Pain and Neural Dysfunction in Cervical Degenerative Disease
Eeric Truumees
With age, the cervical spine degenerates. In most, this degeneration is asymptomatic. In those with symptoms referable to the neck, most report transient pain likely originating in the myofascial structures (1,2).
Degenerative changes tend to progress with age and may directly affect some or all levels of the cervical spine. Common findings include intervertebral disk height loss and uncovertebral joint, facet joint, and end plate osteophyte formation. The longitudinal ligaments and ligamentum flavum calcify, overgrow, or fold into the canal. These changes may decrease or increase segmental motion.
This chapter focuses on structural changes in the cervical spine and their associated, potentially debilitating, symptoms. Those symptoms are frequently classified into axial pain syndromes, radicular symptoms, and myelopathy. Often, these symptoms occur together.
CERVICAL DEGENERATIVE DISEASE SYNDROMES AND THEIR INCIDENCE
A best evidence synthesis of the literature from 1980 to 2006 assayed the determinants of neck pain and its socioeconomic burden (3). The authors identified 469 studies and found 249 scientifically admissible. Of those, 101 articles reported on the burden and determinants of neck pain. The incidence of neck pain was 213 per 1,000 persons. The 12-month prevalence ranged between 30% and 50%. Activity-limiting pain ranged from 1.7% to 11.5%. Neck pain was more prevalent among women and peaked in middle age.
Cervical axial degenerative disease often presents with a decreased range of motion or referred somatic pain. At any one time, 5% of the population is disabled from neck pain (4). Nonmodifiable risk factors include female gender, middle age, and genetics. Modifiable risk factors include poor psychological health and tobacco exposure.
Symptomatic cervical spondylosis represents 2% of all US hospital admissions (5). Mechanical neck pain arising from spinal arthritis is much more common than neurologic dysfunction. Radiculopathy from disk herniation was reported in 0.055 cases per 1,000 person years. Radicular symptoms may result from chemical mediators from the disrupted disk, vascular embarrassment, or nerve root compression from disk protrusions or osteophytes. These radicular symptoms include pain (i.e., radiculitis) or nerve root dysfunction (radiculopathy). In younger patients, symptoms tend to arise acutely from disk protrusion. In older patients, more gradual symptom onset stems from spondylotic narrowing of the foramina or central canal.
Compression of the spinal cord, or its vascular supply, may cause cord dysfunction and myelopathy, often leading to upper motor neuron findings such as spastic weakness and ataxia. The developmental size of the spinal canal and host metabolic factors affect the likelihood of symptom progression (6).
Understanding the pathophysiologic basis for these symptoms is critical in diagnosis, in treatment, and in counseling the patient as to the likelihood of improvement. For example, given that many radicular complaints resolve spontaneously, how long can a patient be treated expectantly before structural changes (i.e., nerve damage) occur? Further, given that most of the morphologic stigmata of degenerative disease are indistinguishable from the universal effects of aging, when do these findings in fact represent a disease state (7)?
The four cardinal attributes that define a disease (8) are as follows:
Cause (etiology)
Mechanism of development (pathogenesis)
Structural alterations induced in cells and tissues (morphology)
Functional consequences observed clinically (symptoms)
For our purposes, a disease process is identified when debilitating symptoms arise.
PAIN AS A SYMPTOM
Most spine patients present to their physician because of pain. With spondylotic etiologies in particular, our understanding of the precipitants of pain remains limited. For example, why do degenerative changes present for decades
suddenly worsen? Why is the degree of spondylosis only marginally related to the severity of the pain itself?
suddenly worsen? Why is the degree of spondylosis only marginally related to the severity of the pain itself?
Certainly, the relationship between the patient’s clinical complaint and his or her underlying radiographic degenerative change is affected by the subjective aspects of the pain experience. A better understanding of the pathway between the objective, anatomic insult, and the patient’s symptoms allows the physician to treat the whole individual and not simply an anatomic irregularity. The irregularity may have been present for decades and certainly exists in an asymptomatic form in large portions of the population.
Biologically, pain exists to signal actual or impending tissue damage to the organism. In cervical spine disorders, common patterns include nociceptive, neuropathic, and chronic pain (9,10). Musculoskeletal, nociceptive pain arises from nociceptors widely distributed in cutaneous tissue, bone, muscle, connective tissue, vessels, and viscera. Typically described as sharp, dull, aching, or throbbing, nociceptive pain often responds to nonsteroidal antiinflammatory drugs (NSAIDs) and opioids.
Neuropathic pain arises from nervous system injury or disease. Neuropathic pain is associated with burning and hyperalgesias and does not typically respond to NSAIDs (11). Unlike acute pain patterns of either nociceptive or neuropathic origin, chronic pain has extended beyond the period of normal tissue healing. Over time, pain behaviors become globalized and less closely associated with the inciting nociceptive input.
Frequently, a discrete pain generator cannot be identified despite sophisticated imaging techniques. Even when a putative pain generator is identified, directed treatment is not always adequate to resolve the chronic pain pattern. In these patients, multidisciplinary rehabilitation and pain management are preferred to surgery (11,12).
Even in those with an acute pain pattern, the physician must recognize that suffering is the emotional response to a given nociceptive input. Interestingly, the suffering response is markedly affected by factors outside the cervical spine itself (e.g., sleep disorders and depression). Common comorbidities such as fibromyalgia, complex regional pain syndrome, and depression are all associated with inferior results after decompression surgery (13).
PAIN PATHWAYS IN CERVICAL DEGENERATIVE DISEASE
Cervical spine degenerative disease leads to pain through one of several pathways. First, the receptor relays an impulse to the primary afferent nerve. The primary afferent feeds into the spinal nerve (nerve root), which conveys signals to the spinal cord and ultimately the brain (see Fig. 68.1).
Most nociceptive pain is mediated through mechanical, thermal, or chemical receptors and unmyelinated or lightly myelinated afferents, such as A-delta and C fibers. These small primary afferents exhibit little or no spontaneous activity. Discharge frequency increases with increased stimulus intensity and correlates with pain response (14). Local tissue injury leads to persistent, spontaneous bursting activity from the primary afferents at the site (14).
Nociceptive neurotransmitters include glutamate, substance P, VIP, and somatostatin. Most small primary afferents have multiple transmitters. Typically, amino acid neurotransmitters, such as glutamate, result in rapid, short-lasting depolarization while peptide neurotransmitters, such as substance P, cause delayed, long-lasting depolarization (15).
These primary afferent sensory nerves converge as the spinal nerve root, which then supplies the dorsal horn neurons of the spinal cord. Like the primary afferents, the spinal nerve does not normally produce spontaneous discharges (16,17). The dorsal horn neurons are secondorder nociresponsive elements classified by firing threshold (14). Nociceptive specific neurons fire in an all-or-nothing pattern to detect presence of nociceptive signal.
Wide dynamic range (WDR) neurons, on the other hand, have the ability to encode stimulus intensity. These WDR neurons may be activated by stimulation from variety of organ systems. For example, the same WDR neuron is excited by both cutaneous and deep input within a dermatome. Therefore, T5 dermatomal receptors excite the same WDR as the coronary arteries, causing the brain to perceive coronary occlusion as left arm pain (14).
In the spinal cord, sensory signals coalesce into several fiber tracts including the tract of Lissauer, a small C fiber tract. Large afferents are typically conveyed via the dorsal columns. These fibers typically pass rostrally on the ipsilateral side for several segments before crossing to the contralateral side (14,18). Cervical spine afferents collateralize less and, on entering the cord, are more level specific than lumbar afferents (19).
Nociceptive signals are processed in the midbrain and cortex. Brainstem processing includes the medullary reticular formation that acts as a relay station. This medullary relay also incorporates nociceptive input into the autonomic system. In the cortex, there are two important somatosensory areas, SI and SII. SII is more important in pain perception.
NOCICEPTIVE RESPONSE MODULATORS
Subacute pain stems from exaggerated neuronal discharge in response to subthreshold stimuli. Factors released into periterminal milieu after local tissue injury mediate this effect. Important factors include amines, kinins, lipidic acids, cytokines, and peptides. A number of modulators on the terminal of the primary afferent also function to inhibit the release of afferent neurotransmitters. For example, agonists of the opiate or alpha-2 adrenoceptors block C fiber transmission and thus local analgesia (20,21). Li and Zhuo (22) found that multiple receptor agonists and antagonists, including glutamate and serotonin, affect conduction rates in sensory nerves.
Modulators at the nerve root level have been closely studied in the desire to develop pharmacologic interventions for chronic radicular pain. The tachykinins are neuropeptides such as substance P, neurokinin A, and neurokinin B, which bind to dorsal horn receptors (23). Substance P increases synaptic transmission in nociceptive pathways by affecting calcium release from intracellular stores (24,25). Peripheral nerve transection or chronic compression decreases levels of substance P in the cord (24).
NMDA (N-methyl-D-aspartic acid) receptors bind the neurotransmitter glutamate and are found throughout the CNS, including the dorsal horn of the cord. These receptors mediate synaptic plasticity and some forms of memory (26). Blocking these receptors reduces spinal nociception (27). Nitric oxide (NO) functions as a secondary mediator of many of these effects, increasing the responsivity of dorsal horn neurons to nociceptive afferents (28,29).
NO has been found in the cerebrospinal fluid (CSF) of chronic pain patients. Intracutaneous NO injection evokes pain in a dose-dependent manner. NSAIDs may decrease pain by blocking the mediators. These agents inhibit cyclooxygenase and therefore the production of prostaglandins from arachidonic acid in cell membranes (29). While intrathecal substance P injections lead to hyperalgesia, intrathecal NSAID injection antagonizes this effect (15).
Modulation of nociceptive input occurs at the dorsal horn level as well. Repetitive stimulation leads to windup and somatotopic remodeling. With windup and remodeling, the receptive field of the dorsal horn neuron enlarges and responds more vigorously to modestly aversive stimuli (30). Descending inhibitory and excitatory pathways modulate these effects through interneurons at the spinal level.
Over time, the altered sensory input changes the dorsal horn’s processing patterns of afferent information (24). Severing the afferent nerve has this cord reorganizing effect, but long-term tetrodotoxin blockade does not (31). This difference implies that chemical or metabolic factors, not the electrical impulses themselves, are responsible for cord reorganization. A redistribution of central terminals of thick, myelinated afferents from low-threshold mechanoreceptors is observed. This shift could lead to inappropriate responses to innocuous peripheral stimuli and may explain the intractability of neuropathic pain (32).
Over time, modulation of afferent impulses is also mediated through differential gene expression in dorsal horn neurons. For example, the c-fos protein acts as a third messenger in stimulus-transcription coupling in neurons. That is, c-fos encodes transcription factors that regulate target gene expression. Genes for neuropeptides such as the tachykinins and enkephalins are effected (14). This gene level modulation can be blocked by morphine.
CERVICAL DEGENERATION AS AN AXIAL PAIN GENERATOR
The cervical spine contains many potential pain generators. The vast majority of pain is benign, self-limited, and thought to be related to the neck’s myofascial structures. Other axial pain generators include the disk, uncovertebral joints, facets, and ligaments.
Muscular pain and spasm are a common component of neck pain and may result directly from injury or indirectly from overuse, inflammation, or ischemia. In the muscle, pain receptors are either chemonociceptive or mechanonociceptive. Damaged tissues release vascular permeability factors and attract inflammatory cells. Edema, inflammation, and irritation of the injured muscle trigger muscle spasms and involuntary contractions.
Over time, these structures continue to interact with one another. With chronic degenerative disease, pathoanatomic findings and pathomechanical changes are rarely seen in only one portion of the motion segment. For example, gross muscular contractions further load damaged or spondylotic disks and facets (33). Even when deeper pain generators are responsible for the pain, overlying muscular factors often contribute to the pattern. Pain of purely muscular origin, while occasionally severe, is typically self-limited.
The disk is part of a three-point articulated support system including two zygapophyseal or facet joints dorsally (34). As part of a tripod, the synovial zygapophyseal joints allow for a large arc of motion while limiting space loss for the neural elements. Forces applied to the intervertebral disk produce coupled motions in the facets. In the lumbar spine, Kirkaldy-Willis et al. (34) reported that degenerative changes in the disk are mirrored in the facets. However, cervical load bearing differs. In the lumbar spine, 80% of the axial load passes through the disk (35). In the cervical spine, axial load is distributed almost equally between the disk ventrally and each articular pillar dorsally (36).
Cervical spondylosis is a slowly progressive phenomenon. Early disk degeneration typically precedes bony changes by 10 years (37). Later, with decreasing disk height, facet mechanics are altered, increasing mechanical stress at the cartilaginous end plates (38). As with synovial joint degeneration elsewhere, findings include cartilage space narrowing, sclerosis of the bony margins, and osteophyte formation (7). Facet osteophytes occlude the neuroforamen more often than the central canal.
In some cases, mechanical incompetence of disk and facets leads to spondylolisthesis (39). The superior joint surfaces slip backward eroding into the lamina below. Alternatively, osteophytes form to stabilize the vertebral motion segment (38). As disk degeneration progresses, bone morphogenetic protein (BMP) receptors move from cartilage cells to fibrous cells. The new BMP receptivity may promote osteophyte formation (40).
Osteophytes increase vertebral end plate weight-bearing surface in proportion to the square of the new vertebral radius (41,42). Mechanically, the increased surface area decreases force per unit area. Osteophytes also decrease motion, acting as a “limiting mechanism” against further deterioration. In some cases, osteophytes effectively “fuse” the degenerated segment (7).
In one series of 260 patients with cervical spine disorders, flexion/extension radiographs identified hypermobility in 14.5% (151) of the motion segments (43). Hypermobility correlated with magnetic resonance imaging (MRI) findings of early or moderate degeneration. Others report increased mobility in only mildly degenerated disks. More severely degenerated disks exhibit less motion (34). These findings led to Kirkaldy-Willis’ hypothesis of three phases of degeneration:
Dysfunction
Unstable phase
Stabilization
CERVICAL DISK DEGENERATION
As in the lumbar spine, recurrent, debilitating, and intractable axial pain is often attributed to degeneration of the cervical intervertebral disk. However, given the far smaller percentage of axial load passing through the disk, the pathomechanics are certainly not analogous to the lumbar condition. In a recent best evidence synthesis, radiographic evidence of disk degeneration was not identified as a risk factor for axial neck pain (3).
While distinguishing between the confluence of nerve fibers supplying the disk, posterior longitudinal ligament (PLL), and periosteum remains difficult, the outer portions of disk are likely innervated by sinuvertebral nerve complex (44). Occurrence of nerve endings below the outer lamellae of the annulus is still controversial (45).
The sinuvertebral nerve emerges just distal to the dorsal root ganglion (DRG) whereupon it reenters the foramen. The sinuvertebral nerve then arborizes over two levels on the PLL. This overlapping, dual innervation provides a less-than-precise anatomic basis for the painreferral patterns seen during diskography. The sinuvertebral nerve principally conveys proprioceptive information from a combination of mechanical deformation and position receptors. Overstimulation of the sinuvertebral nerve complex causes the surrounding muscles to go into spasm (46,47) (see Fig. 68.2).
Buckwalter (48) described cervical spine degeneration as a cascading process beginning in the intervertebral disk. The intervertebral disks are the largest avascular tissues in the human body (49). Disk nutrition derives from diffusion across cartilaginous end plate. Normally, oxygen tension drops at the center of the disk. With spinal degeneration, the end plates become sclerotic, thereby decreasing nutrient exchange. Thus, sclerosis accelerates disk degeneration as one of several “vicious cycles” of cervical spondylosis.
A number of factors influence the rate and degree of disk degeneration, including loading, genetics, and local autocrine factors. An underlying susceptibility to disk degeneration is genetically coded but accelerated by repeated subclinical traumas to the joints of the spine. Early biochemical degeneration produces mechanical destabilization. With mechanical change come additional, abnormal stresses borne by the spinal articulations. Mobility changes and lost disk height incite the second vicious cycle of progressive degeneration.
The cells of the intervertebral disk are metabolically active and are capable of responding to both biochemical and biomechanical stimuli. Axial loading is implicated in disk degeneration through its frequency in the lordotic portions of the spine (50).
These pathomechanical changes begin biochemical changes in the nucleus pulposus. The nucleus is a three-dimensional lattice of collagen fibrils and a protein-polysaccharide complex (7). These hydrophilic polysaccharides cause the disk to imbibe water. The normal nucleus pulposus acts as a contained fluid (51). The spine converts axial loads to tensile strain on annular fibers, which then transmit the force to the vertebral end plates. With continuous loading, the nucleus exhibits creep.
When compressive force exceeds the disk’s swelling pressure, water is forced out, altering intradiskal stress distribution. These stresses precipitate a number of harmful, dose-dependent responses, beginning with nuclear cell apoptosis (51). With down-regulation of the collagen II and aggrecan genes, the annulus fibrosis becomes increasingly disorganized. Autocrine factors signal disk cells to either increase or decrease disk degeneration. For example, matrix metalloproteinases decompose aggregating proteoglycans, contributing to both the articular cartilage and intervertebral disk destruction.
With age, the disk’s gel structure degenerates. Starting in the second decade of life, the collagen content of the disk increases while glycoprotein content decreases (44). Loss of glycoproteins decreases imbibition pressure. When relaxed, the degenerated disk imbibes fluid, but under pressure, this fluid is not retained. Over time, disk hydration decreases
from 88% weight by volume to 69% (52) (see Fig. 68.3). The overall mechanical effectiveness of the disk decreases with decreasing hydration (7). Diminished intradiskal pressure retention decreases effective force distribution (52).
from 88% weight by volume to 69% (52) (see Fig. 68.3). The overall mechanical effectiveness of the disk decreases with decreasing hydration (7). Diminished intradiskal pressure retention decreases effective force distribution (52).
Cells of the intervertebral disks increase their production of matrix metalloproteinases when stimulated by interleukin-1 (53). Endogenously produced NO strongly inhibits the production of interleukin-6. The matrix metalloproteinase-3-positive cell ratio correlated with the degree of degeneration on MRI (54). Further, degenerated disks exhibited matrix metalloproteinase-3 but no metalloproteinase tissue inhibitor. For cervical intervertebral disks, the matrix metalloproteinase-3-positive cell ratio correlated with osteophyte size. Cathepsins and other proteolytic enzymes are also involved in disk degeneration, forming clefts that separate the disks from their vertebral bodies (55).
The normal annulus functions to contain the nucleus and convert compressive stress to tangential stress. When nuclear hydration fails, strain increases at the nucleusannulus interface. Under constant compressive loading, the central annular lamellae buckle. Subsequent disk collapse causes outward bulging of the external concentric bands of the annulus fibrosis (see Fig. 68.4).
Increased annular stress leads to fibrillation and tearing of the annular fibers. Especially in younger patients, nuclear material can protrude through these defects. Thus, disk herniation falls along the continuum of degeneration (56). Disk herniations become less likely with progressive nuclear dehydration. While acute disk herniation also
complicates chronic spondylotic changes, annular degeneration and protrusion predominate in older patients.
complicates chronic spondylotic changes, annular degeneration and protrusion predominate in older patients.
The last several years have recorded progress in our understanding of disk degeneration pathophysiology (57). Over time, it is hoped that this understanding will produce new biologic therapies, including molecular, gene, and cell-based strategies, to reverse degeneration. Today, the difficult mechanical and vascular environment of the disk has conferred only limited clinical success. Currently under way in Europe, the EuroDisc clinical trial expands ex vivo and reimplants disk cells removed at discectomy.
THE FACETS
With loss of disk height, the facets override, increasing point loading. With decreasing facet competence, segmental motion increases, further hastening disk degeneration (56). The contribution of facet arthrosis to cervical axial pain syndromes remains poorly understood. Cervical facet arthrosis has been implicated in pure axial pain, occipital headache, and ear pain. Stimulation of zygapophyseal joint afferents produces a discrete referral pattern mimicking cervical radiculopathy (58). Marked reduction in nerve activity can be achieved with lidocaine facet injections (35).
Zygapophyseal joint degeneration generates pain indirectly through subchondral bone fracture, ligament attenuation, and excessive muscle strains and injury (35). The bone of the cervical spine remains a metabolically active composite tissue affected by a variety of physiologic processes. Subchondral sclerosis is an example of a dynamic response to injury. Fine nerve endings with pain-related neuropeptides are found in the marrow, periosteum, and cortex. These fibers may play a direct role in bone pain, triggering intraosseous hypertension or ischemia (41,59).
The facets are richly innervated by the posterior primary rami, including substance P-containing fibers (35). Encapsulated mechanoreceptors respond to capsular distention, ligamentous instability, and direct trauma. Periarticular receptors respond to chemical stimuli. The facet meniscoid represents a highly vascularized and innervated infolding of the capsule (60). With age, the meniscoid atrophies and disappears.
One recent study of 215 patients with cervical spondylosis examined the imaging, clinical characteristics, and significance of their cervical facet hypertrophy. The patients were divided into 32 with hypertrophic facets and 183 without. Most hypertrophy was unilateral and involved one level. Changes were most frequent at C4-C5 and gradually diminished both cranially and caudally from that level. Facet hypertrophy was seen significantly more frequently in males and was predictive of neck pain in nearly 80% of cases (61).
Facet arthrosis was graded in 465 skeletally mature human cervical spines from the Hamann-Todd Collection in Cleveland (62). They graded the specimens from normal (0) to ankylosed (3) [DNS: preceding (0) and (3) are not references]. Grading bilaterally over five levels yielded 4,650 specimen assessments. In this study, facet arthrosis was encountered more frequently in the upper cervical spine, with fairly equal involvement of C2-C3, C3-C4, and C4-C5. More significant involvement favored the C4-C5 level. The prevalence of cervical facet arthrosis increases with age.
THE UNCOVERTEBRAL JOINTS, LIGAMENTS, AND HYPERMOBILITY
Axial neck pain may arise from malalignment and hypermobility. Both increase the muscular work required for daily activities. For example, positive sagittal balance places the head in front of the center of gravity. Such as holding a weight away from the body, this position markedly increases the forces against which the cervical extensors have to work. Similarly, hypermobility requires cocontraction of cervical flexors and extensors to decrease shear force across the motion segment. In healthy controls, the sternocleidomastoid (SCM) fires before neck motion begins, for example (63).
Both malalignment and hypermobility may arise from disk degeneration. Cervical vertebral bodies are bigger dorsally and smaller ventrally. Therefore, cervical lordosis arises from the relatively larger disk height anteriorly (7). As the disks lose height, the cervical spine loses lordosis.
Uncovertebral joints begin to touch dorsally preventing further dorsal height loss. Preferential anterior height loss continues (64). It is unknown to what degree uncovertebral joint arthrosis contributes to axial neck pain. Uncovertebral joint apposition clearly leads to osteophyte formation and subsequent root compression (see Fig. 68.5). Disk collapse and uncovertebral joint apposition fosters changes in spinal alignment and segmental motion.
The cervical spine ligaments, such as the PLL, are richly innervated (65,66). While there is a significant interdigitation of annular fibers and the PLL fibers, the nerve endings in the PLL are more numerous and varied. Mechanical distortion or disruption of fibers in the posterolateral aspect of the intervertebral disk generates pain after disk
protrusion. Segmental instability may stimulate a similar pain response.
protrusion. Segmental instability may stimulate a similar pain response.
Malalignment and regional hyper- and hypomobility affect the cervical ligaments, which also lose their elasticity and thicken with age (67). Disk collapse increases tension on the surrounding ligaments and renders the ligamentum flavum redundant. The flavum buckles inward (see Fig. 68.6).
CERVICOGENIC HEADACHES
Axial pain remains problematic from both a diagnostic and therapeutic standpoint. Unlike radiculitis, in which the pain’s distribution may track to a specific root level, the location of axial pain does not reliably identify its generator. Axial nociceptors share segmental innervation with the levels above and below.
Cervicogenic headaches offer an excellent example of the difficulty in understanding axial pain syndromes. These pain complaints are common but remain poorly understood. Headaches have been attributed to muscular factors with enthesopathic pain at the insertion of the deep extensors on the occiput. Others attribute the symptoms to upper cervical radiculopathy with associated greater or lesser occipital nerve irritation.
Proponents of an upper cervical origin argue that symptoms often stem from cervical trauma and are provocative with cervical motion and loading. In these patients, pain often occurs over or along the C2 or C3 nerve roots. In one prospective case study, 39 patients, all of whom had undergone extensive diagnostic testing, underwent unilateral blocks (70). The patients were followed for 6 months. Both initial and final injections were rated 40% effective. By 6 months, the headaches had returned to pretreatment levels.
More significant and lasting relief of cervical headache was reported in a post hoc analysis of the prospective Prestige and Bryan cervical arthroplasty studies (71). More than 800 anterior cevical decompression and fusion (ACDF) and total disc replacement (TDR) patients were available for a 2-year follow-up. Pre- and postoperative Neck Disability Index measures revealed significant improvements in headaches with both procedures. The authors concluded that ventral cervical procedures could decrease cervicogenic headache symptoms. These results, however, may arise from either discogenic or radicular factors. That arthroplasty improved headache more than ACDF implies that the pain syndrome is not likely facet mediated.
To test the influence of lower cervical radiculopathy on the causal effect of headaches, a variety of other injection studies have been performed. In one consecutive series of 275 patients with cervical radiculopathy, 161 reported daily or recurrent headache (72). In this series, the headaches were most unilateral and ipsilateral to the radicular pain. When selective nerve root blocks were performed, 59% reported that more than half of their headache had resolved. The authors concluded that root compression in the lower cervical spine producing radiculopathy also induced headache. In their patient population, headaches were also associated with higher neck and arm pain intensity and greater functional limitations.
CERVICAL RADICULOPATHY
Cervical radiculopathy implies nerve root dysfunction. Clinically, this dysfunction commonly manifests as pain radiating from neck into the arm in the affected root’s distribution. Radiculitis implies pain only while a complete radiculopathy implies myotomal weakness, numbness, and diminished reflexes.
Most cervical radiculopathy of degenerative origin has a self-limited clinical course with up to 75% spontaneously improving. When time and nonoperative management fail to alleviate the patient’s symptoms, surgical decompression of the root is recommended. Ventral and dorsal cervical decompression procedures are associated with 80% to 90% rates of arm pain relief (73). Especially in the cervical spine, the clinician should not overrely on a discrete dermatomal pattern. In one study, root compression led to dermatomal pain in under one-third of the cases (74).
NORMAL NERVE ROOT FUNCTION
Each nerve has two functions:
Electrical impulse propagation
Axonal transport of proteins and organelles
Depending on how they impact these functions, nerve injuries are divided into primary and secondary axotomy and impaired axoplasmic transport (75). Primary axotomy implies torn fibers. Secondary axotomy refers to an
increase in periaxonal spacing. The extent of each of these findings is concomitant with functional injury.
increase in periaxonal spacing. The extent of each of these findings is concomitant with functional injury.
Both of these functions require energy and can be blocked by local ischemia or root compression (16,76,77). Different cellular mechanisms are responsible for disruption of these functions. That is, a nerve segment unable to conduct impulses may maintain axonal transport.
The nerve root (spinal nerve) has two parts. Its ventral efferent conveys motor information. The dorsal, afferent roots convey sensory information (78). These elements are contained together in the root sleeve, an extension of the dura. Within the root sleeve, the neural elements are soaked in CSF.
NERVE ROOT VASCULARITY AND AUTONOMIC ISSUES
Spinal nerve blood supply is sparser than that of peripheral nerves (14). CSF diffusion confers some of the root’s nutritional needs (79). The blood-nerve barrier limits the flow of albumin, rendering spinal nerves more susceptible to edema than peripheral nerves (80).
![]() Figure 68.7. Depictions of the radicular vascular supply. A: Main sources of arterial blood for the nerve roots and the cord. In the upper cervical spine (C1 to C4), the cord is supplied by the anterior spinal artery from intracranial sources. From C5 to T1, the anterior spinal artery is supplied by a unilateral medullary feeder, usually near C6. Alternatively, this feeder may arise from C3 or C4. B: Local blood supply at the root level. The intermediate branch of the segmental artery (1) enters the canal dividing into an anterior spinal canal branch (2), a nervous system branch (3), and a posterior spinal canal branch (4). A ganglionic plexus (5) is created where the lateralward nervous system branch joins the nerve root and meets the medialward supply from the caudal nerve root arteries (7) in the cranial half of the root (8). Other segments of the nervous system branch pass medially to become medullary feeder arteries (9). One branch passes anteriorly to contribute to the anterior spinal artery (10). The other branch contributes to one of the two dorsal spinal arteries (11). C: Photograph of an injected neonatal spinal cord. Note that the anterior spinal artery receives thoracic- and cervicallevel medullary feeders. Lesser degrees of supply pass through the segmentals at each foramen. (A and C from Parke WW. Correlative anatomy of cervical spondylotic myelopathy. Spine 1988;13(7):831-837; B Adapted from Rydevik B, Hasue M, Wehling P. Eitology of sciatic pain and mechanisms of nerve root compression. In: Wiesel SW, et al., eds. The lumbar spine, 2nd ed. Philadelphia: WB Saunders, 1996:123-141.) |
Segmental arteries carry radicular blood supply. Outside the spine, approaching the foramen, these arteries divide into three branches. The ventral branch supplies visceral structures ventral to the spinal column. The dorsal branch supplies the paraspinal muscles and facets. The intermediate branch arborizes to supply the spinal canal contents, including the DRG, ventral root, dorsal root, and the vasocorona of the cord itself. These vessels run parallel to the nerve roots, but there is no apparent connection with them (81,82).
The nerve root’s vascular supply enters from two directions, the intermediate branch distally and the cord’s vasocorona proximally. These vessels run in the epipia, the outer layer of the root sheath. They anastomose at a point two-thirds of the root’s distance from the cord in a watershed area vulnerable to insufficiency (83) (see Fig. 68.7).
Radicular feeders are innervated by the autonomic nervous system. These fibers contain adrenergic, cholinergic, peptidergic, and nitroxydergic receptors (84). Inflammatory mediators may affect root circulation through these perivascular nerve plexuses.
Root compression causes pain through both direct and indirect mechanisms. Direct pain generation occurs through deformation of the rich nervous plexus around the spinal nerve and ventral dura (19). These fibers travel with the root’s vascular elements and convey both somatic and sympathetic information through the sinuvertebral nerve (85). The dorsal root and dura, on the other hand, carry very few nerve fibers (86).
Additional evidence of sympathetic nervous system activity in radiculopathy is seen in a sympathectomy model. After sympathectomy, mechanical hypersensitivity and allodynia are significantly attenuated (87).
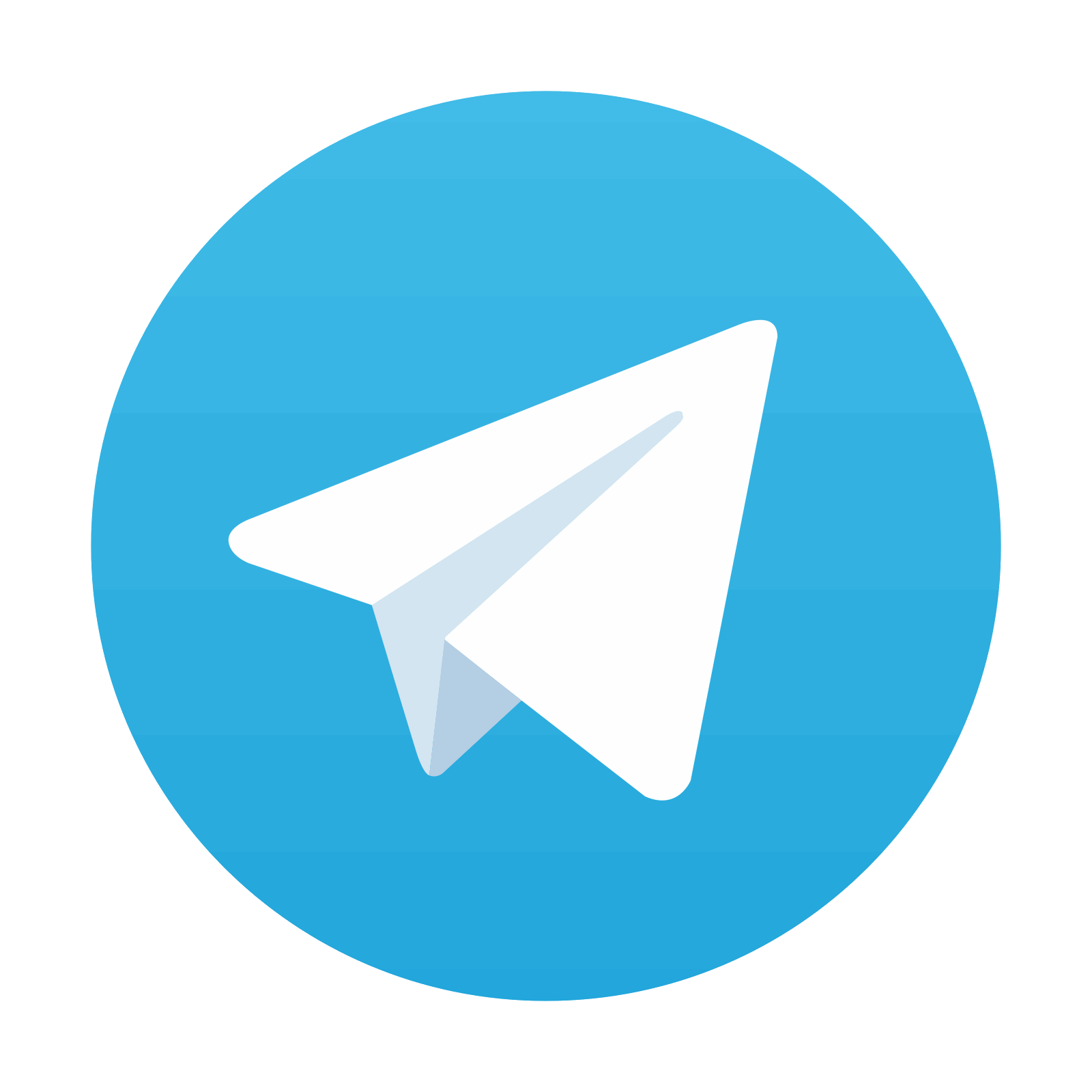
Stay updated, free articles. Join our Telegram channel

Full access? Get Clinical Tree
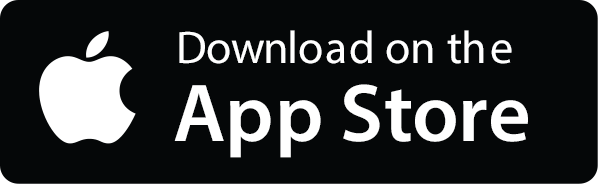
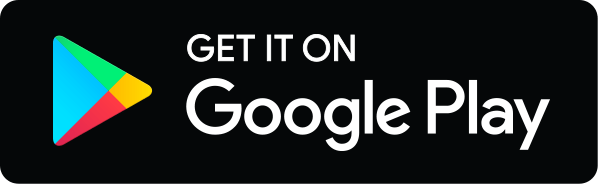