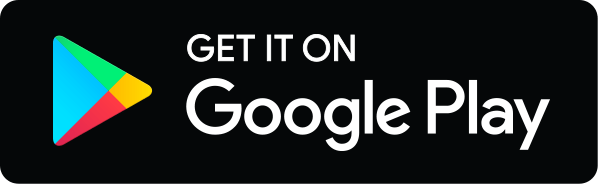