!DOCTYPE html PUBLIC “-//W3C//DTD XHTML 1.1//EN” “http://www.w3.org/TR/xhtml11/DTD/xhtml11.dtd”>
Pain Assessment in Cognitively Impaired Animals
Lydia Giménez-Llort and C. G. Pick
“Pain” is defined by the International Association for the Study of Pain (IASP) [76] as “an unpleasant sensory and emotional experience associated with actual or potential tissue damage or described in terms of such damage.” Such a psychophysiological experience is difficult to assess wholly in nonhuman animals, and it is still not exempt of speciesist discussions. Thus, some still consider that the conscious nature of the pain experience would not entitle other animal species to feel “pain” as it is conceptually understood in humans. Since nonhuman animals exhibit an aversive response to noxious stimulation, the pure physiological concept of “nociception” [133]—defined as “the neural processes of encoding and processing noxious stimuli”—was traditionally recommended to be used instead [102]. Nevertheless, the translational relevance of the study of pain in nonhuman animals provides new insights to this regard, and it is the focus of the present chapter.
TRANSLATIONAL RELEVANCE OF THE STUDY OF PAIN IN NONHUMAN ANIMALS
Like in humans, the primary goal of studying pain/nociception in the other animal species is to prevent, alleviate, and relief the subject from the perception of noxious stimuli as well as to improve anesthetic and analgesic management. These are issues that have classically received most attention for domestic and farm animals [101] but also apply for laboratory specimens [55, 68]. It is reflected by the increasing public and scientific concern regarding animal welfare [146], which leads to improved and refined ethical guidelines and regulatory imperatives in the investigations of “experimental pain” in conscious animals [3, 22, 30, 62, 100, 123, 124] and animal “pain” scales [115]. The other undeniable relevance of the study of pain in nonhuman animals is their significant contribution to the understanding of the fundamental neurobiological mechanisms of pain that we all have in common. Their role as models for developing new and better analgesic compounds and management strategies becomes critical [66]. For the current topic, the translational relevance of the study of pain in nonhuman animals originates from the option to easily study the impact of impaired cognition and dementia. These are clinical situations that render the concepts of perception, assessment, and management to be an exceptional challenge. In the following paragraphs, we will first briefly present two key examples of close fruitful scientific cooperation between basic and clinical sciences in the study of pain that empathize the relevance of complementary but also synergistic bidirectional translational efforts. They illustrate how the translational approach, with joint basic and clinical research, is contributing to the faster advancement of knowledge that is needed to improve pain relief in both humans and nonhuman animals. This fast progress in knowledge is even more important when our frail aging populations are considered.
Among scientists, clinicians, health-care providers, and policymakers devoted to the study of pain and the translation of basic knowledge into improved pain relief worldwide—as the mission of IASP is defined—there is a growing number of those who are interested in “pain and pain management in nonhuman species” [76]. The aims of this “special interest group” of IASP are (1) to encourage basic and clinical research on the recognition of pain in animals, mechanisms of pain in animals, and management of pain in animals; (2) to encourage the use of spontaneous animal disease as a model for studying mechanisms and alleviation of human pain; (3) to encourage cross-species collaboration in pain research; and, finally, (4) to promote interdisciplinary discussion of pain in nonhuman species. Each of these statements reveals the significance, new meaning, and vision that assessment of nociception in nonhuman animals has been achieved in the last decades. More importantly, they can be foreseen eventually guiding the future conceptual and ethical frames that will lead to a better scenario for the study of “pain” and “pain management” in animals as well as its translational output to pain relief in humans.
Similarly as translational approach, the “European Cooperation in the Field of Scientific and Technical Research” (COST), an intergovernmental framework for fostering collaboration between researchers in Europe in a bottom-up manner, has also brought together leading researchers from a wide range of scientific disciplines in a COST Action TD1005 for “Pain Assessment in Patients with Impaired Cognition, Especially Dementia,” to which the authors of this chapter belong [51]. It is an effort to prompt the urgently needed improvement of pain management in dementia since evidences warn that pain is grossly undertreated in these patients [31]. The major aim of this COST Action is the development of a comprehensive and internationally agreed-on assessment toolkit for older adults targeting the various subtypes of dementia and various aspects of pain, including pain diagnostics, cognitive examination, and guidelines for proper assessment [1, 75]. It will have an obviously relevant application in the management of pain in palliative care [5, 46, 75]. For the validation of this toolkit, joint action of both basic and clinical sciences has been requested. For this purpose, neuroscientists, physiologists, pharmacologists, and neuropsychologists are participating and together providing experimental tests for the validity of the tools and physiological markers of pain, which do not solely rely on self-report. Experimental designs will be used to help testing the validity and pain specificity of the toolkit in development. To do this, different pain biomarkers (e.g., EEG, ERP, facial expression, autonomic responses [ECG, skin conductance]) are being assessed in individuals with different types of cognitive impairment (Alzheimer’s, frontotemporal dementia [FTD], vascular dementia, mild cognitive impairment, mental retardation) using different types of experimental pain induction procedures [38]. Of course, the impact of cognitive impairment on pain processing is also investigated in murine models trying to reproduce the cognitive deficits of Alzheimer’s disease (AD) [8]. Special attention is paid to study nociceptive behaviors at the different stages of the disease and how the neuropathological and neuropsychiatric-like symptoms affect perception and processing of noxious stimuli.
Species [136] and even strains [45, 94, 110, 111, 116] may show different behaviors to a similar noxious stimulus. Nevertheless, the homology of “nociception” among animals and “pain experiences” in humans—including the conscious sensation of “pain”—has rendered some species, mainly rodents, a relevant model in basic and preclinical pain research [66]. Thus, tests and animal models of nociception in rats and mice [93] complement the use of molecular, biochemical, and cellular experimental approaches, which are limited by the absence of other factors known to drive and modulate pain in human individuals. These factors are the wide variety of external stimuli as well as those idiosyncratic factors such as the cognitive and emotional traits and states [11, 70] but also gender and age [15, 109]. Overall, the animal models have significantly contributed to our understanding of the physiology, pathophysiology, and pharmacology associated with experimentally induced or naturally occurring acute and chronic pain states [15, 109]. Still, controversies questioning the face and predictive validity of these models (mostly for chronic pain) arise, and basic and preclinical researchers are challenged to ensure the expected and necessary translational results [80, 109].
In a review of the actual progress and challenges of animal models of pain, Mogil [109] examined the factors that may underlie the slow translational progress in spite of the increasing availability of mutant animal models, assays that more closely resemble clinical pain states, and dependent measures beyond simple reflexive withdrawal. Although his critical analysis was done with regard to the effectiveness and safety of analgesic drugs, he took notice of the confusion that results from the different use of the term “animal model.” Mogil claimed the existence of the following three types of concepts of “animal model” in the literature:
The Pain Test as the “Animal Model”
Classical studies of pain use the concept of “animal model” to refer to the “experimental procedure” or “laboratory method” by which the noxious stimulus is applied to the subject. A wide range of assays seeks to mimic human forms of clinical pain in hopes of gaining a better understanding of the etiology and pathophysiology of disease-related pain phenomena: nociceptive (thermal, mechanical, chemical, or electrical stimuli), inflammatory (algogen and sensitizing compounds, inflammatory mediators, polyarthritic, or monoarthritic agents), or neuropathic (mechanical or surgical lesions). The time point postinjury (acute, persistent, or chronic) and the part of the body studied (cutaneous, muscular, orofacial, visceral) are the other features that are chosen to define the experimental design. Examples of the most prominent pain induction methods are the hot-plate test, the pinch test, and the formalin test. All these models allow the experimenter various degrees of control over the parameters of pain stimulus: intensity, duration, location, and temporal patterning.
The Measures of Pain as the “Animal Model”
This concept of “model” may also refer to the wide range of responses that can be measured. The responses can be a simple spinal withdrawal reflex response to sudden mechanical or thermal (heat or cold) stimuli such as induced in the tail-flick nociception test but also an avoidance response requiring higher brain centers such as jumping up or raising or licking the forepaw as elicited in the hot plate nociception test. Thus, responses may also involve spontaneously emitted behaviors: the so-called directed behaviors such as biting, flinching, guarding, licking, lifting, and shaking or—as the most severe behavioral expression—autotomy. Gait, walking, sitting, standing, and posture are also possible measures. Finally, nociception measures may consist in the choice the animal takes to avoid noxious stimulus in operant behaviors such as learned escape, place aversion, or reinforcement conflict. Indirect measures of nociception can be pain-affected complex behaviors such as anxiety, disability, changes in attention, sociability, or sleep. However, they are not preserved from being confounding factors, too.
The Subject as the “Animal Model”
The animal “model” may refer to the “experimental subject.” That is a species or strain that spontaneously develops a painful disease or that is sensitive or resistant to pain. Mutant animals such as transgenic mice and rats, knockout and knock-in mice have become experimental subjects with a target mutation that modifies the pain phenotype. The idiosyncratic factors of the subject that will influence the study of pain are the genetic background (of the mutation), the gender, and the age. The husbandry (cage density, diet, social factors) and the context of the testing procedures (arousal, communication, handling, and restraint) are the most important environmental factors that must be controlled for as well.
PAIN-RELATED COMORBIDITY AND PAIN IN DEMENTIA
In the last two decades, the modeling of neurological and psychiatric diseases [56] in spontaneously mutant or genetically engineered rodents, in parallel to classical neuropsychopharmacological approaches, has provided new chances to study pain comorbidity [17, 99]. Analgesia is one of the behavioral items included in standardized protocols that screen for comprehensive phenotypes of animal models of disease prior to further functional analysis [33, 79, 121]. However, a limited number of experimental studies have yet examined the comorbidity of pain in cognitively impaired rodents or in naturally occurring canine and feline “cognitive dysfunction syndrome” (CDS) [89, 90]. A recent review [99] covers this important gap—but still from an opposite perspective—because it focuses on the negative impact that chronic pain has on affective disorders and cognitive dysfunctions as pain-related comorbidities. The question about pain perception and assessment in animal models of cognitive impairment or dementia is, for several reasons, still scarcely addressed and request the best endeavor undertakings. Some of the key questions are (1) how these diseases compromise the characteristics of pain, (2) how the validity of the models is to replicate pain in mild to moderate AD [78], or (3) what can we learn from these animal models whose shorter generation cycles and life spans imply a substantial advantage for longitudinal studies.
The first obvious limitation for the translational assessment and quantification of human pain is that self-report of the aversive nociception requiring verbal expression is not possible in nonhuman animals. Instead, pain must be estimated from the measurement of a range of responses, from simple avoidance reflexes to more complex behavioral responses of the animal to the nociceptive stimuli, as detailed before [93]. This is to the extent that some authors advice to rather refer to them as “tests and animal models of nociception” [11, 102]. Interestingly, this scenario resembles the difficulties that neurologists and psychiatrists face when they assess pain in patients with poor or absent verbal communicative abilities, cognitive impairment, or even dementia [4, 29], including patients in palliative care [5, 75]. Most authors agree that the combination of pain and dementia makes pain recognition in humans much more difficult, resulting in major diagnostic challenges [104]. Interestingly, preclinical pain research has gained interest because of its experience with the restrictive frames set by behavioral pain assessment tools that rely on nonverbal communications or behavioral responses. Since the first descriptions of nociception tests in the 1940s [34, 143], the perception of experimental pain has been measured with standardized methods that assess nociception by means of reliable and objective scores. Best example is the latency of appearance of an avoidance behavior, usually a nocifensive withdrawal reflex of the paw or tail exposed to the thermal (heat or cold), mechanical (von Frey filaments) but also electrical or chemical stimuli [93]. As mentioned before, the measurable behavioral changes may also be spontaneously emitted behaviors (autotomy, goal-directed oral or body movements, gait or posture), operant behaviors (learned escape, place aversion, reinforcement conflict) and changes induced by pain in complex cognitive (attention, memory), emotional (freezing, neophobia, anxiety-like behaviors, behavioral despair), physical (e.g., disability), physiological (e.g., sleep), or social behaviors [71, 109]. Some other authors also consider the traditional observer assessments of the body appearance (i.e., coat condition, piloerection, anorexia), posture/gait (i.e., hunched posture, abnormal gait), demeanor (i.e., aggression, hiding) but also objective assessments of locomotion and motor activity, food and water intake or changes in body weight and physiological measures (respiratory rate, heart rate, and blood pressure). Still, some authors warn that the relative high basal heart and respiratory rates of small mammals present technical difficulties in their use as physiological indices. Therefore, alternatives such as food and water intake and weight loss have been used for the evaluation of chronic pain, without perfect confidence in the precision of these measures [101]. Systematic evaluation of simultaneous behaviors in complex ethograms can also be done, although their sensitivity to analgesics as well as their specificity, reliability, and frequency are not considered to be completely satisfactory [109].
ON THE RELEVANCE OF NONVERBAL COMMUNICATION IN THE STUDY OF PAIN IN DEMENTIA
Most of the observational pain behavior rating scales that have been developed to assess pain in patients with dementia include classical nonverbal communication categories such as facial expression with furrowed brow, narrowed eyes, closed eyes known as “facial action units” (FAU), body movement (e.g., freezing, crouching, limping), and vocalization (e.g., groaning, mumbling, sighing) [29]. These behavioral indicators of pain are considered relevant for guiding the management of pain in dementia [1] and their translational relevance and applicability have started to be considered. Especially, the facial indicators assessed by the Facial Action Coding System (FACS), which seem to specifically encode the experience of pain and do only change little in the course of dementia [72, 92], have been successfully translated in the so-called grimace scale (GS) [91]. In rats (RGS) and mice (MGS) the GS comprises 4 or 5 FAU, respectively. The GS shows a high reliability (ICC: 0.9) and accuracy (72–97%) in mice [91] but is even more accurate in rats (accuracy >81%, ICC also 0.9) [135]. Besides, the scale showed a high correlation with other behavioral pain scores and can effectively assess pain associated with routine procedures [91]. In contrast, contradictory opinions exist on the utility of other spontaneously emitted pain behaviors such as the audible and ultrasonic vocalizations [19]. Their fine computerized recording has allowed the standardization of the measure in rats [65], and they have been found also useful to assess acute pain in laboratory mice [142]. However, other authors argue that they are not specific for the pain status [81] and do not correlate with behavioral measures of persistent pain [141]. Further studies using other experimental settings together with the improvement of our methodological skills to discriminate different vocalization registers, as achieved in cats [21] and in rats [65], will provide new insights in the vocal expression of pain in nonhuman animals that may closely resemble human verbal and paralanguage pain expression.
LIMITATIONS AND CONFOUNDING FACTORS IN THE STUDY OF PAIN IN DEMENTIA
The criticisms of the poor predictive validity of the animal models for chronic pain [13] may also apply to animal models for dementia when studied with experimental pain. That is in part due to the interspecies gaps in terms of pharmacodynamics and pharmacokinetics [14]. Furthermore, the background factor “aging” has strong implications and differs from one species to the other. The short life-span of small animals is an obvious advantage to answer questions that otherwise require too much time to be answered by the only means of human studies. However, this idiosyncrasy may also turn into a limiting factor as the life-span of small animals may be too short to perfectly mimic the time-depending modifications of the “neuromatrix” [6] that are involved in the processing of the noxious stimuli in the elderly patients. Here, those large animals, which mostly develop pain spontaneously [70], are likely to be the more successful models of pain in aging. However, Livingston [101] drew attention to the situation that, in contrast to humans, many large species show much less overt behaviors during noxious stimulation as a survival strategy to avoid showing signs of pain and weakness that may increase the chances to become a prey. Similarly, small rodents show specific pain-related behaviors, which are not always noticeable during casual observations [122, 123]. Among the limiting factors, the ethical considerations as regards the study of large animals are the most sensitive issues that restrict research on them [100], while studies on pain and pain management are very welcome as soon as these animals were seen as domestic or farm animals [101]. Interestingly, the “CDS” is a naturally emerging disease of the aging canine and feline brains [89] that is also considered useful from a translational perspective as a canine model of human brain aging and AD [69]. Yet, second-generation models (transgenic rodents harboring the human familial Alzheimer’s disease [FAD] mutations) that closely mimic the temporal, neuroanatomical, and behavioral patterns of the human disease have greatest advantages due to their shorter time requirements [60].
Cognitive impairment or intellectual disability in humans have historically raised the question to which extent the influence of pain-modulatory factors differs from patients with other conditions [107], in spite of the apparent methodological limitations due to the doubtful level of understanding of the pain tests and the poor reliability of self-report in consequence of the limited ability to communicate. In nonhuman animals, the individual cognitive and emotional traits and states as well as prior experiences of the animal but also external influences such as the interaction with the researcher, the novel context and the restrain procedures are considered important—sometimes stress-related—confounders. These problems are counteracted by procedures including handling, preparatory experiences, or habituation in order to control and stabilize the influence of the confounding factors [16] as well as to establish a basal line of background noise. Such experimental problem solutions are not always available in clinical care settings. Moreover, each animal model (test, measure, or subject) has its strengths and weaknesses, and therefore, special precautions dependent on the model are strongly recommended to be taken [82, 83]. Whenever possible, a battery of several nociceptive assays is also advised in order to confirm the findings by different tests. Even in this case, important factor interactions effects with gender, tester, and cohort are needed to be controlled to avoid confounding results [112].
ANIMAL MODELS OF AGING, COGNITIVE IMPAIRMENT, AND DEMENTIA
During the last two decades, the neurobiology of aging has emerged as one of the scientific disciplines with a vast and quick expansion and its future looks promising. The major methodological advances in recent years offer a unique scientific capacity to study aging. However, the dramatically increasing prevalence of neurodegenerative diseases in the elderly, in parallel with the increase of life expectancy and social aging, makes such an historical success both a challenge and obligation.
Most scientific research efforts have focused on the understanding of the genetic basis and the brain mechanisms (neuronal and glial) related to changes in the nervous system that occur during aging and may underlie neurodegenerative diseases. To support these efforts from the side of animal research, we could make use of three tremendously valuable principles: (1) the similarity in the basic biological mechanisms of the various animal species, (2) the difference in longevity between them and (3) their different complexity. Thus, those species, which are simpler, produce easier “biological” scenarios for a better understanding of crucial etiological processes. For example, a 18-month-old mouse, a 30-day-old Drosophila melanogaster (fruit fly), or the small nematode Caenorhabditis elegans, which has only 1099 genes and lives only for about 3 weeks, all of them have the equivalent biological age of an octogenarian human. This higher pace in animal research allows us to ask, seek, and answer pressing questions for humans and in particular for the sick and their families, which would otherwise take years to be addressed or are even totally inaccessible.
A wide variety of animal models of cognitive impairment has been established based on genetic, lesion, and pharmacological approaches or naturally occurring aging-related cognitive impairments [95
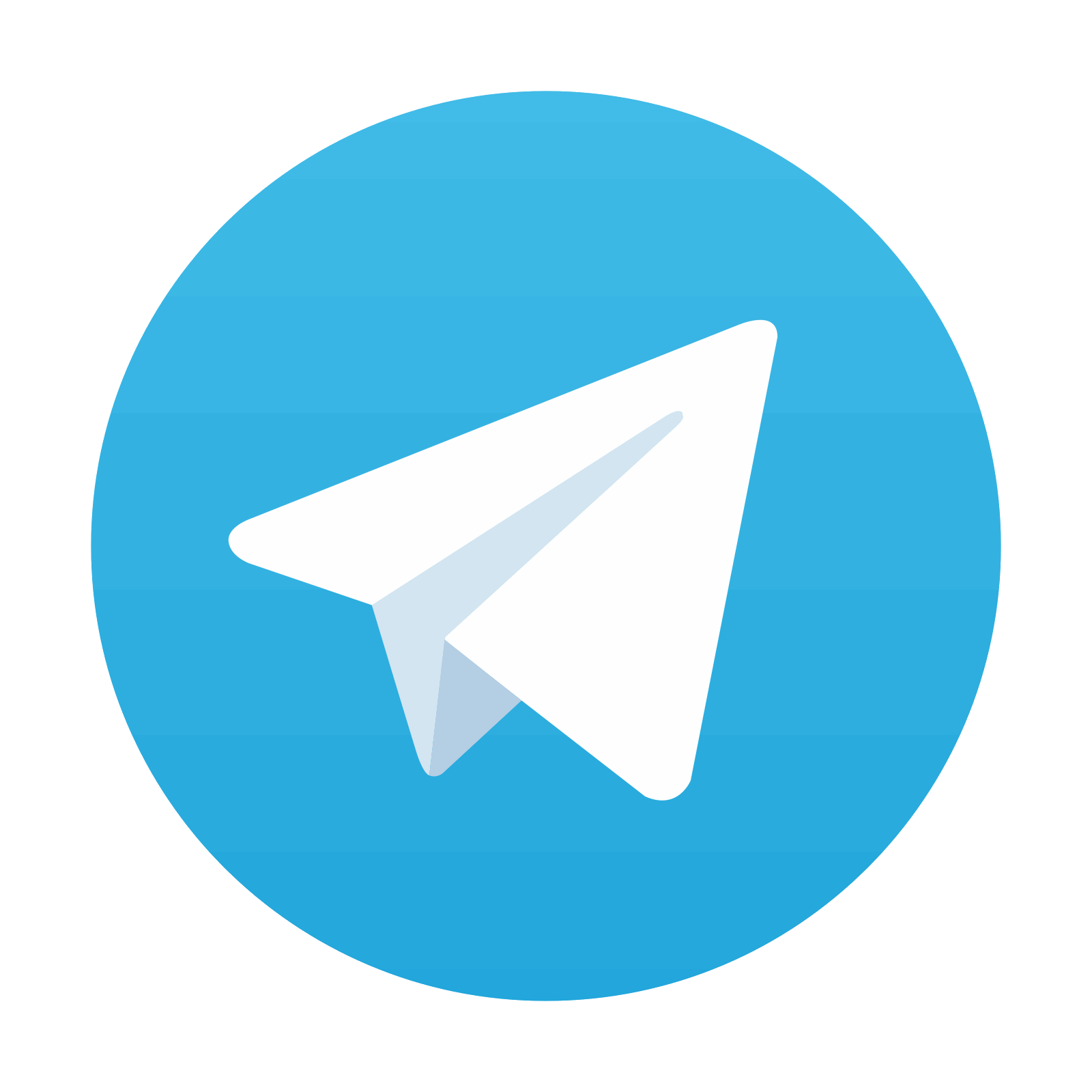
Stay updated, free articles. Join our Telegram channel

Full access? Get Clinical Tree
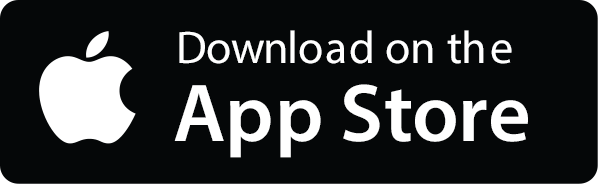
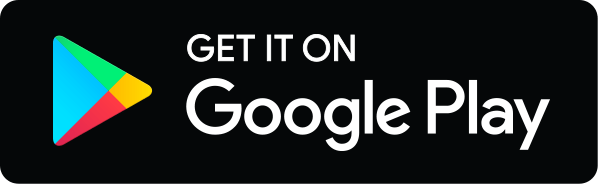