Chapter 65 Performance Deficits during Sleep Loss
Effects of Time Awake, Time of Day, and Time on Task
Abstract
Awareness of the deleterious effects of sleep loss have grown steadily over the past decade. Awareness has been bolstered by public educational campaigns by the National Sleep Foundation, the National Sleep Disorders Center at the National Institutes of Health (NIH), and other like-minded organizations; by publication of the Institute of Medicine’s report Sleep Disorders and Sleep Deprivation: An Unmet Public Health Problem (2006)1; and by findings from epidemiologic studies linking reduced average daily sleep duration with potential health consequences including obesity, diabetes,2 and reduced longevity.3
However, the most widely appreciated and most extensively researched effects of sleep loss are its acute and profound effects on alertness and performance, with performance defined generally as goal-directed behavior requiring mental effort. Although difficult to estimate, it is likely that sleepiness-induced performance deficits cost the world economy hundreds of billions of dollars per year in accidents, direct health care costs, and lost operational efficiency and productivity. This can be inferred from the fact that significant sleepiness is experienced at least occasionally by everyone and may be experienced chronically by a considerable portion of the adult population. According to the 2004-2006 National Health Interview Survey, approximately 21% of adults in the United States typically obtain 6 or fewer hours of sleep per 24 hours4—considerably less than the 7 to 8 hours of sleep that is recommended.5 And if those with sleep disorders (e.g., sleep apnea, insomnia) are considered along with those who are chronically sleep restricted for other reasons (e.g., shift work), it is estimated that as many as 70 million Americans experience chronic sleep loss5 and therefore may experience correspondingly increased sleepiness and impaired performance on a daily basis.
The Nature of Sleepiness-Induced Performance Deficits
Cognitive performance is not solely a function of sleepiness, and it is therefore not simply a direct reflection of level of sleepiness. But level of sleepiness—as determined by duration of prior sleep, time since awakening, and phase of the circadian rhythm of alertness—does affect performance on a variety of tasks in a predictable manner. Thus, for example, average reaction time performance on the psychomotor vigilance test (PVT) increases in a dose-dependent fashion with decreasing amounts of nighttime sleep and increasing levels of sleepiness across multiple days of sleep restriction.6,7 Sleepiness-induced declines in average performance are not, however, the result of a wholesale shift in the entire distribution of reaction times, but rather reflect increased variability in the distribution of reaction times—the result of an increasing proportion of relatively long reaction times intermixed with “normal” reaction times (i.e., reaction times within the range typical for normal alertness).
Based largely on this observation, it has been suggested that the increased response variability that characterizes sleep loss is most likely a manifestation of a reduced level of stability in the physiologic processes by which wakefulness is maintained—specifically, that it is caused by intermittent intrusion of sleep-onset mechanisms into wakefulness.8,9 It has been proposed that the physiologic basis of this state instability may be a toggle switch (of the sort proposed by Saper and colleagues10), in which sleep-promoting neurons within the ventral lateral preoptic nucleus and monoaminergic wake-promoting neurons inhibit each other, resulting in stable sleep when the former neurons are more active, stable wakefulness when the latter are more active, and relative instability as the activity levels and influence of these two cell groups on the toggle switch approaches parity.11
Functional Brain Imaging Studies of Sleepiness and Performance
Functional brain imaging techniques have shown that sleep loss results in reduced brain activation, with greatest reductions manifest in prefrontal cortex, inferior parietal and superior temporal cortex, and thalamus.12 Based on such findings, it has proved possible to predict specific sleep loss–induced deficits in various aspects of performance. For example, noting that sleep deprivation results in reduced metabolic activity in specific regions of the prefrontal cortex known to mediate specific aspects of cognitive performance and perception, it has been predicted and confirmed that sleep deprivation causes deficits in executive mental functions such as decision making,13 moral judgment,14 and humor appreciation15 and that it results in reduced ability to differentiate odors.16
Although such studies clearly suggest a relationship between the absolute level of activity in specific brain regions and cognitive performance, the relationship between regional brain activation and performance is not always straightforward. In an elegant series of studies, Drummond and coworkers17–19 demonstrated not only that sleepiness-related performance deficits are associated with region-specific deficits in activation but also that the ability to maintain baseline-level performance on specific tasks during sleep deprivation was associated with relative activation of cortical regions that were not significantly activated during performance of the same task in the well-rested state. Subjects whose functional magnetic resonance imaging (fMRI) images revealed such new regional relative activations during sleep deprivation were better able to maintain task performance than were those whose fMRI images failed to reveal similar new activations. This suggests that individual differences in ability to recruit resources from other brain regions might underlie task-specific individual differences in resilience during sleep loss.
The relationship between sleepiness and performance is perhaps best illustrated during the first several minutes after awakening, when sleep inertia effects are manifest. The term sleep inertia refers to the immediate postawakening period that is characterized by profound sleepiness and performance deficits, which rapidly dissipate over 20 minutes or more of continuous wakefulness.20 Sleep inertia constitutes a unique and somewhat paradoxical state because it is a period of profound sleepiness and performance deficits without a correspondingly profound sleep debt. That is, actual sleep debt is at a local minimum immediately upon awakening (by virtue of the recuperation obtained during the preceding sleep period) but it takes 20 minutes or more of continuous wakefulness before the normal correspondence between sleep debt and alertness level is reestablished. Therefore, functional brain imaging studies during the immediate postawakening period characterize a sleep-sated (but sleepy) brain that is ascending toward alertness, rather than a sleep-deficient, sleepy brain struggling to remain awake (as during sleep-deprivation studies).21
A comparison of the regional cerebral blood flow pattern during the sleep inertia period versus previous studies of sleep deprivation and non–rapid eye movement (NREM) sleep reveal that sleep-deprived wakefulness is characterized by global reductions in brain activity (relative to normal, well-rested wakefulness), with the greatest reductions evident in heteromodal (primarily prefrontal) association cortices and thalamus.12 A similar pattern is evident when comparing slow-wave sleep to normal wakefulness: global deactivation (albeit of a greater magnitude than during sleep-deprived wakefulness), with the greatest deactivations evident in anterior (prefrontal) cortical regions and centrencephalic regions, including the thalamus.22 In contrast, the immediate postawakening (sleep inertia) period is characterized by bidirectional changes in regional cerebral blood flow, with waxing activity in anterior cortical regions and waning activity in centrencephalic regions across the first 5 to 20 minutes of wakefulness (Fig. 65-1).23 Because deactivated prefrontal cortex is the only common finding during these three states (sleep-deprived wakefulness, sleep, and sleep inertia), it can be surmised that sleepiness and its attendant performance deficits are ultimately and specifically a function of activity in the prefrontal cortices.
Changes in the pattern of interregional functional connectivity across the first 20 minutes of wakefulness (i.e., during the time period when sleepiness and performance deficits are known to dissipate most rapidly) confirm a primary role of the prefrontal cortices, revealing that some of the most robust changes in functional connectivity patterns across the first 20 minutes of wakefulness center on this region: A negative correlation between activity in the prefrontal cortex and reticular formation at 5 minutes after awakening dissipates by 20 minutes after awakening. Conversely, activity in the caudate and prefrontal cortex is not significantly correlated at 5 minutes after awakening, but it is positively and robustly correlated at 20 minutes after awakening.23 In contrast, a positive, relatively stable correlation of activity in the anterior cingulate cortex and prefrontal cortex across the 20 minute postawakening period suggests that this functional relationship probably does not mediate alertness and performance but might instead be part of the pattern of functional connectivity that underlies conscious wakefulness itself, which is also, of course, a basic requirement for goal-directed performance.
Influence of the Circadian Rhythm of Alertness on Performance
The circadian rhythm of alertness has been well described (for review, see reference 24 and see Section 5). Briefly, output from the suprachiasmatic nucleus (SCN) of the hypothalamus drives a variety of body functions on an approximately 24-hour rhythm. Core body temperature follows a 24-hour rhythm, rising throughout the day to peak in the evening and subsequently declining to a trough in the morning; cognitive performance parallels fluctuations in temperature.25 In the absence of sleep, subjective26 and objective27 sleepiness (as well as performance) continue to track this rhythm.
The three-process model of alertness28 provides a convenient framework for conceptualizing the main factors that interactively mediate sleepiness and performance. In this model, which is an extension of the two-process model,29 Process S is an exponential function representing the sleep homeostat, a reflection of the underlying physiologically based need for sleep.30 Process S thus increases across the waking period, reflecting an increasing physiologic need for sleep.
Process W reflects the temporary performance deficits and sleepiness that characterize the first approximately 20 minutes of wakefulness.20 Because sleep need immediately upon awakening must be at a local minimum (i.e., Process S must be at a local minimum) it is likely that Process W reflects the residual (and waning during wakefulness) influence of sleep maintenance mechanisms.21
Process C is the circadian factor, a sinusoidal function with a peak (acrophase) in the evening hours and a nadir in the early morning hours. From an evolutionary standpoint, Process C can be thought of as an adaptively useful physiologic opponent to Process S, consolidating wakefulness during daytime hours and sleep during nighttime hours in diurnal animals such as humans.31
Functionally, sleepiness and its attendant performance deficits can be conceptualized as the product of the combined effects of Processes S, C, and (during the immediate postawakening period) W. The desynchrony between circadian phase and work–sleep schedule associated with shift work can result in reduced performance and alertness during the night shift and cumulative sleep loss resulting from inadequate daytime sleep. Regardless of total amount of sleep lost, human performance declines in the early morning hours when the circadian rhythm of alertness is approaching or at its nadir; in a complementary manner, daytime sleep is impaired during the ascending phase of the circadian rhythm of alertness, and sleep at this time of the day is typically shorter, more fragmented, and therefore less recuperative. Under normal (nonlaboratory) conditions, the circadian rhythm of alertness is primarily influenced by the timing of daily light exposure.32
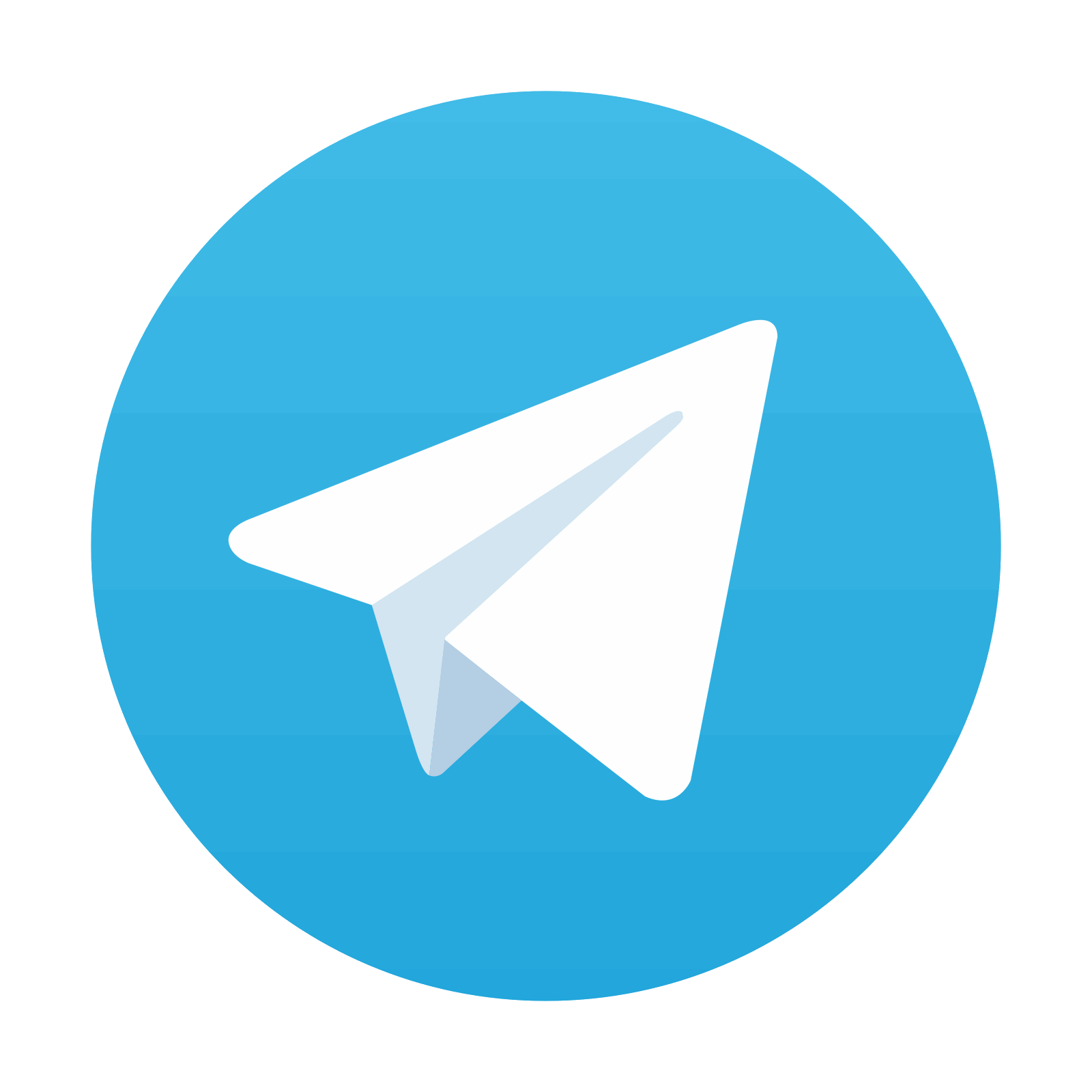
Stay updated, free articles. Join our Telegram channel

Full access? Get Clinical Tree
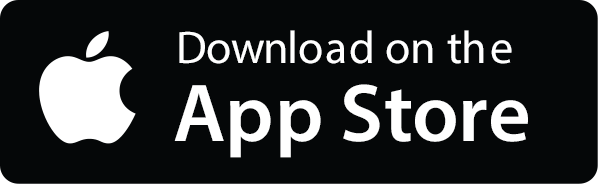
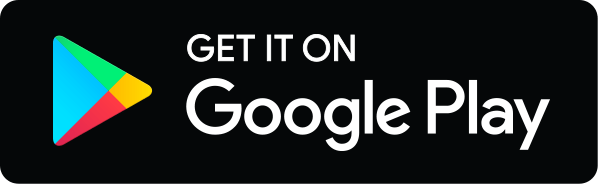